- Research article
- Open access
- Published: 04 June 2021
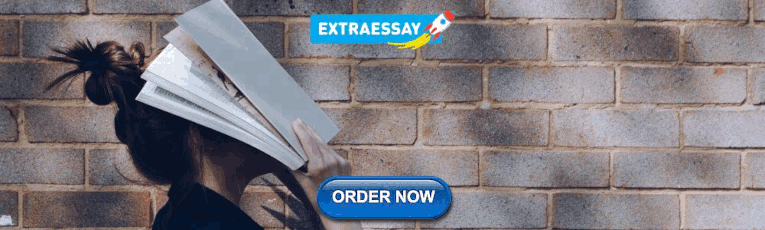
Coronavirus disease (COVID-19) pandemic: an overview of systematic reviews
- Israel Júnior Borges do Nascimento 1 , 2 ,
- Dónal P. O’Mathúna 3 , 4 ,
- Thilo Caspar von Groote 5 ,
- Hebatullah Mohamed Abdulazeem 6 ,
- Ishanka Weerasekara 7 , 8 ,
- Ana Marusic 9 ,
- Livia Puljak ORCID: orcid.org/0000-0002-8467-6061 10 ,
- Vinicius Tassoni Civile 11 ,
- Irena Zakarija-Grkovic 9 ,
- Tina Poklepovic Pericic 9 ,
- Alvaro Nagib Atallah 11 ,
- Santino Filoso 12 ,
- Nicola Luigi Bragazzi 13 &
- Milena Soriano Marcolino 1
On behalf of the International Network of Coronavirus Disease 2019 (InterNetCOVID-19)
BMC Infectious Diseases volume 21 , Article number: 525 ( 2021 ) Cite this article
16k Accesses
29 Citations
13 Altmetric
Metrics details
Navigating the rapidly growing body of scientific literature on the SARS-CoV-2 pandemic is challenging, and ongoing critical appraisal of this output is essential. We aimed to summarize and critically appraise systematic reviews of coronavirus disease (COVID-19) in humans that were available at the beginning of the pandemic.
Nine databases (Medline, EMBASE, Cochrane Library, CINAHL, Web of Sciences, PDQ-Evidence, WHO’s Global Research, LILACS, and Epistemonikos) were searched from December 1, 2019, to March 24, 2020. Systematic reviews analyzing primary studies of COVID-19 were included. Two authors independently undertook screening, selection, extraction (data on clinical symptoms, prevalence, pharmacological and non-pharmacological interventions, diagnostic test assessment, laboratory, and radiological findings), and quality assessment (AMSTAR 2). A meta-analysis was performed of the prevalence of clinical outcomes.
Eighteen systematic reviews were included; one was empty (did not identify any relevant study). Using AMSTAR 2, confidence in the results of all 18 reviews was rated as “critically low”. Identified symptoms of COVID-19 were (range values of point estimates): fever (82–95%), cough with or without sputum (58–72%), dyspnea (26–59%), myalgia or muscle fatigue (29–51%), sore throat (10–13%), headache (8–12%) and gastrointestinal complaints (5–9%). Severe symptoms were more common in men. Elevated C-reactive protein and lactate dehydrogenase, and slightly elevated aspartate and alanine aminotransferase, were commonly described. Thrombocytopenia and elevated levels of procalcitonin and cardiac troponin I were associated with severe disease. A frequent finding on chest imaging was uni- or bilateral multilobar ground-glass opacity. A single review investigated the impact of medication (chloroquine) but found no verifiable clinical data. All-cause mortality ranged from 0.3 to 13.9%.
Conclusions
In this overview of systematic reviews, we analyzed evidence from the first 18 systematic reviews that were published after the emergence of COVID-19. However, confidence in the results of all reviews was “critically low”. Thus, systematic reviews that were published early on in the pandemic were of questionable usefulness. Even during public health emergencies, studies and systematic reviews should adhere to established methodological standards.
Peer Review reports
The spread of the “Severe Acute Respiratory Coronavirus 2” (SARS-CoV-2), the causal agent of COVID-19, was characterized as a pandemic by the World Health Organization (WHO) in March 2020 and has triggered an international public health emergency [ 1 ]. The numbers of confirmed cases and deaths due to COVID-19 are rapidly escalating, counting in millions [ 2 ], causing massive economic strain, and escalating healthcare and public health expenses [ 3 , 4 ].
The research community has responded by publishing an impressive number of scientific reports related to COVID-19. The world was alerted to the new disease at the beginning of 2020 [ 1 ], and by mid-March 2020, more than 2000 articles had been published on COVID-19 in scholarly journals, with 25% of them containing original data [ 5 ]. The living map of COVID-19 evidence, curated by the Evidence for Policy and Practice Information and Co-ordinating Centre (EPPI-Centre), contained more than 40,000 records by February 2021 [ 6 ]. More than 100,000 records on PubMed were labeled as “SARS-CoV-2 literature, sequence, and clinical content” by February 2021 [ 7 ].
Due to publication speed, the research community has voiced concerns regarding the quality and reproducibility of evidence produced during the COVID-19 pandemic, warning of the potential damaging approach of “publish first, retract later” [ 8 ]. It appears that these concerns are not unfounded, as it has been reported that COVID-19 articles were overrepresented in the pool of retracted articles in 2020 [ 9 ]. These concerns about inadequate evidence are of major importance because they can lead to poor clinical practice and inappropriate policies [ 10 ].
Systematic reviews are a cornerstone of today’s evidence-informed decision-making. By synthesizing all relevant evidence regarding a particular topic, systematic reviews reflect the current scientific knowledge. Systematic reviews are considered to be at the highest level in the hierarchy of evidence and should be used to make informed decisions. However, with high numbers of systematic reviews of different scope and methodological quality being published, overviews of multiple systematic reviews that assess their methodological quality are essential [ 11 , 12 , 13 ]. An overview of systematic reviews helps identify and organize the literature and highlights areas of priority in decision-making.
In this overview of systematic reviews, we aimed to summarize and critically appraise systematic reviews of coronavirus disease (COVID-19) in humans that were available at the beginning of the pandemic.
Methodology
Research question.
This overview’s primary objective was to summarize and critically appraise systematic reviews that assessed any type of primary clinical data from patients infected with SARS-CoV-2. Our research question was purposefully broad because we wanted to analyze as many systematic reviews as possible that were available early following the COVID-19 outbreak.
Study design
We conducted an overview of systematic reviews. The idea for this overview originated in a protocol for a systematic review submitted to PROSPERO (CRD42020170623), which indicated a plan to conduct an overview.
Overviews of systematic reviews use explicit and systematic methods for searching and identifying multiple systematic reviews addressing related research questions in the same field to extract and analyze evidence across important outcomes. Overviews of systematic reviews are in principle similar to systematic reviews of interventions, but the unit of analysis is a systematic review [ 14 , 15 , 16 ].
We used the overview methodology instead of other evidence synthesis methods to allow us to collate and appraise multiple systematic reviews on this topic, and to extract and analyze their results across relevant topics [ 17 ]. The overview and meta-analysis of systematic reviews allowed us to investigate the methodological quality of included studies, summarize results, and identify specific areas of available or limited evidence, thereby strengthening the current understanding of this novel disease and guiding future research [ 13 ].
A reporting guideline for overviews of reviews is currently under development, i.e., Preferred Reporting Items for Overviews of Reviews (PRIOR) [ 18 ]. As the PRIOR checklist is still not published, this study was reported following the Preferred Reporting Items for Systematic Reviews and Meta-Analyses (PRISMA) 2009 statement [ 19 ]. The methodology used in this review was adapted from the Cochrane Handbook for Systematic Reviews of Interventions and also followed established methodological considerations for analyzing existing systematic reviews [ 14 ].
Approval of a research ethics committee was not necessary as the study analyzed only publicly available articles.
Eligibility criteria
Systematic reviews were included if they analyzed primary data from patients infected with SARS-CoV-2 as confirmed by RT-PCR or another pre-specified diagnostic technique. Eligible reviews covered all topics related to COVID-19 including, but not limited to, those that reported clinical symptoms, diagnostic methods, therapeutic interventions, laboratory findings, or radiological results. Both full manuscripts and abbreviated versions, such as letters, were eligible.
No restrictions were imposed on the design of the primary studies included within the systematic reviews, the last search date, whether the review included meta-analyses or language. Reviews related to SARS-CoV-2 and other coronaviruses were eligible, but from those reviews, we analyzed only data related to SARS-CoV-2.
No consensus definition exists for a systematic review [ 20 ], and debates continue about the defining characteristics of a systematic review [ 21 ]. Cochrane’s guidance for overviews of reviews recommends setting pre-established criteria for making decisions around inclusion [ 14 ]. That is supported by a recent scoping review about guidance for overviews of systematic reviews [ 22 ].
Thus, for this study, we defined a systematic review as a research report which searched for primary research studies on a specific topic using an explicit search strategy, had a detailed description of the methods with explicit inclusion criteria provided, and provided a summary of the included studies either in narrative or quantitative format (such as a meta-analysis). Cochrane and non-Cochrane systematic reviews were considered eligible for inclusion, with or without meta-analysis, and regardless of the study design, language restriction and methodology of the included primary studies. To be eligible for inclusion, reviews had to be clearly analyzing data related to SARS-CoV-2 (associated or not with other viruses). We excluded narrative reviews without those characteristics as these are less likely to be replicable and are more prone to bias.
Scoping reviews and rapid reviews were eligible for inclusion in this overview if they met our pre-defined inclusion criteria noted above. We included reviews that addressed SARS-CoV-2 and other coronaviruses if they reported separate data regarding SARS-CoV-2.
Information sources
Nine databases were searched for eligible records published between December 1, 2019, and March 24, 2020: Cochrane Database of Systematic Reviews via Cochrane Library, PubMed, EMBASE, CINAHL (Cumulative Index to Nursing and Allied Health Literature), Web of Sciences, LILACS (Latin American and Caribbean Health Sciences Literature), PDQ-Evidence, WHO’s Global Research on Coronavirus Disease (COVID-19), and Epistemonikos.
The comprehensive search strategy for each database is provided in Additional file 1 and was designed and conducted in collaboration with an information specialist. All retrieved records were primarily processed in EndNote, where duplicates were removed, and records were then imported into the Covidence platform [ 23 ]. In addition to database searches, we screened reference lists of reviews included after screening records retrieved via databases.
Study selection
All searches, screening of titles and abstracts, and record selection, were performed independently by two investigators using the Covidence platform [ 23 ]. Articles deemed potentially eligible were retrieved for full-text screening carried out independently by two investigators. Discrepancies at all stages were resolved by consensus. During the screening, records published in languages other than English were translated by a native/fluent speaker.
Data collection process
We custom designed a data extraction table for this study, which was piloted by two authors independently. Data extraction was performed independently by two authors. Conflicts were resolved by consensus or by consulting a third researcher.
We extracted the following data: article identification data (authors’ name and journal of publication), search period, number of databases searched, population or settings considered, main results and outcomes observed, and number of participants. From Web of Science (Clarivate Analytics, Philadelphia, PA, USA), we extracted journal rank (quartile) and Journal Impact Factor (JIF).
We categorized the following as primary outcomes: all-cause mortality, need for and length of mechanical ventilation, length of hospitalization (in days), admission to intensive care unit (yes/no), and length of stay in the intensive care unit.
The following outcomes were categorized as exploratory: diagnostic methods used for detection of the virus, male to female ratio, clinical symptoms, pharmacological and non-pharmacological interventions, laboratory findings (full blood count, liver enzymes, C-reactive protein, d-dimer, albumin, lipid profile, serum electrolytes, blood vitamin levels, glucose levels, and any other important biomarkers), and radiological findings (using radiography, computed tomography, magnetic resonance imaging or ultrasound).
We also collected data on reporting guidelines and requirements for the publication of systematic reviews and meta-analyses from journal websites where included reviews were published.
Quality assessment in individual reviews
Two researchers independently assessed the reviews’ quality using the “A MeaSurement Tool to Assess Systematic Reviews 2 (AMSTAR 2)”. We acknowledge that the AMSTAR 2 was created as “a critical appraisal tool for systematic reviews that include randomized or non-randomized studies of healthcare interventions, or both” [ 24 ]. However, since AMSTAR 2 was designed for systematic reviews of intervention trials, and we included additional types of systematic reviews, we adjusted some AMSTAR 2 ratings and reported these in Additional file 2 .
Adherence to each item was rated as follows: yes, partial yes, no, or not applicable (such as when a meta-analysis was not conducted). The overall confidence in the results of the review is rated as “critically low”, “low”, “moderate” or “high”, according to the AMSTAR 2 guidance based on seven critical domains, which are items 2, 4, 7, 9, 11, 13, 15 as defined by AMSTAR 2 authors [ 24 ]. We reported our adherence ratings for transparency of our decision with accompanying explanations, for each item, in each included review.
One of the included systematic reviews was conducted by some members of this author team [ 25 ]. This review was initially assessed independently by two authors who were not co-authors of that review to prevent the risk of bias in assessing this study.
Synthesis of results
For data synthesis, we prepared a table summarizing each systematic review. Graphs illustrating the mortality rate and clinical symptoms were created. We then prepared a narrative summary of the methods, findings, study strengths, and limitations.
For analysis of the prevalence of clinical outcomes, we extracted data on the number of events and the total number of patients to perform proportional meta-analysis using RStudio© software, with the “meta” package (version 4.9–6), using the “metaprop” function for reviews that did not perform a meta-analysis, excluding case studies because of the absence of variance. For reviews that did not perform a meta-analysis, we presented pooled results of proportions with their respective confidence intervals (95%) by the inverse variance method with a random-effects model, using the DerSimonian-Laird estimator for τ 2 . We adjusted data using Freeman-Tukey double arcosen transformation. Confidence intervals were calculated using the Clopper-Pearson method for individual studies. We created forest plots using the RStudio© software, with the “metafor” package (version 2.1–0) and “forest” function.
Managing overlapping systematic reviews
Some of the included systematic reviews that address the same or similar research questions may include the same primary studies in overviews. Including such overlapping reviews may introduce bias when outcome data from the same primary study are included in the analyses of an overview multiple times. Thus, in summaries of evidence, multiple-counting of the same outcome data will give data from some primary studies too much influence [ 14 ]. In this overview, we did not exclude overlapping systematic reviews because, according to Cochrane’s guidance, it may be appropriate to include all relevant reviews’ results if the purpose of the overview is to present and describe the current body of evidence on a topic [ 14 ]. To avoid any bias in summary estimates associated with overlapping reviews, we generated forest plots showing data from individual systematic reviews, but the results were not pooled because some primary studies were included in multiple reviews.
Our search retrieved 1063 publications, of which 175 were duplicates. Most publications were excluded after the title and abstract analysis ( n = 860). Among the 28 studies selected for full-text screening, 10 were excluded for the reasons described in Additional file 3 , and 18 were included in the final analysis (Fig. 1 ) [ 25 , 26 , 27 , 28 , 29 , 30 , 31 , 32 , 33 , 34 , 35 , 36 , 37 , 38 , 39 , 40 , 41 , 42 ]. Reference list screening did not retrieve any additional systematic reviews.
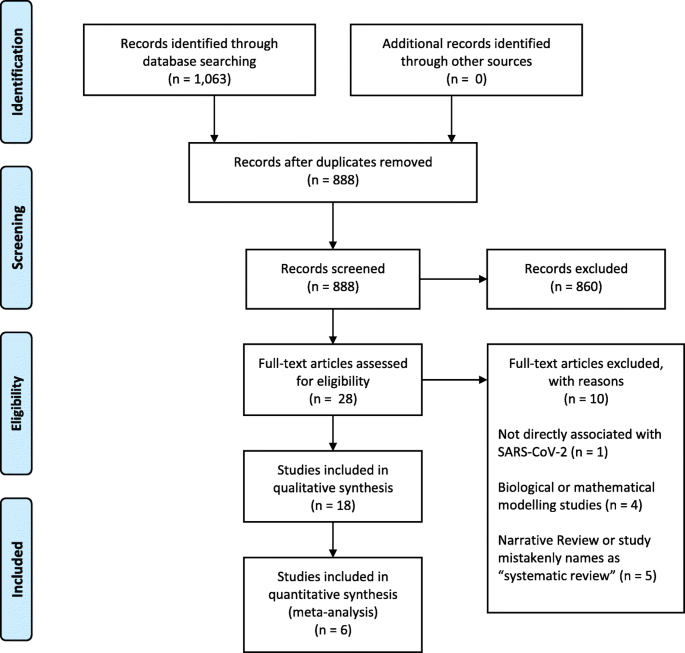
PRISMA flow diagram
Characteristics of included reviews
Summary features of 18 systematic reviews are presented in Table 1 . They were published in 14 different journals. Only four of these journals had specific requirements for systematic reviews (with or without meta-analysis): European Journal of Internal Medicine, Journal of Clinical Medicine, Ultrasound in Obstetrics and Gynecology, and Clinical Research in Cardiology . Two journals reported that they published only invited reviews ( Journal of Medical Virology and Clinica Chimica Acta ). Three systematic reviews in our study were published as letters; one was labeled as a scoping review and another as a rapid review (Table 2 ).
All reviews were published in English, in first quartile (Q1) journals, with JIF ranging from 1.692 to 6.062. One review was empty, meaning that its search did not identify any relevant studies; i.e., no primary studies were included [ 36 ]. The remaining 17 reviews included 269 unique studies; the majority ( N = 211; 78%) were included in only a single review included in our study (range: 1 to 12). Primary studies included in the reviews were published between December 2019 and March 18, 2020, and comprised case reports, case series, cohorts, and other observational studies. We found only one review that included randomized clinical trials [ 38 ]. In the included reviews, systematic literature searches were performed from 2019 (entire year) up to March 9, 2020. Ten systematic reviews included meta-analyses. The list of primary studies found in the included systematic reviews is shown in Additional file 4 , as well as the number of reviews in which each primary study was included.
Population and study designs
Most of the reviews analyzed data from patients with COVID-19 who developed pneumonia, acute respiratory distress syndrome (ARDS), or any other correlated complication. One review aimed to evaluate the effectiveness of using surgical masks on preventing transmission of the virus [ 36 ], one review was focused on pediatric patients [ 34 ], and one review investigated COVID-19 in pregnant women [ 37 ]. Most reviews assessed clinical symptoms, laboratory findings, or radiological results.
Systematic review findings
The summary of findings from individual reviews is shown in Table 2 . Overall, all-cause mortality ranged from 0.3 to 13.9% (Fig. 2 ).
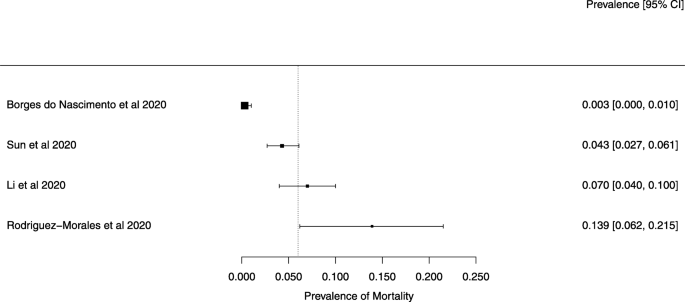
A meta-analysis of the prevalence of mortality
Clinical symptoms
Seven reviews described the main clinical manifestations of COVID-19 [ 26 , 28 , 29 , 34 , 35 , 39 , 41 ]. Three of them provided only a narrative discussion of symptoms [ 26 , 34 , 35 ]. In the reviews that performed a statistical analysis of the incidence of different clinical symptoms, symptoms in patients with COVID-19 were (range values of point estimates): fever (82–95%), cough with or without sputum (58–72%), dyspnea (26–59%), myalgia or muscle fatigue (29–51%), sore throat (10–13%), headache (8–12%), gastrointestinal disorders, such as diarrhea, nausea or vomiting (5.0–9.0%), and others (including, in one study only: dizziness 12.1%) (Figs. 3 , 4 , 5 , 6 , 7 , 8 and 9 ). Three reviews assessed cough with and without sputum together; only one review assessed sputum production itself (28.5%).
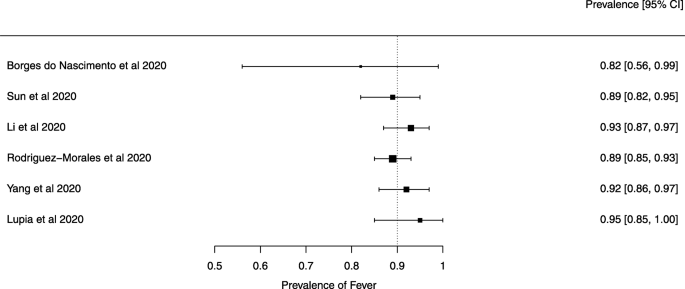
A meta-analysis of the prevalence of fever
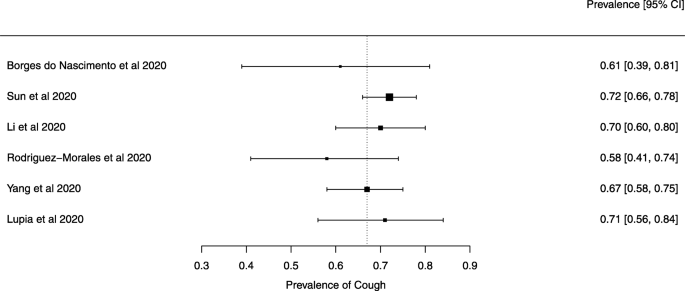
A meta-analysis of the prevalence of cough
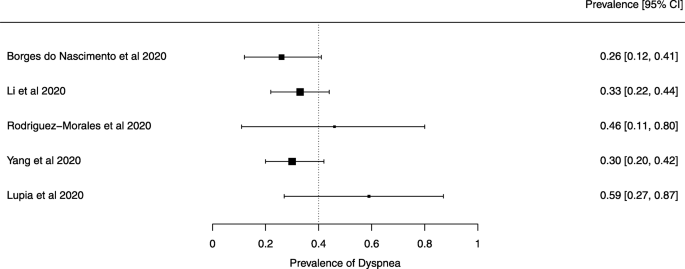
A meta-analysis of the prevalence of dyspnea
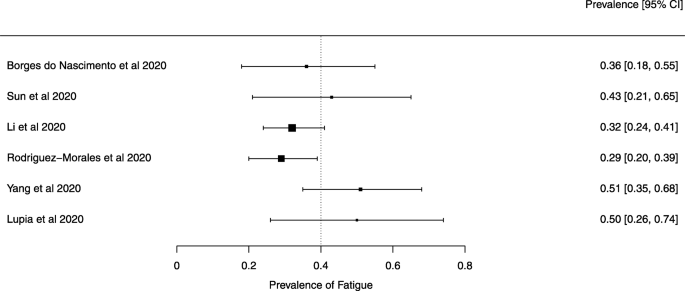
A meta-analysis of the prevalence of fatigue or myalgia
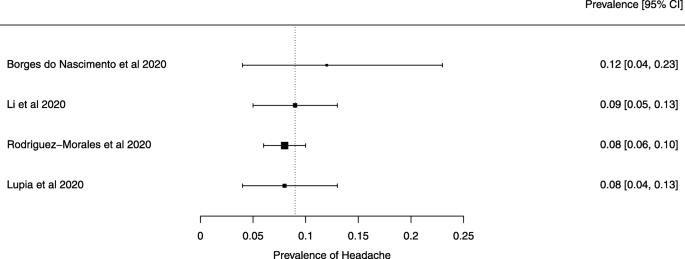
A meta-analysis of the prevalence of headache
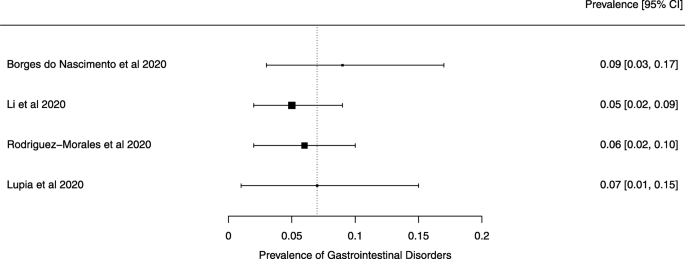
A meta-analysis of the prevalence of gastrointestinal disorders
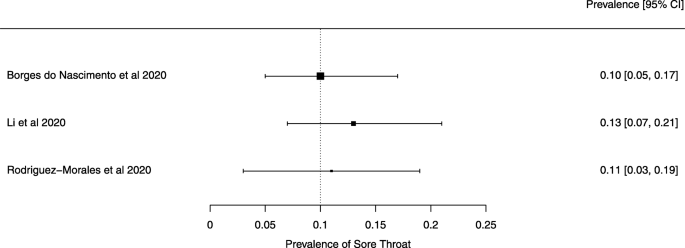
A meta-analysis of the prevalence of sore throat
Diagnostic aspects
Three reviews described methodologies, protocols, and tools used for establishing the diagnosis of COVID-19 [ 26 , 34 , 38 ]. The use of respiratory swabs (nasal or pharyngeal) or blood specimens to assess the presence of SARS-CoV-2 nucleic acid using RT-PCR assays was the most commonly used diagnostic method mentioned in the included studies. These diagnostic tests have been widely used, but their precise sensitivity and specificity remain unknown. One review included a Chinese study with clinical diagnosis with no confirmation of SARS-CoV-2 infection (patients were diagnosed with COVID-19 if they presented with at least two symptoms suggestive of COVID-19, together with laboratory and chest radiography abnormalities) [ 34 ].
Therapeutic possibilities
Pharmacological and non-pharmacological interventions (supportive therapies) used in treating patients with COVID-19 were reported in five reviews [ 25 , 27 , 34 , 35 , 38 ]. Antivirals used empirically for COVID-19 treatment were reported in seven reviews [ 25 , 27 , 34 , 35 , 37 , 38 , 41 ]; most commonly used were protease inhibitors (lopinavir, ritonavir, darunavir), nucleoside reverse transcriptase inhibitor (tenofovir), nucleotide analogs (remdesivir, galidesivir, ganciclovir), and neuraminidase inhibitors (oseltamivir). Umifenovir, a membrane fusion inhibitor, was investigated in two studies [ 25 , 35 ]. Possible supportive interventions analyzed were different types of oxygen supplementation and breathing support (invasive or non-invasive ventilation) [ 25 ]. The use of antibiotics, both empirically and to treat secondary pneumonia, was reported in six studies [ 25 , 26 , 27 , 34 , 35 , 38 ]. One review specifically assessed evidence on the efficacy and safety of the anti-malaria drug chloroquine [ 27 ]. It identified 23 ongoing trials investigating the potential of chloroquine as a therapeutic option for COVID-19, but no verifiable clinical outcomes data. The use of mesenchymal stem cells, antifungals, and glucocorticoids were described in four reviews [ 25 , 34 , 35 , 38 ].
Laboratory and radiological findings
Of the 18 reviews included in this overview, eight analyzed laboratory parameters in patients with COVID-19 [ 25 , 29 , 30 , 32 , 33 , 34 , 35 , 39 ]; elevated C-reactive protein levels, associated with lymphocytopenia, elevated lactate dehydrogenase, as well as slightly elevated aspartate and alanine aminotransferase (AST, ALT) were commonly described in those eight reviews. Lippi et al. assessed cardiac troponin I (cTnI) [ 25 ], procalcitonin [ 32 ], and platelet count [ 33 ] in COVID-19 patients. Elevated levels of procalcitonin [ 32 ] and cTnI [ 30 ] were more likely to be associated with a severe disease course (requiring intensive care unit admission and intubation). Furthermore, thrombocytopenia was frequently observed in patients with complicated COVID-19 infections [ 33 ].
Chest imaging (chest radiography and/or computed tomography) features were assessed in six reviews, all of which described a frequent pattern of local or bilateral multilobar ground-glass opacity [ 25 , 34 , 35 , 39 , 40 , 41 ]. Those six reviews showed that septal thickening, bronchiectasis, pleural and cardiac effusions, halo signs, and pneumothorax were observed in patients suffering from COVID-19.
Quality of evidence in individual systematic reviews
Table 3 shows the detailed results of the quality assessment of 18 systematic reviews, including the assessment of individual items and summary assessment. A detailed explanation for each decision in each review is available in Additional file 5 .
Using AMSTAR 2 criteria, confidence in the results of all 18 reviews was rated as “critically low” (Table 3 ). Common methodological drawbacks were: omission of prospective protocol submission or publication; use of inappropriate search strategy: lack of independent and dual literature screening and data-extraction (or methodology unclear); absence of an explanation for heterogeneity among the studies included; lack of reasons for study exclusion (or rationale unclear).
Risk of bias assessment, based on a reported methodological tool, and quality of evidence appraisal, in line with the Grading of Recommendations Assessment, Development, and Evaluation (GRADE) method, were reported only in one review [ 25 ]. Five reviews presented a table summarizing bias, using various risk of bias tools [ 25 , 29 , 39 , 40 , 41 ]. One review analyzed “study quality” [ 37 ]. One review mentioned the risk of bias assessment in the methodology but did not provide any related analysis [ 28 ].
This overview of systematic reviews analyzed the first 18 systematic reviews published after the onset of the COVID-19 pandemic, up to March 24, 2020, with primary studies involving more than 60,000 patients. Using AMSTAR-2, we judged that our confidence in all those reviews was “critically low”. Ten reviews included meta-analyses. The reviews presented data on clinical manifestations, laboratory and radiological findings, and interventions. We found no systematic reviews on the utility of diagnostic tests.
Symptoms were reported in seven reviews; most of the patients had a fever, cough, dyspnea, myalgia or muscle fatigue, and gastrointestinal disorders such as diarrhea, nausea, or vomiting. Olfactory dysfunction (anosmia or dysosmia) has been described in patients infected with COVID-19 [ 43 ]; however, this was not reported in any of the reviews included in this overview. During the SARS outbreak in 2002, there were reports of impairment of the sense of smell associated with the disease [ 44 , 45 ].
The reported mortality rates ranged from 0.3 to 14% in the included reviews. Mortality estimates are influenced by the transmissibility rate (basic reproduction number), availability of diagnostic tools, notification policies, asymptomatic presentations of the disease, resources for disease prevention and control, and treatment facilities; variability in the mortality rate fits the pattern of emerging infectious diseases [ 46 ]. Furthermore, the reported cases did not consider asymptomatic cases, mild cases where individuals have not sought medical treatment, and the fact that many countries had limited access to diagnostic tests or have implemented testing policies later than the others. Considering the lack of reviews assessing diagnostic testing (sensitivity, specificity, and predictive values of RT-PCT or immunoglobulin tests), and the preponderance of studies that assessed only symptomatic individuals, considerable imprecision around the calculated mortality rates existed in the early stage of the COVID-19 pandemic.
Few reviews included treatment data. Those reviews described studies considered to be at a very low level of evidence: usually small, retrospective studies with very heterogeneous populations. Seven reviews analyzed laboratory parameters; those reviews could have been useful for clinicians who attend patients suspected of COVID-19 in emergency services worldwide, such as assessing which patients need to be reassessed more frequently.
All systematic reviews scored poorly on the AMSTAR 2 critical appraisal tool for systematic reviews. Most of the original studies included in the reviews were case series and case reports, impacting the quality of evidence. Such evidence has major implications for clinical practice and the use of these reviews in evidence-based practice and policy. Clinicians, patients, and policymakers can only have the highest confidence in systematic review findings if high-quality systematic review methodologies are employed. The urgent need for information during a pandemic does not justify poor quality reporting.
We acknowledge that there are numerous challenges associated with analyzing COVID-19 data during a pandemic [ 47 ]. High-quality evidence syntheses are needed for decision-making, but each type of evidence syntheses is associated with its inherent challenges.
The creation of classic systematic reviews requires considerable time and effort; with massive research output, they quickly become outdated, and preparing updated versions also requires considerable time. A recent study showed that updates of non-Cochrane systematic reviews are published a median of 5 years after the publication of the previous version [ 48 ].
Authors may register a review and then abandon it [ 49 ], but the existence of a public record that is not updated may lead other authors to believe that the review is still ongoing. A quarter of Cochrane review protocols remains unpublished as completed systematic reviews 8 years after protocol publication [ 50 ].
Rapid reviews can be used to summarize the evidence, but they involve methodological sacrifices and simplifications to produce information promptly, with inconsistent methodological approaches [ 51 ]. However, rapid reviews are justified in times of public health emergencies, and even Cochrane has resorted to publishing rapid reviews in response to the COVID-19 crisis [ 52 ]. Rapid reviews were eligible for inclusion in this overview, but only one of the 18 reviews included in this study was labeled as a rapid review.
Ideally, COVID-19 evidence would be continually summarized in a series of high-quality living systematic reviews, types of evidence synthesis defined as “ a systematic review which is continually updated, incorporating relevant new evidence as it becomes available ” [ 53 ]. However, conducting living systematic reviews requires considerable resources, calling into question the sustainability of such evidence synthesis over long periods [ 54 ].
Research reports about COVID-19 will contribute to research waste if they are poorly designed, poorly reported, or simply not necessary. In principle, systematic reviews should help reduce research waste as they usually provide recommendations for further research that is needed or may advise that sufficient evidence exists on a particular topic [ 55 ]. However, systematic reviews can also contribute to growing research waste when they are not needed, or poorly conducted and reported. Our present study clearly shows that most of the systematic reviews that were published early on in the COVID-19 pandemic could be categorized as research waste, as our confidence in their results is critically low.
Our study has some limitations. One is that for AMSTAR 2 assessment we relied on information available in publications; we did not attempt to contact study authors for clarifications or additional data. In three reviews, the methodological quality appraisal was challenging because they were published as letters, or labeled as rapid communications. As a result, various details about their review process were not included, leading to AMSTAR 2 questions being answered as “not reported”, resulting in low confidence scores. Full manuscripts might have provided additional information that could have led to higher confidence in the results. In other words, low scores could reflect incomplete reporting, not necessarily low-quality review methods. To make their review available more rapidly and more concisely, the authors may have omitted methodological details. A general issue during a crisis is that speed and completeness must be balanced. However, maintaining high standards requires proper resourcing and commitment to ensure that the users of systematic reviews can have high confidence in the results.
Furthermore, we used adjusted AMSTAR 2 scoring, as the tool was designed for critical appraisal of reviews of interventions. Some reviews may have received lower scores than actually warranted in spite of these adjustments.
Another limitation of our study may be the inclusion of multiple overlapping reviews, as some included reviews included the same primary studies. According to the Cochrane Handbook, including overlapping reviews may be appropriate when the review’s aim is “ to present and describe the current body of systematic review evidence on a topic ” [ 12 ], which was our aim. To avoid bias with summarizing evidence from overlapping reviews, we presented the forest plots without summary estimates. The forest plots serve to inform readers about the effect sizes for outcomes that were reported in each review.
Several authors from this study have contributed to one of the reviews identified [ 25 ]. To reduce the risk of any bias, two authors who did not co-author the review in question initially assessed its quality and limitations.
Finally, we note that the systematic reviews included in our overview may have had issues that our analysis did not identify because we did not analyze their primary studies to verify the accuracy of the data and information they presented. We give two examples to substantiate this possibility. Lovato et al. wrote a commentary on the review of Sun et al. [ 41 ], in which they criticized the authors’ conclusion that sore throat is rare in COVID-19 patients [ 56 ]. Lovato et al. highlighted that multiple studies included in Sun et al. did not accurately describe participants’ clinical presentations, warning that only three studies clearly reported data on sore throat [ 56 ].
In another example, Leung [ 57 ] warned about the review of Li, L.Q. et al. [ 29 ]: “ it is possible that this statistic was computed using overlapped samples, therefore some patients were double counted ”. Li et al. responded to Leung that it is uncertain whether the data overlapped, as they used data from published articles and did not have access to the original data; they also reported that they requested original data and that they plan to re-do their analyses once they receive them; they also urged readers to treat the data with caution [ 58 ]. This points to the evolving nature of evidence during a crisis.
Our study’s strength is that this overview adds to the current knowledge by providing a comprehensive summary of all the evidence synthesis about COVID-19 available early after the onset of the pandemic. This overview followed strict methodological criteria, including a comprehensive and sensitive search strategy and a standard tool for methodological appraisal of systematic reviews.
In conclusion, in this overview of systematic reviews, we analyzed evidence from the first 18 systematic reviews that were published after the emergence of COVID-19. However, confidence in the results of all the reviews was “critically low”. Thus, systematic reviews that were published early on in the pandemic could be categorized as research waste. Even during public health emergencies, studies and systematic reviews should adhere to established methodological standards to provide patients, clinicians, and decision-makers trustworthy evidence.
Availability of data and materials
All data collected and analyzed within this study are available from the corresponding author on reasonable request.
World Health Organization. Timeline - COVID-19: Available at: https://www.who.int/news/item/29-06-2020-covidtimeline . Accessed 1 June 2021.
COVID-19 Dashboard by the Center for Systems Science and Engineering (CSSE) at Johns Hopkins University (JHU). Available at: https://coronavirus.jhu.edu/map.html . Accessed 1 June 2021.
Anzai A, Kobayashi T, Linton NM, Kinoshita R, Hayashi K, Suzuki A, et al. Assessing the Impact of Reduced Travel on Exportation Dynamics of Novel Coronavirus Infection (COVID-19). J Clin Med. 2020;9(2):601.
Chinazzi M, Davis JT, Ajelli M, Gioannini C, Litvinova M, Merler S, et al. The effect of travel restrictions on the spread of the 2019 novel coronavirus (COVID-19) outbreak. Science. 2020;368(6489):395–400. https://doi.org/10.1126/science.aba9757 .
Article CAS PubMed PubMed Central Google Scholar
Fidahic M, Nujic D, Runjic R, Civljak M, Markotic F, Lovric Makaric Z, et al. Research methodology and characteristics of journal articles with original data, preprint articles and registered clinical trial protocols about COVID-19. BMC Med Res Methodol. 2020;20(1):161. https://doi.org/10.1186/s12874-020-01047-2 .
EPPI Centre . COVID-19: a living systematic map of the evidence. Available at: http://eppi.ioe.ac.uk/cms/Projects/DepartmentofHealthandSocialCare/Publishedreviews/COVID-19Livingsystematicmapoftheevidence/tabid/3765/Default.aspx . Accessed 1 June 2021.
NCBI SARS-CoV-2 Resources. Available at: https://www.ncbi.nlm.nih.gov/sars-cov-2/ . Accessed 1 June 2021.
Gustot T. Quality and reproducibility during the COVID-19 pandemic. JHEP Rep. 2020;2(4):100141. https://doi.org/10.1016/j.jhepr.2020.100141 .
Article PubMed PubMed Central Google Scholar
Kodvanj, I., et al., Publishing of COVID-19 Preprints in Peer-reviewed Journals, Preprinting Trends, Public Discussion and Quality Issues. Preprint article. bioRxiv 2020.11.23.394577; doi: https://doi.org/10.1101/2020.11.23.394577 .
Dobler CC. Poor quality research and clinical practice during COVID-19. Breathe (Sheff). 2020;16(2):200112. https://doi.org/10.1183/20734735.0112-2020 .
Article Google Scholar
Bastian H, Glasziou P, Chalmers I. Seventy-five trials and eleven systematic reviews a day: how will we ever keep up? PLoS Med. 2010;7(9):e1000326. https://doi.org/10.1371/journal.pmed.1000326 .
Lunny C, Brennan SE, McDonald S, McKenzie JE. Toward a comprehensive evidence map of overview of systematic review methods: paper 1-purpose, eligibility, search and data extraction. Syst Rev. 2017;6(1):231. https://doi.org/10.1186/s13643-017-0617-1 .
Pollock M, Fernandes RM, Becker LA, Pieper D, Hartling L. Chapter V: Overviews of Reviews. In: Higgins JPT, Thomas J, Chandler J, Cumpston M, Li T, Page MJ, Welch VA (editors). Cochrane Handbook for Systematic Reviews of Interventions version 6.1 (updated September 2020). Cochrane. 2020. Available from www.training.cochrane.org/handbook .
Higgins JPT, Thomas J, Chandler J, Cumpston M, Li T, Page MJ, et al. Cochrane handbook for systematic reviews of interventions version 6.1 (updated September 2020). Cochrane. 2020; Available from www.training.cochrane.org/handbook .
Pollock M, Fernandes RM, Newton AS, Scott SD, Hartling L. The impact of different inclusion decisions on the comprehensiveness and complexity of overviews of reviews of healthcare interventions. Syst Rev. 2019;8(1):18. https://doi.org/10.1186/s13643-018-0914-3 .
Pollock M, Fernandes RM, Newton AS, Scott SD, Hartling L. A decision tool to help researchers make decisions about including systematic reviews in overviews of reviews of healthcare interventions. Syst Rev. 2019;8(1):29. https://doi.org/10.1186/s13643-018-0768-8 .
Hunt H, Pollock A, Campbell P, Estcourt L, Brunton G. An introduction to overviews of reviews: planning a relevant research question and objective for an overview. Syst Rev. 2018;7(1):39. https://doi.org/10.1186/s13643-018-0695-8 .
Pollock M, Fernandes RM, Pieper D, Tricco AC, Gates M, Gates A, et al. Preferred reporting items for overviews of reviews (PRIOR): a protocol for development of a reporting guideline for overviews of reviews of healthcare interventions. Syst Rev. 2019;8(1):335. https://doi.org/10.1186/s13643-019-1252-9 .
Moher D, Liberati A, Tetzlaff J, Altman DG, PRISMA Group. Preferred reporting items for systematic reviews and meta-analyses: the PRISMA statement. Open Med. 2009;3(3):e123–30.
Krnic Martinic M, Pieper D, Glatt A, Puljak L. Definition of a systematic review used in overviews of systematic reviews, meta-epidemiological studies and textbooks. BMC Med Res Methodol. 2019;19(1):203. https://doi.org/10.1186/s12874-019-0855-0 .
Puljak L. If there is only one author or only one database was searched, a study should not be called a systematic review. J Clin Epidemiol. 2017;91:4–5. https://doi.org/10.1016/j.jclinepi.2017.08.002 .
Article PubMed Google Scholar
Gates M, Gates A, Guitard S, Pollock M, Hartling L. Guidance for overviews of reviews continues to accumulate, but important challenges remain: a scoping review. Syst Rev. 2020;9(1):254. https://doi.org/10.1186/s13643-020-01509-0 .
Covidence - systematic review software. Available at: https://www.covidence.org/ . Accessed 1 June 2021.
Shea BJ, Reeves BC, Wells G, Thuku M, Hamel C, Moran J, et al. AMSTAR 2: a critical appraisal tool for systematic reviews that include randomised or non-randomised studies of healthcare interventions, or both. BMJ. 2017;358:j4008.
Borges do Nascimento IJ, et al. Novel Coronavirus Infection (COVID-19) in Humans: A Scoping Review and Meta-Analysis. J Clin Med. 2020;9(4):941.
Article PubMed Central Google Scholar
Adhikari SP, Meng S, Wu YJ, Mao YP, Ye RX, Wang QZ, et al. Epidemiology, causes, clinical manifestation and diagnosis, prevention and control of coronavirus disease (COVID-19) during the early outbreak period: a scoping review. Infect Dis Poverty. 2020;9(1):29. https://doi.org/10.1186/s40249-020-00646-x .
Cortegiani A, Ingoglia G, Ippolito M, Giarratano A, Einav S. A systematic review on the efficacy and safety of chloroquine for the treatment of COVID-19. J Crit Care. 2020;57:279–83. https://doi.org/10.1016/j.jcrc.2020.03.005 .
Li B, Yang J, Zhao F, Zhi L, Wang X, Liu L, et al. Prevalence and impact of cardiovascular metabolic diseases on COVID-19 in China. Clin Res Cardiol. 2020;109(5):531–8. https://doi.org/10.1007/s00392-020-01626-9 .
Article CAS PubMed Google Scholar
Li LQ, Huang T, Wang YQ, Wang ZP, Liang Y, Huang TB, et al. COVID-19 patients’ clinical characteristics, discharge rate, and fatality rate of meta-analysis. J Med Virol. 2020;92(6):577–83. https://doi.org/10.1002/jmv.25757 .
Lippi G, Lavie CJ, Sanchis-Gomar F. Cardiac troponin I in patients with coronavirus disease 2019 (COVID-19): evidence from a meta-analysis. Prog Cardiovasc Dis. 2020;63(3):390–1. https://doi.org/10.1016/j.pcad.2020.03.001 .
Lippi G, Henry BM. Active smoking is not associated with severity of coronavirus disease 2019 (COVID-19). Eur J Intern Med. 2020;75:107–8. https://doi.org/10.1016/j.ejim.2020.03.014 .
Lippi G, Plebani M. Procalcitonin in patients with severe coronavirus disease 2019 (COVID-19): a meta-analysis. Clin Chim Acta. 2020;505:190–1. https://doi.org/10.1016/j.cca.2020.03.004 .
Lippi G, Plebani M, Henry BM. Thrombocytopenia is associated with severe coronavirus disease 2019 (COVID-19) infections: a meta-analysis. Clin Chim Acta. 2020;506:145–8. https://doi.org/10.1016/j.cca.2020.03.022 .
Ludvigsson JF. Systematic review of COVID-19 in children shows milder cases and a better prognosis than adults. Acta Paediatr. 2020;109(6):1088–95. https://doi.org/10.1111/apa.15270 .
Lupia T, Scabini S, Mornese Pinna S, di Perri G, de Rosa FG, Corcione S. 2019 novel coronavirus (2019-nCoV) outbreak: a new challenge. J Glob Antimicrob Resist. 2020;21:22–7. https://doi.org/10.1016/j.jgar.2020.02.021 .
Marasinghe, K.M., A systematic review investigating the effectiveness of face mask use in limiting the spread of COVID-19 among medically not diagnosed individuals: shedding light on current recommendations provided to individuals not medically diagnosed with COVID-19. Research Square. Preprint article. doi : https://doi.org/10.21203/rs.3.rs-16701/v1 . 2020 .
Mullins E, Evans D, Viner RM, O’Brien P, Morris E. Coronavirus in pregnancy and delivery: rapid review. Ultrasound Obstet Gynecol. 2020;55(5):586–92. https://doi.org/10.1002/uog.22014 .
Pang J, Wang MX, Ang IYH, Tan SHX, Lewis RF, Chen JIP, et al. Potential Rapid Diagnostics, Vaccine and Therapeutics for 2019 Novel coronavirus (2019-nCoV): a systematic review. J Clin Med. 2020;9(3):623.
Rodriguez-Morales AJ, Cardona-Ospina JA, Gutiérrez-Ocampo E, Villamizar-Peña R, Holguin-Rivera Y, Escalera-Antezana JP, et al. Clinical, laboratory and imaging features of COVID-19: a systematic review and meta-analysis. Travel Med Infect Dis. 2020;34:101623. https://doi.org/10.1016/j.tmaid.2020.101623 .
Salehi S, Abedi A, Balakrishnan S, Gholamrezanezhad A. Coronavirus disease 2019 (COVID-19): a systematic review of imaging findings in 919 patients. AJR Am J Roentgenol. 2020;215(1):87–93. https://doi.org/10.2214/AJR.20.23034 .
Sun P, Qie S, Liu Z, Ren J, Li K, Xi J. Clinical characteristics of hospitalized patients with SARS-CoV-2 infection: a single arm meta-analysis. J Med Virol. 2020;92(6):612–7. https://doi.org/10.1002/jmv.25735 .
Yang J, Zheng Y, Gou X, Pu K, Chen Z, Guo Q, et al. Prevalence of comorbidities and its effects in patients infected with SARS-CoV-2: a systematic review and meta-analysis. Int J Infect Dis. 2020;94:91–5. https://doi.org/10.1016/j.ijid.2020.03.017 .
Bassetti M, Vena A, Giacobbe DR. The novel Chinese coronavirus (2019-nCoV) infections: challenges for fighting the storm. Eur J Clin Investig. 2020;50(3):e13209. https://doi.org/10.1111/eci.13209 .
Article CAS Google Scholar
Hwang CS. Olfactory neuropathy in severe acute respiratory syndrome: report of a case. Acta Neurol Taiwanica. 2006;15(1):26–8.
Google Scholar
Suzuki M, Saito K, Min WP, Vladau C, Toida K, Itoh H, et al. Identification of viruses in patients with postviral olfactory dysfunction. Laryngoscope. 2007;117(2):272–7. https://doi.org/10.1097/01.mlg.0000249922.37381.1e .
Rajgor DD, Lee MH, Archuleta S, Bagdasarian N, Quek SC. The many estimates of the COVID-19 case fatality rate. Lancet Infect Dis. 2020;20(7):776–7. https://doi.org/10.1016/S1473-3099(20)30244-9 .
Wolkewitz M, Puljak L. Methodological challenges of analysing COVID-19 data during the pandemic. BMC Med Res Methodol. 2020;20(1):81. https://doi.org/10.1186/s12874-020-00972-6 .
Rombey T, Lochner V, Puljak L, Könsgen N, Mathes T, Pieper D. Epidemiology and reporting characteristics of non-Cochrane updates of systematic reviews: a cross-sectional study. Res Synth Methods. 2020;11(3):471–83. https://doi.org/10.1002/jrsm.1409 .
Runjic E, Rombey T, Pieper D, Puljak L. Half of systematic reviews about pain registered in PROSPERO were not published and the majority had inaccurate status. J Clin Epidemiol. 2019;116:114–21. https://doi.org/10.1016/j.jclinepi.2019.08.010 .
Runjic E, Behmen D, Pieper D, Mathes T, Tricco AC, Moher D, et al. Following Cochrane review protocols to completion 10 years later: a retrospective cohort study and author survey. J Clin Epidemiol. 2019;111:41–8. https://doi.org/10.1016/j.jclinepi.2019.03.006 .
Tricco AC, Antony J, Zarin W, Strifler L, Ghassemi M, Ivory J, et al. A scoping review of rapid review methods. BMC Med. 2015;13(1):224. https://doi.org/10.1186/s12916-015-0465-6 .
COVID-19 Rapid Reviews: Cochrane’s response so far. Available at: https://training.cochrane.org/resource/covid-19-rapid-reviews-cochrane-response-so-far . Accessed 1 June 2021.
Cochrane. Living systematic reviews. Available at: https://community.cochrane.org/review-production/production-resources/living-systematic-reviews . Accessed 1 June 2021.
Millard T, Synnot A, Elliott J, Green S, McDonald S, Turner T. Feasibility and acceptability of living systematic reviews: results from a mixed-methods evaluation. Syst Rev. 2019;8(1):325. https://doi.org/10.1186/s13643-019-1248-5 .
Babic A, Poklepovic Pericic T, Pieper D, Puljak L. How to decide whether a systematic review is stable and not in need of updating: analysis of Cochrane reviews. Res Synth Methods. 2020;11(6):884–90. https://doi.org/10.1002/jrsm.1451 .
Lovato A, Rossettini G, de Filippis C. Sore throat in COVID-19: comment on “clinical characteristics of hospitalized patients with SARS-CoV-2 infection: a single arm meta-analysis”. J Med Virol. 2020;92(7):714–5. https://doi.org/10.1002/jmv.25815 .
Leung C. Comment on Li et al: COVID-19 patients’ clinical characteristics, discharge rate, and fatality rate of meta-analysis. J Med Virol. 2020;92(9):1431–2. https://doi.org/10.1002/jmv.25912 .
Li LQ, Huang T, Wang YQ, Wang ZP, Liang Y, Huang TB, et al. Response to Char’s comment: comment on Li et al: COVID-19 patients’ clinical characteristics, discharge rate, and fatality rate of meta-analysis. J Med Virol. 2020;92(9):1433. https://doi.org/10.1002/jmv.25924 .
Download references
Acknowledgments
We thank Catherine Henderson DPhil from Swanscoe Communications for pro bono medical writing and editing support. We acknowledge support from the Covidence Team, specifically Anneliese Arno. We thank the whole International Network of Coronavirus Disease 2019 (InterNetCOVID-19) for their commitment and involvement. Members of the InterNetCOVID-19 are listed in Additional file 6 . We thank Pavel Cerny and Roger Crosthwaite for guiding the team supervisor (IJBN) on human resources management.
This research received no external funding.
Author information
Authors and affiliations.
University Hospital and School of Medicine, Universidade Federal de Minas Gerais, Belo Horizonte, Minas Gerais, Brazil
Israel Júnior Borges do Nascimento & Milena Soriano Marcolino
Medical College of Wisconsin, Milwaukee, WI, USA
Israel Júnior Borges do Nascimento
Helene Fuld Health Trust National Institute for Evidence-based Practice in Nursing and Healthcare, College of Nursing, The Ohio State University, Columbus, OH, USA
Dónal P. O’Mathúna
School of Nursing, Psychotherapy and Community Health, Dublin City University, Dublin, Ireland
Department of Anesthesiology, Intensive Care and Pain Medicine, University of Münster, Münster, Germany
Thilo Caspar von Groote
Department of Sport and Health Science, Technische Universität München, Munich, Germany
Hebatullah Mohamed Abdulazeem
School of Health Sciences, Faculty of Health and Medicine, The University of Newcastle, Callaghan, Australia
Ishanka Weerasekara
Department of Physiotherapy, Faculty of Allied Health Sciences, University of Peradeniya, Peradeniya, Sri Lanka
Cochrane Croatia, University of Split, School of Medicine, Split, Croatia
Ana Marusic, Irena Zakarija-Grkovic & Tina Poklepovic Pericic
Center for Evidence-Based Medicine and Health Care, Catholic University of Croatia, Ilica 242, 10000, Zagreb, Croatia
Livia Puljak
Cochrane Brazil, Evidence-Based Health Program, Universidade Federal de São Paulo, São Paulo, Brazil
Vinicius Tassoni Civile & Alvaro Nagib Atallah
Yorkville University, Fredericton, New Brunswick, Canada
Santino Filoso
Laboratory for Industrial and Applied Mathematics (LIAM), Department of Mathematics and Statistics, York University, Toronto, Ontario, Canada
Nicola Luigi Bragazzi
You can also search for this author in PubMed Google Scholar
Contributions
IJBN conceived the research idea and worked as a project coordinator. DPOM, TCVG, HMA, IW, AM, LP, VTC, IZG, TPP, ANA, SF, NLB and MSM were involved in data curation, formal analysis, investigation, methodology, and initial draft writing. All authors revised the manuscript critically for the content. The author(s) read and approved the final manuscript.
Corresponding author
Correspondence to Livia Puljak .
Ethics declarations
Ethics approval and consent to participate.
Not required as data was based on published studies.
Consent for publication
Not applicable.
Competing interests
The authors declare no conflict of interest.
Additional information
Publisher’s note.
Springer Nature remains neutral with regard to jurisdictional claims in published maps and institutional affiliations.
Supplementary Information
Additional file 1: appendix 1..
Search strategies used in the study.
Additional file 2: Appendix 2.
Adjusted scoring of AMSTAR 2 used in this study for systematic reviews of studies that did not analyze interventions.
Additional file 3: Appendix 3.
List of excluded studies, with reasons.
Additional file 4: Appendix 4.
Table of overlapping studies, containing the list of primary studies included, their visual overlap in individual systematic reviews, and the number in how many reviews each primary study was included.
Additional file 5: Appendix 5.
A detailed explanation of AMSTAR scoring for each item in each review.
Additional file 6: Appendix 6.
List of members and affiliates of International Network of Coronavirus Disease 2019 (InterNetCOVID-19).
Rights and permissions
Open Access This article is licensed under a Creative Commons Attribution 4.0 International License, which permits use, sharing, adaptation, distribution and reproduction in any medium or format, as long as you give appropriate credit to the original author(s) and the source, provide a link to the Creative Commons licence, and indicate if changes were made. The images or other third party material in this article are included in the article's Creative Commons licence, unless indicated otherwise in a credit line to the material. If material is not included in the article's Creative Commons licence and your intended use is not permitted by statutory regulation or exceeds the permitted use, you will need to obtain permission directly from the copyright holder. To view a copy of this licence, visit http://creativecommons.org/licenses/by/4.0/ . The Creative Commons Public Domain Dedication waiver ( http://creativecommons.org/publicdomain/zero/1.0/ ) applies to the data made available in this article, unless otherwise stated in a credit line to the data.
Reprints and permissions
About this article
Cite this article.
Borges do Nascimento, I.J., O’Mathúna, D.P., von Groote, T.C. et al. Coronavirus disease (COVID-19) pandemic: an overview of systematic reviews. BMC Infect Dis 21 , 525 (2021). https://doi.org/10.1186/s12879-021-06214-4
Download citation
Received : 12 April 2020
Accepted : 19 May 2021
Published : 04 June 2021
DOI : https://doi.org/10.1186/s12879-021-06214-4
Share this article
Anyone you share the following link with will be able to read this content:
Sorry, a shareable link is not currently available for this article.
Provided by the Springer Nature SharedIt content-sharing initiative
- Coronavirus
- Evidence-based medicine
- Infectious diseases
BMC Infectious Diseases
ISSN: 1471-2334
- General enquiries: [email protected]
Coronavirus disease 2019 (COVID-19): A literature review
Affiliations.
- 1 Medical Research Unit, School of Medicine, Universitas Syiah Kuala, Banda Aceh, Indonesia; Tropical Disease Centre, School of Medicine, Universitas Syiah Kuala, Banda Aceh, Indonesia; Department of Microbiology, School of Medicine, Universitas Syiah Kuala, Banda Aceh, Indonesia. Electronic address: [email protected].
- 2 Division of Infectious Diseases, AichiCancer Center Hospital, Chikusa-ku Nagoya, Japan. Electronic address: [email protected].
- 3 Department of Family Medicine, School of Medicine, Universitas Syiah Kuala, Banda Aceh, Indonesia. Electronic address: [email protected].
- 4 Department of Pulmonology and Respiratory Medicine, School of Medicine, Universitas Syiah Kuala, Banda Aceh, Indonesia. Electronic address: [email protected].
- 5 School of Medicine, The University of Western Australia, Perth, Australia. Electronic address: [email protected].
- 6 Siem Reap Provincial Health Department, Ministry of Health, Siem Reap, Cambodia. Electronic address: [email protected].
- 7 Department of Microbiology and Parasitology, Faculty of Medicine and Health Sciences, Warmadewa University, Denpasar, Indonesia; Department of Medical Microbiology and Immunology, University of California, Davis, CA, USA. Electronic address: [email protected].
- 8 Medical Research Unit, School of Medicine, Universitas Syiah Kuala, Banda Aceh, Indonesia; Tropical Disease Centre, School of Medicine, Universitas Syiah Kuala, Banda Aceh, Indonesia; Department of Microbiology, School of Medicine, Universitas Syiah Kuala, Banda Aceh, Indonesia; Department of Clinical Microbiology, School of Medicine, Universitas Syiah Kuala, Banda Aceh, Indonesia. Electronic address: [email protected].
- 9 Department of Epidemiology, University of Michigan, Ann Arbor, Michigan, MI 48109, USA. Electronic address: [email protected].
- 10 Medical Research Unit, School of Medicine, Universitas Syiah Kuala, Banda Aceh, Indonesia; Tropical Disease Centre, School of Medicine, Universitas Syiah Kuala, Banda Aceh, Indonesia; Department of Microbiology, School of Medicine, Universitas Syiah Kuala, Banda Aceh, Indonesia. Electronic address: [email protected].
- PMID: 32340833
- PMCID: PMC7142680
- DOI: 10.1016/j.jiph.2020.03.019
In early December 2019, an outbreak of coronavirus disease 2019 (COVID-19), caused by a novel severe acute respiratory syndrome coronavirus 2 (SARS-CoV-2), occurred in Wuhan City, Hubei Province, China. On January 30, 2020 the World Health Organization declared the outbreak as a Public Health Emergency of International Concern. As of February 14, 2020, 49,053 laboratory-confirmed and 1,381 deaths have been reported globally. Perceived risk of acquiring disease has led many governments to institute a variety of control measures. We conducted a literature review of publicly available information to summarize knowledge about the pathogen and the current epidemic. In this literature review, the causative agent, pathogenesis and immune responses, epidemiology, diagnosis, treatment and management of the disease, control and preventions strategies are all reviewed.
Keywords: 2019-nCoV; COVID-19; Novel coronavirus; Outbreak; SARS-CoV-2.
Copyright © 2020 The Authors. Published by Elsevier Ltd.. All rights reserved.
Publication types
- Betacoronavirus
- Clinical Trials as Topic
- Coronavirus Infections* / epidemiology
- Coronavirus Infections* / immunology
- Coronavirus Infections* / therapy
- Coronavirus Infections* / virology
- Disease Outbreaks* / prevention & control
- Pneumonia, Viral* / epidemiology
- Pneumonia, Viral* / immunology
- Pneumonia, Viral* / therapy
- Pneumonia, Viral* / virology
- - Google Chrome
Intended for healthcare professionals
- Access provided by Google Indexer
- My email alerts
- BMA member login
- Username * Password * Forgot your log in details? Need to activate BMA Member Log In Log in via OpenAthens Log in via your institution

Search form
- Advanced search
- Search responses
- Search blogs
- Virology, transmission...
Virology, transmission, and pathogenesis of SARS-CoV-2
Read our latest coverage of the coronavirus outbreak.
- Related content
- Peer review
- Muge Cevik , clinical lecturer 1 2 ,
- Krutika Kuppalli , assistant professor 3 ,
- Jason Kindrachuk , assistant professor of virology 4 ,
- Malik Peiris , professor of virology 5
- 1 Division of Infection and Global Health Research, School of Medicine, University of St Andrews, St Andrews, UK
- 2 Specialist Virology Laboratory, Royal Infirmary of Edinburgh, Edinburgh, UK and Regional Infectious Diseases Unit, Western General Hospital, Edinburgh, UK
- 3 Division of Infectious Diseases, Medical University of South Carolina, Charleston, SC, USA
- 4 Laboratory of Emerging and Re-Emerging Viruses, Department of Medical Microbiology, University of Manitoba, Winnipeg, MB, Canada
- 5 School of Public Health, LKS Faculty of Medicine, The University of Hong Kong, Hong Kong Special Administrative Region, China
- Correspondence to M Cevik mc349{at}st-andrews.ac.uk
What you need to know
SARS-CoV-2 is genetically similar to SARS-CoV-1, but characteristics of SARS-CoV-2—eg, structural differences in its surface proteins and viral load kinetics—may help explain its enhanced rate of transmission
In the respiratory tract, peak SARS-CoV-2 load is observed at the time of symptom onset or in the first week of illness, with subsequent decline thereafter, indicating the highest infectiousness potential just before or within the first five days of symptom onset
Reverse transcription polymerase chain reaction (RT-PCR) tests can detect viral SARS-CoV-2 RNA in the upper respiratory tract for a mean of 17 days; however, detection of viral RNA does not necessarily equate to infectiousness, and viral culture from PCR positive upper respiratory tract samples has been rarely positive beyond nine days of illness
Symptomatic and pre-symptomatic transmission (1-2 days before symptom onset), is likely to play a greater role in the spread of SARS-CoV-2 than asymptomatic transmission
A wide range of virus-neutralising antibodies have been reported, and emerging evidence suggests that these may correlate with severity of illness but wane over time
Since the emergence of SARS-CoV-2 in December 2019, there has been an unparalleled global effort to characterise the virus and the clinical course of disease. Coronavirus disease 2019 (covid-19), caused by SARS-CoV-2, follows a biphasic pattern of illness that likely results from the combination of an early viral response phase and an inflammatory second phase. Most clinical presentations are mild, and the typical pattern of covid-19 more resembles an influenza-like illness—which includes fever, cough, malaise, myalgia, headache, and taste and smell disturbance—rather than severe pneumonia (although emerging evidence about long term consequences is yet to be understood in detail). 1 In this review, we provide a broad update on the emerging understanding of SARS-CoV-2 pathophysiology, including virology, transmission dynamics, and the immune response to the virus. Any of the mechanisms and assumptions discussed in the article and in our understanding of covid-19 may be revised as further evidence emerges.
What we know about the virus
SARS-CoV-2 is an enveloped β-coronavirus, with a genetic sequence very similar to SARS-CoV-1 (80%) and bat coronavirus RaTG13 (96.2%). 2 The viral envelope is coated by spike (S) glycoprotein, envelope (E), and membrane (M) proteins ( fig 1 ). Host cell binding and entry are mediated by the S protein. The first step in infection is virus binding to a host cell through its target receptor. The S1 sub-unit of the S protein contains the receptor binding domain that binds to the peptidase domain of angiotensin-converting enzyme 2 (ACE 2). In SARS-CoV-2 the S2 sub-unit is highly preserved and is considered a potential antiviral target. The virus structure and replication cycle are described in figure 1 .

(1) The virus binds to ACE 2 as the host target cell receptor in synergy with the host’s transmembrane serine protease 2 (cell surface protein), which is principally expressed in the airway epithelial cells and vascular endothelial cells. This leads to membrane fusion and releases the viral genome into the host cytoplasm (2). Stages (3-7) show the remaining steps of viral replication, leading to viral assembly, maturation, and virus release
- Download figure
- Open in new tab
- Download powerpoint
Coronaviruses have the capacity for proofreading during replication, and therefore mutation rates are lower than in other RNA viruses. As SARS-CoV-2 has spread globally it has, like other viruses, accumulated some mutations in the viral genome, which contains geographic signatures. Researchers have examined these mutations to study virus characterisation and understand epidemiology and transmission patterns. In general, the mutations have not been attributed to phenotypic changes affecting viral transmissibility or pathogenicity. The G614 variant in the S protein has been postulated to increase infectivity and transmissibility of the virus. 3 Higher viral loads were reported in clinical samples with virus containing G614 than previously circulating variant D614, although no association was made with severity of illness as measured by hospitalisation outcomes. 3 These findings have yet to be confirmed with regards to natural infection.
Why is SARS-CoV-2 more infectious than SARS-CoV-1?
SARS-CoV-2 has a higher reproductive number (R 0 ) than SARS-CoV-1, indicating much more efficient spread. 1 Several characteristics of SARS-CoV-2 may help explain this enhanced transmission. While both SARS-CoV-1 and SARS-CoV-2 preferentially interact with the angiotensin-converting enzyme 2 (ACE 2) receptor, SARS-CoV-2 has structural differences in its surface proteins that enable stronger binding to the ACE 2 receptor 4 and greater efficiency at invading host cells. 1 SARS-CoV-2 also has greater affinity (or bonding) for the upper respiratory tract and conjunctiva, 5 thus can infect the upper respiratory tract and can conduct airways more easily. 6
Viral load dynamics and duration of infectiousness
Viral load kinetics could also explain some of the differences between SARS-CoV-2 and SARS-CoV-1. In the respiratory tract, peak SARS-CoV-2 load is observed at the time of symptom onset or in the first week of illness, with subsequent decline thereafter, which indicates the highest infectiousness potential just before or within the first five days of symptom onset ( fig 2 ). 7 In contrast, in SARS-CoV-1 the highest viral loads were detected in the upper respiratory tract in the second week of illness, which explains its minimal contagiousness in the first week after symptom onset, enabling early case detection in the community. 7

After the initial exposure, patients typically develop symptoms within 5-6 days (incubation period). SARS-CoV-2 generates a diverse range of clinical manifestations, ranging from mild infection to severe disease accompanied by high mortality. In patients with mild infection, initial host immune response is capable of controlling the infection. In severe disease, excessive immune response leads to organ damage, intensive care admission, or death. The viral load peaks in the first week of infection, declines thereafter gradually, while the antibody response gradually increases and is often detectable by day 14 (figure adapted with permission from https://www.sciencedirect.com/science/article/pii/S009286742030475X ; https://www.thelancet.com/journals/lanres/article/PIIS2213-2600(20)30230-7/fulltext )
Quantitative reverse transcription polymerase chain reaction (qRT-PCR) technology can detect viral SARS-CoV-2 RNA in the upper respiratory tract for a mean of 17 days (maximum 83 days) after symptom onset. 7 However, detection of viral RNA by qRT-PCR does not necessarily equate to infectiousness, and viral culture from PCR positive upper respiratory tract samples has been rarely positive beyond nine days of illness. 5 This corresponds to what is known about transmission based on contact tracing studies, which is that transmission capacity is maximal in the first week of illness, and that transmission after this period has not been documented. 8 Severely ill or immune-compromised patients may have relatively prolonged virus shedding, and some patients may have intermittent RNA shedding; however, low level results close to the detection limit may not constitute infectious viral particles. While asymptomatic individuals (those with no symptoms throughout the infection) can transmit the infection, their relative degree of infectiousness seems to be limited. 9 10 11 People with mild symptoms (paucisymptomatic) and those whose symptom have not yet appeared still carry large amounts of virus in the upper respiratory tract, which might contribute to the easy and rapid spread of SARS-CoV-2. 7 Symptomatic and pre-symptomatic transmission (one to two days before symptom onset) is likely to play a greater role in the spread of SARS-CoV-2. 10 12 A combination of preventive measures, such as physical distancing and testing, tracing, and self-isolation, continue to be needed.
Route of transmission and transmission dynamics
Like other coronaviruses, the primary mechanism of transmission of SARS-CoV-2 is via infected respiratory droplets, with viral infection occurring by direct or indirect contact with nasal, conjunctival, or oral mucosa, when respiratory particles are inhaled or deposited on these mucous membranes. 6 Target host receptors are found mainly in the human respiratory tract epithelium, including the oropharynx and upper airway. The conjunctiva and gastrointestinal tracts are also susceptible to infection and may serve as transmission portals. 6
Transmission risk depends on factors such as contact pattern, environment, infectiousness of the host, and socioeconomic factors, as described elsewhere. 12 Most transmission occurs through close range contact (such as 15 minutes face to face and within 2 m), 13 and spread is especially efficient within households and through gatherings of family and friends. 12 Household secondary attack rates (the proportion of susceptible individuals who become infected within a group of susceptible contacts with a primary case) ranges from 4% to 35%. 12 Sleeping in the same room as, or being a spouse of an infected individual increases the risk of infection, but isolation of the infected person away from the family is related to lower risk of infection. 12 Other activities identified as high risk include dining in close proximity with the infected person, sharing food, and taking part in group activities 12 The risk of infection substantially increases in enclosed environments compared with outdoor settings. 12 For example, a systematic review of transmission clusters found that most superspreading events occurred indoors. 11 Aerosol transmission can still factor during prolonged stay in crowded, poorly ventilated indoor settings (meaning transmission could occur at a distance >2 m). 12 14 15 16 17
The role of faecal shedding in SARS-CoV-2 transmission and the extent of fomite (through inanimate surfaces) transmission also remain to be fully understood. Both SARS-CoV-2 and SARS-CoV-1 remain viable for many days on smooth surfaces (stainless steel, plastic, glass) and at lower temperature and humidity (eg, air conditioned environments). 18 19 Thus, transferring infection from contaminated surfaces to the mucosa of eyes, nose, and mouth via unwashed hands is a possible route of transmission. This route of transmission may contribute especially in facilities with communal areas, with increased likelihood of environmental contamination. However, both SARS-CoV-1 and SARS-CoV-2 are readily inactivated by commonly used disinfectants, emphasising the potential value of surface cleaning and handwashing. SARS-CoV-2 RNA has been found in stool samples and RNA shedding often persists for longer than in respiratory samples 7 ; however, virus isolation has rarely been successful from the stool. 5 7 No published reports describe faecal-oral transmission. In SARS-CoV-1, faecal-oral transmission was not considered to occur in most circumstances; but, one explosive outbreak was attributed to aerosolisation and spread of the virus across an apartment block via a faulty sewage system. 20 It remains to be seen if similar transmission may occur with SARS-CoV-2.
Pathogenesis
Viral entry and interaction with target cells.
SARS-CoV-2 binds to ACE 2, the host target cell receptor. 1 Active replication and release of the virus in the lung cells lead to non-specific symptoms such as fever, myalgia, headache, and respiratory symptoms. 1 In an experimental hamster model, the virus causes transient damage to the cells in the olfactory epithelium, leading to olfactory dysfunction, which may explain temporary loss of taste and smell commonly seen in covid-19. 21 The distribution of ACE 2 receptors in different tissues may explain the sites of infection and patient symptoms. For example, the ACE 2 receptor is found on the epithelium of other organs such as the intestine and endothelial cells in the kidney and blood vessels, which may explain gastrointestinal symptoms and cardiovascular complications. 22 Lymphocytic endotheliitis has been observed in postmortem pathology examination of the lung, heart, kidney, and liver as well as liver cell necrosis and myocardial infarction in patients who died of covid-19. 1 23 These findings indicate that the virus directly affects many organs, as was seen in SARS-CoV-1 and influenzae.
Much remains unknown. Are the pathological changes in the respiratory tract or endothelial dysfunction the result of direct viral infection, cytokine dysregulation, coagulopathy, or are they multifactorial? And does direct viral invasion or coagulopathy directly contribute to some of the ischaemic complications such as ischaemic infarcts? These and more, will require further work to elucidate.
Immune response and disease spectrum ( figure 2 )
After viral entry, the initial inflammatory response attracts virus-specific T cells to the site of infection, where the infected cells are eliminated before the virus spreads, leading to recovery in most people. 24 In patients who develop severe disease, SARS-CoV-2 elicits an aberrant host immune response. 24 25 For example, postmortem histology of lung tissues of patients who died of covid-19 have confirmed the inflammatory nature of the injury, with features of bilateral diffuse alveolar damage, hyaline-membrane formation, interstitial mononuclear inflammatory infiltrates, and desquamation consistent with acute respiratory distress syndrome (ARDS), and is similar to the lung pathology seen in severe Middle East respiratory syndrome (MERS) and severe acute respiratory syndrome (SARS). 26 27 A distinctive feature of covid-19 is the presence of mucus plugs with fibrinous exudate in the respiratory tract, which may explain the severity of covid-19 even in young adults. 28 This is potentially caused by the overproduction of pro-inflammatory cytokines that accumulate in the lungs, eventually damaging the lung parenchyma. 24
Some patients also experience septic shock and multi-organ dysfunction. 24 For example, the cardiovascular system is often involved early in covid-19 disease and is reflected in the release of highly sensitive troponin and natriuretic peptides. 29 Consistent with the clinical context of coagulopathy, focal intra-alveolar haemorrhage and presence of platelet-fibrin thrombi in small arterial vessels is also seen. 27 Cytokines normally mediate and regulate immunity, inflammation, and haematopoiesis; however, further exacerbation of immune reaction and accumulation of cytokines in other organs in some patients may cause extensive tissue damage, or a cytokine release syndrome (cytokine storm), resulting in capillary leak, thrombus formation, and organ dysfunction. 24 30
Mechanisms underlying the diverse clinical outcomes
Clinical outcomes are influenced by host factors such as older age, male sex, and underlying medical conditions, 1 as well as factors related to the virus (such as viral load kinetics), host-immune response, and potential cross-reactive immune memory from previous exposure to seasonal coronaviruses ( box 1 ).
Risk factors associated with the development of severe disease, admission to intensive care unit, and mortality
Underlying condition.
Hypertension
Cardiovascular disease
Chronic obstructive pulmonary disease
Presentation
Higher fever (≥39°C on admission)
Dyspnoea on admission
Higher qSOFA score
Laboratory markers
Neutrophilia/lymphopenia
Raised lactate and lactate dehydrogenase
Raised C reactive protein
Raised ferritin
Raised IL-6
Raised ACE2
D-dimer >1 μg/mL
Sex-related differences in immune response have been reported, revealing that men had higher plasma innate immune cytokines and chemokines at baseline than women. 31 In contrast, women had notably more robust T cell activation than men, and among male participants T cell activation declined with age, which was sustained among female patients. These findings suggest that adaptive immune response may be important in defining the clinical outcome as older age and male sex is associated with increased risk of severe disease and mortality.
Increased levels of pro-inflammatory cytokines correlate with severe pneumonia and increased ground glass opacities within the lungs. 30 32 In people with severe illness, increased plasma concentrations of inflammatory cytokines and biomarkers were observed compared with people with non-severe illness. 30 33 34
Emerging evidence suggests a correlation between viral dynamics, the severity of illness, and disease outcome. 7 Longitudinal characteristics of immune response show a correlation between the severity of illness, viral load, and IFN- α, IFN-γ, and TNF-α response. 34 In the same study many interferons, cytokines, and chemokines were elevated early in disease for patients who had severe disease and higher viral loads. This emphasises that viral load may drive these cytokines and the possible pathological roles associated with the host defence factors. This is in keeping with the pathogenesis of influenza, SARS, and MERS whereby prolonged viral shedding was also associated with severity of illness. 7 35
Given the substantial role of the immune response in determining clinical outcomes, several immunosuppressive therapies aimed at limiting immune-mediated damage are currently in various phases of development ( table 1 ).
Therapeutics currently under investigation
- View inline
Immune response to the virus and its role in protection
Covid-19 leads to an antibody response to a range of viral proteins, but the spike (S) protein and nucleocapsid are those most often used in serological diagnosis. Few antibodies are detectable in the first four days of illness, but patients progressively develop them, with most achieving a detectable response after four weeks. 36 A wide range of virus-neutralising antibodies have been reported, and emerging evidence suggests that these may correlate with severity but wane over time. 37 The duration and protectivity of antibody and T cell responses remain to be defined through studies with longer follow-up. CD-4 T cell responses to endemic human coronaviruses appear to manifest cross-reactivity with SARS-CoV-2, but their role in protection remains unclear. 38
Unanswered questions
Further understanding of the pathogenesis for SARS-CoV-2 will be vital in developing therapeutics, vaccines, and supportive care modalities in the treatment of covid-19. More data are needed to understand the determinants of healthy versus dysfunctional response and immune markers for protection and the severity of disease. Neutralising antibodies are potential correlates of protection, but other protective antibody mechanisms may exist. Similarly, the protective role of T cell immunity and duration of both antibody and T cell responses and the correlates of protection need to be defined. In addition, we need optimal testing systems and technologies to support and inform early detection and clinical management of infection. Greater understanding is needed regarding the long term consequences following acute illness and multisystem inflammatory disease, especially in children.
Education into practice
How would you describe SARS-CoV-2 transmission routes and ways to prevent infection?
How would you describe to a patient why cough, anosmia, and fever occur in covid-19?
Questions for future research
What is the role of the cytokine storm and how could it inform the development of therapeutics, vaccines, and supportive care modalities?
What is the window period when patients are most infectious?
Why do some patients develop severe disease while others, especially children, remain mildly symptomatic or do not develop symptoms?
What are the determinants of healthy versus dysfunctional response, and the biomarkers to define immune correlates of protection and disease severity for the effective triage of patients?
What is the protective role of T cell immunity and duration of both antibody and T cell responses, and how would you define the correlates of protection?
How patients were involved in the creation of this article
No patients were directly involved in the creation of this article.
How this article was created
We searched PubMed from 2000 to 18 September 2020, limited to publications in English. Our search strategy used a combination of key words including “COVID-19,” “SARS-CoV-2,” “SARS”, “MERS,” “Coronavirus,” “Novel Coronavirus,” “Pathogenesis,” “Transmission,” “Cytokine Release,” “immune response,” “antibody response.” These sources were supplemented with systematic reviews. We also reviewed technical documents produced by the Centers for Disease Control and Prevention and World Health Organization technical documents.
Author contributions: MC, KK, JK, MP drafted the first and subsequent versions of the manuscript and all authors provided critical feedback and contributed to the manuscript.
Competing interests The BMJ has judged that there are no disqualifying financial ties to commercial companies. The authors declare the following other interests: none.
Further details of The BMJ policy on financial interests are here: https://www.bmj.com/about-bmj/resources-authors/forms-policies-and-checklists/declaration-competing-interests
Provenance and peer review: commissioned; externally peer reviewed.
This article is made freely available for use in accordance with BMJ's website terms and conditions for the duration of the covid-19 pandemic or until otherwise determined by BMJ. You may use, download and print the article for any lawful, non-commercial purpose (including text and data mining) provided that all copyright notices and trade marks are retained.
- Bamford CGG ,
- Fischer WM ,
- Gnanakaran S ,
- Sheffield COVID-19 Genomics Group
- Corbett KS ,
- Corman VM ,
- Guggemos W ,
- Cheung MC ,
- Perera RAPM ,
- Cheng H-Y ,
- Taiwan COVID-19 Outbreak Investigation Team
- Meyerowitz E ,
- Richterman A ,
- Nergiz AI ,
- Maraolo AE ,
- Buitrago-Garcia D ,
- Egli-Gany D ,
- Counotte MJ ,
- ↵ European Centre for Disease Prevention and Control. Surveillance definitions for COVID-19. 2020. https://www.ecdc.europa.eu/en/covid-19/surveillance/surveillance-definitions .
- Klompas M ,
- ↵ Centers for Disease Control and Prevention. How COVID-19 spreads. 2020. https://www.cdc.gov/coronavirus/2019-ncov/prevent-getting-sick/how-covid-spreads.html
- ↵ Centers for Disease Control and Prevention. Scientific brief: SARS-CoV-2 and potential airborne transmission. 2020. https://www.cdc.gov/coronavirus/2019-ncov/more/scientific-brief-sars-cov-2.html
- Perera MRA ,
- van Doremalen N ,
- Bushmaker T ,
- Morris DH ,
- Monteil V ,
- Flammer AJ ,
- Steiger P ,
- Mangalmurti N ,
- Blanco-Melo D ,
- Nilsson-Payant BE ,
- Carsana L ,
- Sonzogni A ,
- ↵ Takahashi T, Ellingson MK, Wong P, et al. Sex differences in immune responses that underlie COVID-19 disease outcomes. Nature 2020. https://pubmed.ncbi.nlm.nih.gov/32846427/
- Yale IMPACT Team
- CAP-China Network
- Perera RA ,
- Merrick B ,
- Grifoni A ,
- Weiskopf D ,
- Ramirez SI ,
- Search Search
- CN (Chinese)
- DE (German)
- ES (Spanish)
- FR (Français)
- JP (Japanese)
- Open Research
- Booksellers
- Peer Reviewers
- Springer Nature Group ↗
Coronavirus (COVID-19) Research Highlights
On the 5th may 2023 the who declared an end to the public health emergency of international concern for covid-19. this page remains as a point of reference for interested parties..
Springer Nature is committed to supporting the global response to COVID-19 enabling fast and direct access to the latest available research, evidence, and data.
The need for continued access to research and learning has never been more important. We recognise our role in this and we continue to work with global organisations, such as the World Health Organisation and the initiative from the White House Office of Science and Technology to make all relevant global research, and data, immediately available. We also continue to work directly with teachers, lecturers, librarians, students and institutions to support their work. See more from our CEO, Frank Vrancken Peeters on our response to the pandemic.
Across the pandemic* we:
- Enabled free access to over 70,000 articles, book chapters, reference works and protocols on our platforms
- Published over 67,000 new COVID-19 articles and supported all researchers in making their underlying experimental data sets available for free and re-use
- Made available, via Research Square, over 10,000 COVID-19 preprint articles
Below, you will find links to key resources, free content and updated information related to COVID-19, alongside key policies and information in supporting remote access and working. Please contact our Customer Services Team if you require further support.
* counted as 2019-2021
Free access to COVID-19 journal and book content
Remote access: information & resources for librarians, remote access: resources for institutions and students, remote access: learn about remote access for researchers, report: impact of covid-19 on the research enterprise, supporting covid-19 research, how can ai support the research community in times of crisis.
Learn how AI was used to build a rapid prototype report to give a quick and simple overview of coronavirus research.
In Review and Preprints
Springer Nature encourages early sharing of research submitted to all our journals through preprints, and our In Review preprint service is available for many journals.
For API purposes, we have made additional content free via the SN OpenAccess API.
Access COVID-19 Research Papers & Articles Across Journals, Books & More
Featured research, reviews and comment, books and chapters.
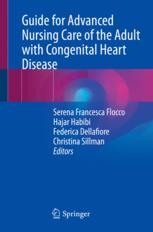
- More highlighted COVID-19 articles and chapters
- Explore registered COVID-19 Research Highlights Trials
- Access COVID-19 datasets
- Discover our Article Latest Collections
Access more COVID-19 research on our platforms
Featured blog posts from "the source" blog see all.
Providing insight and discussion from researchers during the pandemic
Research in the time of a pandemic: SENTINEL- A proactive, early warning system to pre-empt future pandemics
Dr Sabeti and Dr Happi talk about building a pandemic preemption system to safeguard us from future pandemics.
Research in the time of a pandemic: Coronavirus, Class and Mutual Aid in the UK
In this week's blog we discuss how the economic systems in place played a role into how the pandemic has developed.
Research in the time of a pandemic: Africa Centres for Disease Control and Prevention
Read about the Centres for Disease Control and Prevention in Africa and their role during the pandemic.
Research in the time of a pandemic: Leading the Oxford Vaccine Trial
Dr Sarah Gilbert, the lead for the Oxford Vaccine Trial for COVID-19 talks about the vaccine.
Read our blogs
The brain complications of COVID-19
The clinical trials fighting COVID-19: vaccines, testing and other conditions
How is the WHO trial registry network responding to the COVID-19 pandemic?
The clinical trials fighting COVID-19: platform and repurposed drug trials
Keep up with the latest news on covid-19 research, nature briefing.
Sign up to a free daily update on COVID-19, plus a selection of the most important non-coronavirus science news
Nature provides authoritative, timely news and opinion articles on the novel coronavirus and COVID-19
Scientific American International
Scientific American’s international network of magazines now brings you the latest science news and expert analysis on the pandemic in one place
Additional Resources
Adisinsight.
AdisInsight provides profiles of drug development programs actively investigating treatments for Coronavirus and summaries of the clinical trials being conducted for those drugs.
Nano – a Nature Research Solution
Access manually extracted nanomaterial summaries in addition to thousands of freely available patents and article links on the interaction, diagnostics and therapeutic effects of nanomaterials on coronavirus.
Springer Nature Experiments
Covers over 230 well established protocols and methods for identifying & detecting coronavirus, protocols on designing the vaccine for the epidemic, understanding the ultrastructure of viruses to design new drugs.
External resources
Stay up to date.
Here to foster information exchange with the library community
Connect with us on LinkedIn and stay up to date with news and development.
- Tools & Services
- Account Development
- Sales and account contacts
- Professional
- Press office
- Locations & Contact
We are a world leading research, educational and professional publisher. Visit our main website for more information.
- © 2024 Springer Nature
- General terms and conditions
- Your US State Privacy Rights
- Your Privacy Choices / Manage Cookies
- Accessibility
- Legal notice
- Help us to improve this site, send feedback.
- Introduction
- Article Information
First surge was defined as February to June 2020; second surge, October 2020 to March 2021; third surge, June to October 2021; fourth surge, November 2021 to March 2022.
eMethods. Supplementary Methods
Data Sharing Statement
- Health Equity for US Asian, Native Hawaiian, and Pacific Islander Populations JAMA Network Open Invited Commentary May 21, 2024 Naoko Muramatsu, PhD; Marshall H. Chin, MD, MPH
See More About
Sign up for emails based on your interests, select your interests.
Customize your JAMA Network experience by selecting one or more topics from the list below.
- Academic Medicine
- Acid Base, Electrolytes, Fluids
- Allergy and Clinical Immunology
- American Indian or Alaska Natives
- Anesthesiology
- Anticoagulation
- Art and Images in Psychiatry
- Artificial Intelligence
- Assisted Reproduction
- Bleeding and Transfusion
- Caring for the Critically Ill Patient
- Challenges in Clinical Electrocardiography
- Climate and Health
- Climate Change
- Clinical Challenge
- Clinical Decision Support
- Clinical Implications of Basic Neuroscience
- Clinical Pharmacy and Pharmacology
- Complementary and Alternative Medicine
- Consensus Statements
- Coronavirus (COVID-19)
- Critical Care Medicine
- Cultural Competency
- Dental Medicine
- Dermatology
- Diabetes and Endocrinology
- Diagnostic Test Interpretation
- Drug Development
- Electronic Health Records
- Emergency Medicine
- End of Life, Hospice, Palliative Care
- Environmental Health
- Equity, Diversity, and Inclusion
- Facial Plastic Surgery
- Gastroenterology and Hepatology
- Genetics and Genomics
- Genomics and Precision Health
- Global Health
- Guide to Statistics and Methods
- Hair Disorders
- Health Care Delivery Models
- Health Care Economics, Insurance, Payment
- Health Care Quality
- Health Care Reform
- Health Care Safety
- Health Care Workforce
- Health Disparities
- Health Inequities
- Health Policy
- Health Systems Science
- History of Medicine
- Hypertension
- Images in Neurology
- Implementation Science
- Infectious Diseases
- Innovations in Health Care Delivery
- JAMA Infographic
- Law and Medicine
- Leading Change
- Less is More
- LGBTQIA Medicine
- Lifestyle Behaviors
- Medical Coding
- Medical Devices and Equipment
- Medical Education
- Medical Education and Training
- Medical Journals and Publishing
- Mobile Health and Telemedicine
- Narrative Medicine
- Neuroscience and Psychiatry
- Notable Notes
- Nutrition, Obesity, Exercise
- Obstetrics and Gynecology
- Occupational Health
- Ophthalmology
- Orthopedics
- Otolaryngology
- Pain Medicine
- Palliative Care
- Pathology and Laboratory Medicine
- Patient Care
- Patient Information
- Performance Improvement
- Performance Measures
- Perioperative Care and Consultation
- Pharmacoeconomics
- Pharmacoepidemiology
- Pharmacogenetics
- Pharmacy and Clinical Pharmacology
- Physical Medicine and Rehabilitation
- Physical Therapy
- Physician Leadership
- Population Health
- Primary Care
- Professional Well-being
- Professionalism
- Psychiatry and Behavioral Health
- Public Health
- Pulmonary Medicine
- Regulatory Agencies
- Reproductive Health
- Research, Methods, Statistics
- Resuscitation
- Rheumatology
- Risk Management
- Scientific Discovery and the Future of Medicine
- Shared Decision Making and Communication
- Sleep Medicine
- Sports Medicine
- Stem Cell Transplantation
- Substance Use and Addiction Medicine
- Surgical Innovation
- Surgical Pearls
- Teachable Moment
- Technology and Finance
- The Art of JAMA
- The Arts and Medicine
- The Rational Clinical Examination
- Tobacco and e-Cigarettes
- Translational Medicine
- Trauma and Injury
- Treatment Adherence
- Ultrasonography
- Users' Guide to the Medical Literature
- Vaccination
- Venous Thromboembolism
- Veterans Health
- Women's Health
- Workflow and Process
- Wound Care, Infection, Healing
Get the latest research based on your areas of interest.
Others also liked.
- Download PDF
- X Facebook More LinkedIn
Sumibcay JRC , Kunichoff D , Bassett MT. Racial and Ethnic Disparities in COVID-19 Mortality. JAMA Netw Open. 2024;7(5):e2411656. doi:10.1001/jamanetworkopen.2024.11656
Manage citations:
© 2024
- Permissions
Racial and Ethnic Disparities in COVID-19 Mortality
- 1 FXB Center for Health and Human Rights, Harvard T.H. Chan School of Public Health, Boston, Massachusetts
- 2 Department of Health Policy and Management, Harvard T.H. Chan School of Public Health, Boston, Massachusetts
- Invited Commentary Health Equity for US Asian, Native Hawaiian, and Pacific Islander Populations Naoko Muramatsu, PhD; Marshall H. Chin, MD, MPH JAMA Network Open
In May 2023, the United States ended the federal COVID-19 Public Health Emergency (PHE) with more than 1.1 million reported deaths. 1 American Indian or Alaska Native and Native Hawaiian or Pacific Islander individuals represent numerically small populations (constituting 1.3% and 0.3% of the total US population, 2 respectively). Their high mortality rates have minimal impact on national rates and may receive little attention. 3 To uncover these disparities, we analyzed separately the racial and ethnic mortality trends during the PHE, focusing on surge periods.
This cross-sectional analysis used publicly available COVID-19 mortality data from the National Center for Health Statistics 4 from February 2020 to September 2023 for 6 defined racial and ethnic groups: Hispanic, non-Hispanic American Indian or Alaska Native, non-Hispanic Asian, non-Hispanic Black, non-Hispanic Native Hawaiian or Pacific Islander, and non-Hispanic White. We added an additional category combining non-Hispanic Asian and non-Hispanic Native Hawaiian or Pacific Islander to examine the effect of the historical Asian–Pacific Islander grouping. 3 Cumulative age-adjusted mortality rates per 100 000 individuals were calculated for each racial group over 4 periods (February to June 2020, October 2020 to March 2021, June to October 2021, and November 2021 to March 2022), selected to correspond to the surges of COVID-19 mortality. We computed rate ratios with 95% CIs for each specified period, using non-Hispanic White individuals as the reference group. All analyses were performed using R version 4.2.2 (R Core Team 2022). Additional details are provided in the eMethods in Supplement 1 . This study was deemed not human participant research by the Harvard Longwood Campus institutional review board at Harvard University; therefore, the requirement for informed consent was waived. We followed the STROBE reporting guidelines.
During the examined period, the number of COVID-19 deaths equaled 172 129 for Hispanic individuals, 12 113 for non-Hispanic American Indian or Alaska Native individuals, 35 392 for non-Hispanic Asian individuals, 157 072 for non-Hispanic Black individuals, 2321 for non-Hispanic Native Hawaiian or Pacific Islander individuals, and 758 221 for non-Hispanic White individuals; the corresponding population sizes were 60.6 million, 2.4 million, 19.7 million, 41.9 million, 0.6 million, and 196.8 million, respectively ( Table ). Non-Hispanic Black, Hispanic, non-Hispanic American Indian or Alaska Native, and non-Hispanic Native Hawaiian or Pacific Islander groups experienced higher mortality than non-Hispanic White individuals, with disparities widening during surges. Non-Hispanic American Indian or Alaska Native and non-Hispanic Native Hawaiian or Pacific Islander groups consistently exhibited higher mortality rates compared with all other racial and ethnic groups during the second, third, and fourth periods ( Figure ). Throughout, non-Hispanic American Indian or Alaska Native individuals experienced the highest mortality rates, and Hispanic, non-Hispanic Native Hawaiian or Pacific Islander, and non-Hispanic Black populations followed closely ( Table ). Non-Hispanic Native Hawaiian or Pacific Islander individuals had the highest mortality rate among all groups during the third time interval, yielding the largest rate ratio when compared with non-Hispanic White individuals ( Table ). In contrast, non-Hispanic Asian individuals displayed the lowest mortality rates during the second, third, and fourth periods, with the second-lowest rates during the first period ( Table ). Non-Hispanic Asian and non-Hispanic Native Hawaiian or Pacific Islander groups combined exhibited similar results to the non-Hispanic Asian group alone ( Table ).
This analysis underscores the persistently high mortality risk for American Indian or Alaska Native and Native Hawaiian or Pacific Islander individuals during the PHE, exacerbated by the emergence of new variants of COVID-19. Limitations, such as data gaps, underreporting, and the misclassification of race and ethnicity (eg, death records), may lead to underestimating rates for specific groups like American Indian or Alaska Native, Native Hawaiian or Pacific Islander, and multiracial populations. 5 Encouraging consistent use and examination of racial and ethnic disaggregated data are critical to elucidating disparities. Combining Native Hawaiian or Pacific Islander individuals with Asian individuals, a group with lower mortality, masked Native Hawaiian or Pacific Islander persistent mortality risk, cautioning against the Asian–Pacific Islander categorization. The absence of further granular data limited the assessment of within-group differences. Aggregated data hamper the identification of crucial patterns, hindering the development of targeted interventions, such as financial resources and community-driven, culturally sensitive initiatives. It is noteworthy that 2 groups (American Indian or Alaska Native, Native Hawaiian or Pacific Islander) with persistently high COVID-19 mortality represent Indigenous populations subjected to racism and colonialism, suggesting the influence of historical trauma and genocidal policies. 6 Investigating the unique and upstream factors influencing mortality is critical to producing accurate epidemiological accounts of smaller groups with greater risk.
Accepted for Publication: March 13, 2024.
Published: May 21, 2024. doi:10.1001/jamanetworkopen.2024.11656
Open Access: This is an open access article distributed under the terms of the CC-BY License . © 2024 Sumibcay JRC et al. JAMA Network Open .
Corresponding Author: Jake Ryann C. Sumibcay, DrPH, MPH, FXB Center for Health and Human Rights, Harvard T.H. Chan School of Public Health, 651 Huntington Ave, Boston, MA 02115 ( [email protected] ).
Author Contributions: Mr Kunichoff had full access to all of the data in the study and takes responsibility for the integrity of the data and the accuracy of the data analysis.
Concept and design: All authors.
Acquisition, analysis, or interpretation of data: Sumibcay, Kunichoff.
Drafting of the manuscript: All authors.
Critical review of the manuscript for important intellectual content: All authors.
Statistical analysis: Sumibcay, Kunichoff.
Obtained funding: Sumibcay.
Administrative, technical, or material support: Sumibcay.
Supervision: Sumibcay, Bassett.
Conflict of Interest Disclosures: Dr Sumibcay reported receiving grants from JPB Foundation outside the submitted work. No other disclosures were reported.
Data Sharing Statement: See Supplement 2 .
- Register for email alerts with links to free full-text articles
- Access PDFs of free articles
- Manage your interests
- Save searches and receive search alerts

An official website of the United States government
The .gov means it’s official. Federal government websites often end in .gov or .mil. Before sharing sensitive information, make sure you’re on a federal government site.
The site is secure. The https:// ensures that you are connecting to the official website and that any information you provide is encrypted and transmitted securely.
- Publications
- Account settings
Preview improvements coming to the PMC website in October 2024. Learn More or Try it out now .
- Advanced Search
- Journal List
- Springer Nature - PMC COVID-19 Collection

A Review of Coronavirus Disease-2019 (COVID-19)
Tanu singhal.
Department of Pediatrics and Infectious Disease, Kokilaben Dhirubhai Ambani Hospital and Medical Research Institute, Mumbai, India
There is a new public health crises threatening the world with the emergence and spread of 2019 novel coronavirus (2019-nCoV) or the severe acute respiratory syndrome coronavirus 2 (SARS-CoV-2). The virus originated in bats and was transmitted to humans through yet unknown intermediary animals in Wuhan, Hubei province, China in December 2019. There have been around 96,000 reported cases of coronavirus disease 2019 (COVID-2019) and 3300 reported deaths to date (05/03/2020). The disease is transmitted by inhalation or contact with infected droplets and the incubation period ranges from 2 to 14 d. The symptoms are usually fever, cough, sore throat, breathlessness, fatigue, malaise among others. The disease is mild in most people; in some (usually the elderly and those with comorbidities), it may progress to pneumonia, acute respiratory distress syndrome (ARDS) and multi organ dysfunction. Many people are asymptomatic. The case fatality rate is estimated to range from 2 to 3%. Diagnosis is by demonstration of the virus in respiratory secretions by special molecular tests. Common laboratory findings include normal/ low white cell counts with elevated C-reactive protein (CRP). The computerized tomographic chest scan is usually abnormal even in those with no symptoms or mild disease. Treatment is essentially supportive; role of antiviral agents is yet to be established. Prevention entails home isolation of suspected cases and those with mild illnesses and strict infection control measures at hospitals that include contact and droplet precautions. The virus spreads faster than its two ancestors the SARS-CoV and Middle East respiratory syndrome coronavirus (MERS-CoV), but has lower fatality. The global impact of this new epidemic is yet uncertain.
Introduction
The 2019 novel coronavirus (2019-nCoV) or the severe acute respiratory syndrome corona virus 2 (SARS-CoV-2) as it is now called, is rapidly spreading from its origin in Wuhan City of Hubei Province of China to the rest of the world [ 1 ]. Till 05/03/2020 around 96,000 cases of coronavirus disease 2019 (COVID-19) and 3300 deaths have been reported [ 2 ]. India has reported 29 cases till date. Fortunately so far, children have been infrequently affected with no deaths. But the future course of this virus is unknown. This article gives a bird’s eye view about this new virus. Since knowledge about this virus is rapidly evolving, readers are urged to update themselves regularly.
Coronaviruses are enveloped positive sense RNA viruses ranging from 60 nm to 140 nm in diameter with spike like projections on its surface giving it a crown like appearance under the electron microscope; hence the name coronavirus [ 3 ]. Four corona viruses namely HKU1, NL63, 229E and OC43 have been in circulation in humans, and generally cause mild respiratory disease.
There have been two events in the past two decades wherein crossover of animal betacorona viruses to humans has resulted in severe disease. The first such instance was in 2002–2003 when a new coronavirus of the β genera and with origin in bats crossed over to humans via the intermediary host of palm civet cats in the Guangdong province of China. This virus, designated as severe acute respiratory syndrome coronavirus affected 8422 people mostly in China and Hong Kong and caused 916 deaths (mortality rate 11%) before being contained [ 4 ]. Almost a decade later in 2012, the Middle East respiratory syndrome coronavirus (MERS-CoV), also of bat origin, emerged in Saudi Arabia with dromedary camels as the intermediate host and affected 2494 people and caused 858 deaths (fatality rate 34%) [ 5 ].
Origin and Spread of COVID-19 [ 1 , 2 , 6 ]
In December 2019, adults in Wuhan, capital city of Hubei province and a major transportation hub of China started presenting to local hospitals with severe pneumonia of unknown cause. Many of the initial cases had a common exposure to the Huanan wholesale seafood market that also traded live animals. The surveillance system (put into place after the SARS outbreak) was activated and respiratory samples of patients were sent to reference labs for etiologic investigations. On December 31st 2019, China notified the outbreak to the World Health Organization and on 1st January the Huanan sea food market was closed. On 7th January the virus was identified as a coronavirus that had >95% homology with the bat coronavirus and > 70% similarity with the SARS- CoV. Environmental samples from the Huanan sea food market also tested positive, signifying that the virus originated from there [ 7 ]. The number of cases started increasing exponentially, some of which did not have exposure to the live animal market, suggestive of the fact that human-to-human transmission was occurring [ 8 ]. The first fatal case was reported on 11th Jan 2020. The massive migration of Chinese during the Chinese New Year fuelled the epidemic. Cases in other provinces of China, other countries (Thailand, Japan and South Korea in quick succession) were reported in people who were returning from Wuhan. Transmission to healthcare workers caring for patients was described on 20th Jan, 2020. By 23rd January, the 11 million population of Wuhan was placed under lock down with restrictions of entry and exit from the region. Soon this lock down was extended to other cities of Hubei province. Cases of COVID-19 in countries outside China were reported in those with no history of travel to China suggesting that local human-to-human transmission was occurring in these countries [ 9 ]. Airports in different countries including India put in screening mechanisms to detect symptomatic people returning from China and placed them in isolation and testing them for COVID-19. Soon it was apparent that the infection could be transmitted from asymptomatic people and also before onset of symptoms. Therefore, countries including India who evacuated their citizens from Wuhan through special flights or had travellers returning from China, placed all people symptomatic or otherwise in isolation for 14 d and tested them for the virus.
Cases continued to increase exponentially and modelling studies reported an epidemic doubling time of 1.8 d [ 10 ]. In fact on the 12th of February, China changed its definition of confirmed cases to include patients with negative/ pending molecular tests but with clinical, radiologic and epidemiologic features of COVID-19 leading to an increase in cases by 15,000 in a single day [ 6 ]. As of 05/03/2020 96,000 cases worldwide (80,000 in China) and 87 other countries and 1 international conveyance (696, in the cruise ship Diamond Princess parked off the coast of Japan) have been reported [ 2 ]. It is important to note that while the number of new cases has reduced in China lately, they have increased exponentially in other countries including South Korea, Italy and Iran. Of those infected, 20% are in critical condition, 25% have recovered, and 3310 (3013 in China and 297 in other countries) have died [ 2 ]. India, which had reported only 3 cases till 2/3/2020, has also seen a sudden spurt in cases. By 5/3/2020, 29 cases had been reported; mostly in Delhi, Jaipur and Agra in Italian tourists and their contacts. One case was reported in an Indian who traveled back from Vienna and exposed a large number of school children in a birthday party at a city hotel. Many of the contacts of these cases have been quarantined.
These numbers are possibly an underestimate of the infected and dead due to limitations of surveillance and testing. Though the SARS-CoV-2 originated from bats, the intermediary animal through which it crossed over to humans is uncertain. Pangolins and snakes are the current suspects.
Epidemiology and Pathogenesis [ 10 , 11 ]
All ages are susceptible. Infection is transmitted through large droplets generated during coughing and sneezing by symptomatic patients but can also occur from asymptomatic people and before onset of symptoms [ 9 ]. Studies have shown higher viral loads in the nasal cavity as compared to the throat with no difference in viral burden between symptomatic and asymptomatic people [ 12 ]. Patients can be infectious for as long as the symptoms last and even on clinical recovery. Some people may act as super spreaders; a UK citizen who attended a conference in Singapore infected 11 other people while staying in a resort in the French Alps and upon return to the UK [ 6 ]. These infected droplets can spread 1–2 m and deposit on surfaces. The virus can remain viable on surfaces for days in favourable atmospheric conditions but are destroyed in less than a minute by common disinfectants like sodium hypochlorite, hydrogen peroxide etc. [ 13 ]. Infection is acquired either by inhalation of these droplets or touching surfaces contaminated by them and then touching the nose, mouth and eyes. The virus is also present in the stool and contamination of the water supply and subsequent transmission via aerosolization/feco oral route is also hypothesized [ 6 ]. As per current information, transplacental transmission from pregnant women to their fetus has not been described [ 14 ]. However, neonatal disease due to post natal transmission is described [ 14 ]. The incubation period varies from 2 to 14 d [median 5 d]. Studies have identified angiotensin receptor 2 (ACE 2 ) as the receptor through which the virus enters the respiratory mucosa [ 11 ].
The basic case reproduction rate (BCR) is estimated to range from 2 to 6.47 in various modelling studies [ 11 ]. In comparison, the BCR of SARS was 2 and 1.3 for pandemic flu H1N1 2009 [ 2 ].
Clinical Features [ 8 , 15 – 18 ]
The clinical features of COVID-19 are varied, ranging from asymptomatic state to acute respiratory distress syndrome and multi organ dysfunction. The common clinical features include fever (not in all), cough, sore throat, headache, fatigue, headache, myalgia and breathlessness. Conjunctivitis has also been described. Thus, they are indistinguishable from other respiratory infections. In a subset of patients, by the end of the first week the disease can progress to pneumonia, respiratory failure and death. This progression is associated with extreme rise in inflammatory cytokines including IL2, IL7, IL10, GCSF, IP10, MCP1, MIP1A, and TNFα [ 15 ]. The median time from onset of symptoms to dyspnea was 5 d, hospitalization 7 d and acute respiratory distress syndrome (ARDS) 8 d. The need for intensive care admission was in 25–30% of affected patients in published series. Complications witnessed included acute lung injury, ARDS, shock and acute kidney injury. Recovery started in the 2nd or 3rd wk. The median duration of hospital stay in those who recovered was 10 d. Adverse outcomes and death are more common in the elderly and those with underlying co-morbidities (50–75% of fatal cases). Fatality rate in hospitalized adult patients ranged from 4 to 11%. The overall case fatality rate is estimated to range between 2 and 3% [ 2 ].
Interestingly, disease in patients outside Hubei province has been reported to be milder than those from Wuhan [ 17 ]. Similarly, the severity and case fatality rate in patients outside China has been reported to be milder [ 6 ]. This may either be due to selection bias wherein the cases reporting from Wuhan included only the severe cases or due to predisposition of the Asian population to the virus due to higher expression of ACE 2 receptors on the respiratory mucosa [ 11 ].
Disease in neonates, infants and children has been also reported to be significantly milder than their adult counterparts. In a series of 34 children admitted to a hospital in Shenzhen, China between January 19th and February 7th, there were 14 males and 20 females. The median age was 8 y 11 mo and in 28 children the infection was linked to a family member and 26 children had history of travel/residence to Hubei province in China. All the patients were either asymptomatic (9%) or had mild disease. No severe or critical cases were seen. The most common symptoms were fever (50%) and cough (38%). All patients recovered with symptomatic therapy and there were no deaths. One case of severe pneumonia and multiorgan dysfunction in a child has also been reported [ 19 ]. Similarly the neonatal cases that have been reported have been mild [ 20 ].
Diagnosis [ 21 ]
A suspect case is defined as one with fever, sore throat and cough who has history of travel to China or other areas of persistent local transmission or contact with patients with similar travel history or those with confirmed COVID-19 infection. However cases may be asymptomatic or even without fever. A confirmed case is a suspect case with a positive molecular test.
Specific diagnosis is by specific molecular tests on respiratory samples (throat swab/ nasopharyngeal swab/ sputum/ endotracheal aspirates and bronchoalveolar lavage). Virus may also be detected in the stool and in severe cases, the blood. It must be remembered that the multiplex PCR panels currently available do not include the COVID-19. Commercial tests are also not available at present. In a suspect case in India, the appropriate sample has to be sent to designated reference labs in India or the National Institute of Virology in Pune. As the epidemic progresses, commercial tests will become available.
Other laboratory investigations are usually non specific. The white cell count is usually normal or low. There may be lymphopenia; a lymphocyte count <1000 has been associated with severe disease. The platelet count is usually normal or mildly low. The CRP and ESR are generally elevated but procalcitonin levels are usually normal. A high procalcitonin level may indicate a bacterial co-infection. The ALT/AST, prothrombin time, creatinine, D-dimer, CPK and LDH may be elevated and high levels are associated with severe disease.
The chest X-ray (CXR) usually shows bilateral infiltrates but may be normal in early disease. The CT is more sensitive and specific. CT imaging generally shows infiltrates, ground glass opacities and sub segmental consolidation. It is also abnormal in asymptomatic patients/ patients with no clinical evidence of lower respiratory tract involvement. In fact, abnormal CT scans have been used to diagnose COVID-19 in suspect cases with negative molecular diagnosis; many of these patients had positive molecular tests on repeat testing [ 22 ].
Differential Diagnosis [ 21 ]
The differential diagnosis includes all types of respiratory viral infections [influenza, parainfluenza, respiratory syncytial virus (RSV), adenovirus, human metapneumovirus, non COVID-19 coronavirus], atypical organisms (mycoplasma, chlamydia) and bacterial infections. It is not possible to differentiate COVID-19 from these infections clinically or through routine lab tests. Therefore travel history becomes important. However, as the epidemic spreads, the travel history will become irrelevant.
Treatment [ 21 , 23 ]
Treatment is essentially supportive and symptomatic.
The first step is to ensure adequate isolation (discussed later) to prevent transmission to other contacts, patients and healthcare workers. Mild illness should be managed at home with counseling about danger signs. The usual principles are maintaining hydration and nutrition and controlling fever and cough. Routine use of antibiotics and antivirals such as oseltamivir should be avoided in confirmed cases. In hypoxic patients, provision of oxygen through nasal prongs, face mask, high flow nasal cannula (HFNC) or non-invasive ventilation is indicated. Mechanical ventilation and even extra corporeal membrane oxygen support may be needed. Renal replacement therapy may be needed in some. Antibiotics and antifungals are required if co-infections are suspected or proven. The role of corticosteroids is unproven; while current international consensus and WHO advocate against their use, Chinese guidelines do recommend short term therapy with low-to-moderate dose corticosteroids in COVID-19 ARDS [ 24 , 25 ]. Detailed guidelines for critical care management for COVID-19 have been published by the WHO [ 26 ]. There is, as of now, no approved treatment for COVID-19. Antiviral drugs such as ribavirin, lopinavir-ritonavir have been used based on the experience with SARS and MERS. In a historical control study in patients with SARS, patients treated with lopinavir-ritonavir with ribavirin had better outcomes as compared to those given ribavirin alone [ 15 ].
In the case series of 99 hospitalized patients with COVID-19 infection from Wuhan, oxygen was given to 76%, non-invasive ventilation in 13%, mechanical ventilation in 4%, extracorporeal membrane oxygenation (ECMO) in 3%, continuous renal replacement therapy (CRRT) in 9%, antibiotics in 71%, antifungals in 15%, glucocorticoids in 19% and intravenous immunoglobulin therapy in 27% [ 15 ]. Antiviral therapy consisting of oseltamivir, ganciclovir and lopinavir-ritonavir was given to 75% of the patients. The duration of non-invasive ventilation was 4–22 d [median 9 d] and mechanical ventilation for 3–20 d [median 17 d]. In the case series of children discussed earlier, all children recovered with basic treatment and did not need intensive care [ 17 ].
There is anecdotal experience with use of remdeswir, a broad spectrum anti RNA drug developed for Ebola in management of COVID-19 [ 27 ]. More evidence is needed before these drugs are recommended. Other drugs proposed for therapy are arbidol (an antiviral drug available in Russia and China), intravenous immunoglobulin, interferons, chloroquine and plasma of patients recovered from COVID-19 [ 21 , 28 , 29 ]. Additionally, recommendations about using traditional Chinese herbs find place in the Chinese guidelines [ 21 ].
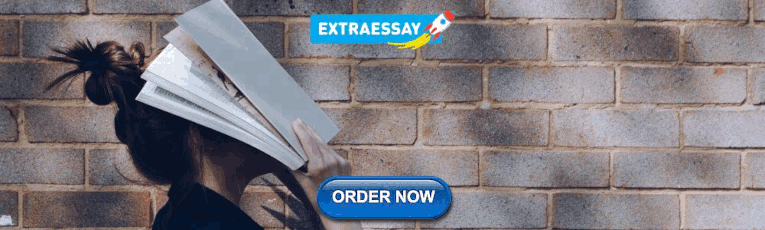
Prevention [ 21 , 30 ]
Since at this time there are no approved treatments for this infection, prevention is crucial. Several properties of this virus make prevention difficult namely, non-specific features of the disease, the infectivity even before onset of symptoms in the incubation period, transmission from asymptomatic people, long incubation period, tropism for mucosal surfaces such as the conjunctiva, prolonged duration of the illness and transmission even after clinical recovery.
Isolation of confirmed or suspected cases with mild illness at home is recommended. The ventilation at home should be good with sunlight to allow for destruction of virus. Patients should be asked to wear a simple surgical mask and practice cough hygiene. Caregivers should be asked to wear a surgical mask when in the same room as patient and use hand hygiene every 15–20 min.
The greatest risk in COVID-19 is transmission to healthcare workers. In the SARS outbreak of 2002, 21% of those affected were healthcare workers [ 31 ]. Till date, almost 1500 healthcare workers in China have been infected with 6 deaths. The doctor who first warned about the virus has died too. It is important to protect healthcare workers to ensure continuity of care and to prevent transmission of infection to other patients. While COVID-19 transmits as a droplet pathogen and is placed in Category B of infectious agents (highly pathogenic H5N1 and SARS), by the China National Health Commission, infection control measures recommended are those for category A agents (cholera, plague). Patients should be placed in separate rooms or cohorted together. Negative pressure rooms are not generally needed. The rooms and surfaces and equipment should undergo regular decontamination preferably with sodium hypochlorite. Healthcare workers should be provided with fit tested N95 respirators and protective suits and goggles. Airborne transmission precautions should be taken during aerosol generating procedures such as intubation, suction and tracheostomies. All contacts including healthcare workers should be monitored for development of symptoms of COVID-19. Patients can be discharged from isolation once they are afebrile for atleast 3 d and have two consecutive negative molecular tests at 1 d sampling interval. This recommendation is different from pandemic flu where patients were asked to resume work/school once afebrile for 24 h or by day 7 of illness. Negative molecular tests were not a prerequisite for discharge.
At the community level, people should be asked to avoid crowded areas and postpone non-essential travel to places with ongoing transmission. They should be asked to practice cough hygiene by coughing in sleeve/ tissue rather than hands and practice hand hygiene frequently every 15–20 min. Patients with respiratory symptoms should be asked to use surgical masks. The use of mask by healthy people in public places has not shown to protect against respiratory viral infections and is currently not recommended by WHO. However, in China, the public has been asked to wear masks in public and especially in crowded places and large scale gatherings are prohibited (entertainment parks etc). China is also considering introducing legislation to prohibit selling and trading of wild animals [ 32 ].
The international response has been dramatic. Initially, there were massive travel restrictions to China and people returning from China/ evacuated from China are being evaluated for clinical symptoms, isolated and tested for COVID-19 for 2 wks even if asymptomatic. However, now with rapid world wide spread of the virus these travel restrictions have extended to other countries. Whether these efforts will lead to slowing of viral spread is not known.
A candidate vaccine is under development.
Practice Points from an Indian Perspective
At the time of writing this article, the risk of coronavirus in India is extremely low. But that may change in the next few weeks. Hence the following is recommended:
- Healthcare providers should take travel history of all patients with respiratory symptoms, and any international travel in the past 2 wks as well as contact with sick people who have travelled internationally.
- They should set up a system of triage of patients with respiratory illness in the outpatient department and give them a simple surgical mask to wear. They should use surgical masks themselves while examining such patients and practice hand hygiene frequently.
- Suspected cases should be referred to government designated centres for isolation and testing (in Mumbai, at this time, it is Kasturba hospital). Commercial kits for testing are not yet available in India.
- Patients admitted with severe pneumonia and acute respiratory distress syndrome should be evaluated for travel history and placed under contact and droplet isolation. Regular decontamination of surfaces should be done. They should be tested for etiology using multiplex PCR panels if logistics permit and if no pathogen is identified, refer the samples for testing for SARS-CoV-2.
- All clinicians should keep themselves updated about recent developments including global spread of the disease.
- Non-essential international travel should be avoided at this time.
- People should stop spreading myths and false information about the disease and try to allay panic and anxiety of the public.
Conclusions
This new virus outbreak has challenged the economic, medical and public health infrastructure of China and to some extent, of other countries especially, its neighbours. Time alone will tell how the virus will impact our lives here in India. More so, future outbreaks of viruses and pathogens of zoonotic origin are likely to continue. Therefore, apart from curbing this outbreak, efforts should be made to devise comprehensive measures to prevent future outbreaks of zoonotic origin.
Compliance with Ethical Standards
Publisher’s Note
Springer Nature remains neutral with regard to jurisdictional claims in published maps and institutional affiliations.
Thank you for visiting nature.com. You are using a browser version with limited support for CSS. To obtain the best experience, we recommend you use a more up to date browser (or turn off compatibility mode in Internet Explorer). In the meantime, to ensure continued support, we are displaying the site without styles and JavaScript.
- View all journals
- Explore content
- About the journal
- Publish with us
- Sign up for alerts
- Published: 16 June 2020
COVID-19 impact on research, lessons learned from COVID-19 research, implications for pediatric research
- Debra L. Weiner 1 , 2 ,
- Vivek Balasubramaniam 3 ,
- Shetal I. Shah 4 &
- Joyce R. Javier 5 , 6
on behalf of the Pediatric Policy Council
Pediatric Research volume 88 , pages 148–150 ( 2020 ) Cite this article
147k Accesses
81 Citations
19 Altmetric
Metrics details
The COVID-19 pandemic has resulted in unprecedented research worldwide. The impact on research in progress at the time of the pandemic, the importance and challenges of real-time pandemic research, and the importance of a pediatrician-scientist workforce are all highlighted by this epic pandemic. As we navigate through and beyond this pandemic, which will have a long-lasting impact on our world, including research and the biomedical research enterprise, it is important to recognize and address opportunities and strategies for, and challenges of research and strengthening the pediatrician-scientist workforce.
The first cases of what is now recognized as SARS-CoV-2 infection, termed COVID-19, were reported in Wuhan, China in December 2019 as cases of fatal pneumonia. By February 26, 2020, COVID-19 had been reported on all continents except Antarctica. As of May 4, 2020, 3.53 million cases and 248,169 deaths have been reported from 210 countries. 1
Impact of COVID-19 on ongoing research
The impact on research in progress prior to COVID-19 was rapid, dramatic, and no doubt will be long term. The pandemic curtailed most academic, industry, and government basic science and clinical research, or redirected research to COVID-19. Most clinical trials, except those testing life-saving therapies, have been paused, and most continuing trials are now closed to new enrollment. Ongoing clinical trials have been modified to enable home administration of treatment and virtual monitoring to minimize participant risk of COVID-19 infection, and to avoid diverting healthcare resources from pandemic response. In addition to short- and long-term patient impact, these research disruptions threaten the careers of physician-scientists, many of whom have had to shift efforts from research to patient care. To protect research in progress, as well as physician-scientist careers and the research workforce, ongoing support is critical. NIH ( https://grants.nih.gov/policy/natural-disasters/corona-virus.htm ), PCORI ( https://www.pcori.org/funding-opportunities/applicant-and-awardee-faqs-related-covid-19 ), and other funders acted swiftly to provide guidance on proposal submission and award management, and implement allowances that enable grant personnel to be paid and time lines to be relaxed. Research institutions have also implemented strategies to mitigate the long-term impact of research disruptions. Support throughout and beyond the pandemic to retain currently well-trained research personnel and research support teams, and to accommodate loss of research assets, including laboratory supplies and study participants, will be required to complete disrupted research and ultimately enable new research.
In the long term, it is likely that the pandemic will force reallocation of research dollars at the expense of research areas funded prior to the pandemic. It will be more important than ever for the pediatric research community to engage in discussion and decisions regarding prioritization of funding goals for dedicated pediatric research and meaningful inclusion of children in studies. The recently released 2020 National Institute of Child Health and Development (NICHD) strategic plan that engaged stakeholders, including scientists and patients, to shape the goals of the Institute, will require modification to best chart a path toward restoring normalcy within pediatric science.
COVID-19 research
This global pandemic once again highlights the importance of research, stable research infrastructure, and funding for public health emergency (PHE)/disaster preparedness, response, and resiliency. The stakes in this worldwide pandemic have never been higher as lives are lost, economies falter, and life has radically changed. Ultimate COVID-19 mitigation and crisis resolution is dependent on high-quality research aligned with top priority societal goals that yields trustworthy data and actionable information. While the highest priority goals are treatment and prevention, biomedical research also provides data critical to manage and restore economic and social welfare.
Scientific and technological knowledge and resources have never been greater and have been leveraged globally to perform COVID-19 research at warp speed. The number of studies related to COVID-19 increases daily, the scope and magnitude of engagement is stunning, and the extent of global collaboration unprecedented. On January 5, 2020, just weeks after the first cases of illness were reported, the genetic sequence, which identified the pathogen as a novel coronavirus, SARS-CoV-2, was released, providing information essential for identifying and developing treatments, vaccines, and diagnostics. As of May 3, 2020 1133 COVID-19 studies, including 148 related to hydroxychloroquine, 13 to remdesivir, 50 to vaccines, and 100 to diagnostic testing, were registered on ClinicalTrials.gov, and 980 different studies on the World Health Organization’s International Clinical Trials Registry Platform (WHO ICTRP), made possible, at least in part, by use of data libraries to inform development of antivirals, immunomodulators, antibody-based biologics, and vaccines. On April 7, 2020, the FDA launched the Coronavirus Treatment Acceleration Program (CTAP) ( https://www.fda.gov/drugs/coronavirus-covid-19-drugs/coronavirus-treatment-acceleration-program-ctap ). On April 17, 2020, NIH announced a partnership with industry to expedite vaccine development ( https://www.nih.gov/news-events/news-releases/nih-launch-public-private-partnership-speed-covid-19-vaccine-treatment-options ). As of May 1, 2020, remdesivir (Gilead), granted FDA emergency use authorization, is the only approved therapeutic for COVID-19. 2
The pandemic has intensified research challenges. In a rush for data already thousands of manuscripts, news reports, and blogs have been published, but to date, there is limited scientifically robust data. Some studies do not meet published clinical trial standards, which now include FDA’s COVID-19-specific standards, 3 , 4 , 5 and/or are published without peer review. Misinformation from studies diverts resources from development and testing of more promising therapeutic candidates and has endangered lives. Ibuprofen, initially reported as unsafe for patients with COVID-19, resulted in a shortage of acetaminophen, endangering individuals for whom ibuprofen is contraindicated. Hydroxychloroquine initially reported as potentially effective for treatment of COVID-19 resulted in shortages for patients with autoimmune diseases. Remdesivir, in rigorous trials, showed decrease in duration of COVID-19, with greater effect given early. 6 Given the limited availability and safety data, the use outside clinical trials is currently approved only for severe disease. Vaccines typically take 10–15 years to develop. As of May 3, 2020, of nearly 100 vaccines in development, 8 are in trial. Several vaccines are projected to have emergency approval within 12–18 months, possibly as early as the end of the year, 7 still an eternity for this pandemic, yet too soon for long-term effectiveness and safety data. Antibody testing, necessary for diagnosis, therapeutics, and vaccine testing, has presented some of the greatest research challenges, including validation, timing, availability and prioritization of testing, interpretation of test results, and appropriate patient and societal actions based on results. 8 Relaxing physical distancing without data regarding test validity, duration, and strength of immunity to different strains of COVID-19 could have catastrophic results. Understanding population differences and disparities, which have been further exposed during this pandemic, is critical for response and long-term pandemic recovery. The “Equitable Data Collection and Disclosure on COVID-19 Act” calls for the CDC (Centers for Disease Control and Prevention) and other HHS (United States Department of Health & Human Services) agencies to publicly release racial and demographic information ( https://bass.house.gov/sites/bass.house.gov/files/Equitable%20Data%20Collection%20and%20Dislosure%20on%20COVID19%20Act_FINAL.pdf )
Trusted sources of up-to-date, easily accessible information must be identified (e.g., WHO https://www.who.int/emergencies/diseases/novel-coronavirus-2019/global-research-on-novel-coronavirus-2019-ncov , CDC https://www.cdc.gov/coronavirus/2019-nCoV/hcp/index.html , and for children AAP (American Academy of Pediatrics) https://www.aappublications.org/cc/covid-19 ) and should comment on quality of data and provide strategies and crisis standards to guide clinical practice.
Long-term, lessons learned from research during this pandemic could benefit the research enterprise worldwide beyond the pandemic and during other PHE/disasters with strategies for balancing multiple novel approaches and high-quality, time-efficient, cost-effective research. This challenge, at least in part, can be met by appropriate study design, collaboration, patient registries, automated data collection, artificial intelligence, data sharing, and ongoing consideration of appropriate regulatory approval processes. In addition, research to develop and evaluate innovative strategies and technologies to improve access to care, management of health and disease, and quality, safety, and cost effectiveness of care could revolutionize healthcare and healthcare systems. During PHE/disasters, crisis standards for research should be considered along with ongoing and just-in-time PHE/disaster training for researchers willing to share information that could be leveraged at time of crisis. A dedicated funded core workforce of PHE/disaster researchers and funded infrastructure should be considered, potentially as a consortium of networks, that includes physician-scientists, basic scientists, social scientists, mental health providers, global health experts, epidemiologists, public health experts, engineers, information technology experts, economists and educators to strategize, consult, review, monitor, interpret studies, guide appropriate clinical use of data, and inform decisions regarding effective use of resources for PHE/disaster research.
Differences between adult and pediatric COVID-19, the need for pediatric research
As reported by the CDC, from February 12 to April 2, 2020, of 149,760 cases of confirmed COVID-19 in the United States, 2572 (1.7%) were children aged <18 years, similar to published rates in China. 9 Severe illness has been rare. Of 749 children for whom hospitalization data is available, 147 (20%) required hospitalization (5.7% of total children), and 15 of 147 required ICU care (2.0%, 0.58% of total). Of the 95 children aged <1 year, 59 (62%) were hospitalized, and 5 (5.3%) required ICU admission. Among children there were three deaths. Despite children being relatively spared by COVID-19, spread of disease by children, and consequences for their health and pediatric healthcare are potentially profound with immediate and long-term impact on all of society.
We have long been aware of the importance and value of pediatric research on children, and society. COVID-19 is no exception and highlights the imperative need for a pediatrician-scientist workforce. Understanding differences in epidemiology, susceptibility, manifestations, and treatment of COVID-19 in children can provide insights into this pathogen, pathogen–host interactions, pathophysiology, and host response for the entire population. Pediatric clinical registries of COVID-infected, COVID-exposed children can provide data and specimens for immediate and long-term research. Of the 1133 COVID-19 studies on ClinicalTrials.gov, 202 include children aged ≤17 years. Sixty-one of the 681 interventional trials include children. With less diagnostic testing and less pediatric research, we not only endanger children, but also adults by not identifying infected children and limiting spread by children.
Pediatric considerations and challenges related to treatment and vaccine research for COVID-19 include appropriate dosing, pediatric formulation, and pediatric specific short- and long-term effectiveness and safety. Typically, initial clinical trials exclude children until safety has been established in adults. But with time of the essence, deferring pediatric research risks the health of children, particularly those with special needs. Considerations specific to pregnant women, fetuses, and neonates must also be addressed. Childhood mental health in this demographic, already struggling with a mental health pandemic prior to COVID-19, is now further challenged by social disruption, food and housing insecurity, loss of loved ones, isolation from friends and family, and exposure to an infodemic of pandemic-related information. Interestingly, at present mental health visits along with all visits to pediatric emergency departments across the United States are dramatically decreased. Understanding factors that mitigate and worsen psychiatric symptoms should be a focus of research, and ideally will result in strategies for prevention and management in the long term, including beyond this pandemic. Social well-being of children must also be studied. Experts note that the pandemic is a perfect storm for child maltreatment given that vulnerable families are now socially isolated, facing unemployment, and stressed, and that children are not under the watch of mandated reporters in schools, daycare, and primary care. 10 Many states have observed a decrease in child abuse reports and an increase in severity of emergency department abuse cases. In the short term and long term, it will be important to study the impact of access to care, missed care, and disrupted education during COVID-19 on physical and cognitive development.
Training and supporting pediatrician-scientists, such as through NIH physician-scientist research training and career development programs ( https://researchtraining.nih.gov/infographics/physician-scientist ) at all stages of career, as well as fostering research for fellows, residents, and medical students willing to dedicate their research career to, or at least understand implications of their research for, PHE/disasters is important for having an ongoing, as well as a just-in-time surge pediatric-focused PHE/disaster workforce. In addition to including pediatric experts in collaborations and consortiums with broader population focus, consideration should be given to pediatric-focused multi-institutional, academic, industry, and/or government consortiums with infrastructure and ongoing funding for virtual training programs, research teams, and multidisciplinary oversight.
The impact of the COVID-19 pandemic on research and research in response to the pandemic once again highlights the importance of research, challenges of research particularly during PHE/disasters, and opportunities and resources for making research more efficient and cost effective. New paradigms and models for research will hopefully emerge from this pandemic. The importance of building sustained PHE/disaster research infrastructure and a research workforce that includes training and funding for pediatrician-scientists and integrates the pediatrician research workforce into high-quality research across demographics, supports the pediatrician-scientist workforce and pipeline, and benefits society.
Johns Hopkins Coronavirus Resource Center. Covid-19 Case Tracker. Center for Systems Science and Engineering (CSSE) at Johns Hopkins University (JHU). https://coronavirus.jhu.edu/map.html (2020).
US Food and Drug Administration. Coronavirus (COVID-19) update: FDA issues emergency use authorization for potential COVID-19 treatment. FDA News Release . https://www.fda.gov/news-events/press-announcements/coronavirus-covid-19-update-fda-issues-emergency-use-authorization-potential-covid-19-treatment (2020).
Evans, S. R. Fundamentals of clinical trial design. J. Exp. Stroke Transl. Med. 3 , 19–27 (2010).
Article Google Scholar
Antman, E. M. & Bierer, B. E. Standards for clinical research: keeping pace with the technology of the future. Circulation 133 , 823–825 (2016).
Food and Drug Administration. FDA guidance on conduct of clinical trials of medical products during COVID-19 public health emergency. Guidance for Industry, Investigators and Institutional Review Boards . https://www.fda.gov/regulatory-information/search-fda-guidance-documents/fda-guidance-conduct-clinical-trials-medical-products-during-covid-19-public-health-emergency (2020).
National Institutes of Health. NIH clinical trials shows remdesivir accelerates recovery from advanced COVID-19. NIH New Releases . https://www.nih.gov/news-events/news-releases/nih-clinical-trial-shows-remdesivir-accelerates-recovery-advanced-covid-19#.XrIX75ZmQeQ.email (2020).
Radcliffe, S. Here’s exactly where we are with vaccines and treatments for COVID-19. Health News . https://www.healthline.com/health-news/heres-exactly-where-were-at-with-vaccines-and-treatments-for-covid-19 (2020).
Abbasi, J. The promise and peril of antibody testing for COVID-19. JAMA . https://doi.org/10.1001/jama.2020.6170 (2020).
CDC COVID-19 Response Team. Coronavirus disease 2019 in children—United States, February 12–April 2, 2020. Morb. Mortal Wkly Rep . 69 , 422–426 (2020).
Agarwal, N. Opinion: the coronavirus could cause a child abuse epidemic. The New York Times . https://www.nytimes.com/2020/04/07/opinion/coronavirus-child-abuse.html (2020).
Download references
Author information
Authors and affiliations.
Department of Pediatrics, Division of Emergency Medicine, Boston Children’s Hospital, Boston, MA, USA
Debra L. Weiner
Harvard Medical School, Boston, MA, USA
Department of Pediatrics, University of Wisconsin School of Medicine and Public Health, Madison, WI, USA
Vivek Balasubramaniam
Department of Pediatrics and Division of Neonatology, Maria Fareri Children’s Hospital at Westchester Medical Center, New York Medical College, Valhalla, NY, USA
Shetal I. Shah
Division of General Pediatrics, Children’s Hospital Los Angeles, Los Angeles, CA, USA
Joyce R. Javier
Keck School of Medicine, University of Southern California, Los Angeles, CA, USA
You can also search for this author in PubMed Google Scholar
Contributions
All authors made substantial contributions to conception and design, data acquisition and interpretation, drafting the manuscript, and providing critical revisions. All authors approve this final version of the manuscript.
Pediatric Policy Council
Scott C. Denne, MD, Chair, Pediatric Policy Council; Mona Patel, MD, Representative to the PPC from the Academic Pediatric Association; Jean L. Raphael, MD, MPH, Representative to the PPC from the Academic Pediatric Association; Jonathan Davis, MD, Representative to the PPC from the American Pediatric Society; DeWayne Pursley, MD, MPH, Representative to the PPC from the American Pediatric Society; Tina Cheng, MD, MPH, Representative to the PPC from the Association of Medical School Pediatric Department Chairs; Michael Artman, MD, Representative to the PPC from the Association of Medical School Pediatric Department Chairs; Shetal Shah, MD, Representative to the PPC from the Society for Pediatric Research; Joyce Javier, MD, MPH, MS, Representative to the PPC from the Society for Pediatric Research.
Corresponding author
Correspondence to Debra L. Weiner .
Ethics declarations
Competing interests.
The authors declare no competing interests.
Additional information
Publisher’s note Springer Nature remains neutral with regard to jurisdictional claims in published maps and institutional affiliations.
Members of the Pediatric Policy Council are listed below Author contributions.
Rights and permissions
Reprints and permissions
About this article
Cite this article.
Weiner, D.L., Balasubramaniam, V., Shah, S.I. et al. COVID-19 impact on research, lessons learned from COVID-19 research, implications for pediatric research. Pediatr Res 88 , 148–150 (2020). https://doi.org/10.1038/s41390-020-1006-3
Download citation
Received : 07 May 2020
Accepted : 21 May 2020
Published : 16 June 2020
Issue Date : August 2020
DOI : https://doi.org/10.1038/s41390-020-1006-3
Share this article
Anyone you share the following link with will be able to read this content:
Sorry, a shareable link is not currently available for this article.
Provided by the Springer Nature SharedIt content-sharing initiative
This article is cited by
Catalysing global surgery: a meta-research study on factors affecting surgical research collaborations with africa.
- Thomas O. Kirengo
- Hussein Dossajee
- Nchafatso G. Obonyo
Systematic Reviews (2024)
Lessons learnt while designing and conducting a longitudinal study from the first Italian COVID-19 pandemic wave up to 3 years
- Alvisa Palese
- Stefania Chiappinotto
- Carlo Tascini
Health Research Policy and Systems (2023)
Pediatric Research and COVID-19: the changed landscape
- E. J. Molloy
- C. B. Bearer
Pediatric Research (2022)
Cancer gene therapy 2020: highlights from a challenging year
- Georgios Giamas
- Teresa Gagliano
Cancer Gene Therapy (2022)
Quick links
- Explore articles by subject
- Guide to authors
- Editorial policies

- Open access
- Published: 22 May 2024
A scoping review of ethics review processes during public health emergencies in Africa
- Kingsley Orievulu 1 , 2 , 3 ,
- Alex Hinga 4 ,
- Busisiwe Nkosi 1 , 5 ,
- Nothando Ngwenya 1 , 2 ,
- Janet Seeley 1 , 2 , 6 ,
- Anthony Akanlu 7 ,
- Paulina Tindana 8 ,
- Sassy Molyneux 4 , 9 ,
- Samson Kinyanjui 4 , 9 &
- Dorcas Kamuya 4 , 9
BMC Medical Ethics volume 25 , Article number: 63 ( 2024 ) Cite this article
457 Accesses
2 Altmetric
Metrics details
The COVID-19 pandemic forced governments, multilateral public health organisations and research institutions to undertake research quickly to inform their responses to the pandemic. Most COVID-19-related studies required swift approval, creating ethical and practical challenges for regulatory authorities and researchers. In this paper, we examine the landscape of ethics review processes in Africa during public health emergencies (PHEs).
We searched four electronic databases (Web of Science, PUBMED, MEDLINE Complete, and CINAHL) to identify articles describing ethics review processes during public health emergencies and/or pandemics. We selected and reviewed those articles that were focused on Africa. We charted the data from the retrieved articles including the authors and year of publication, title, country and disease(s) reference, broad areas of (ethical) consideration, paper type, and approach.
Of an initial 4536 records retrieved, we screened the titles and abstracts of 1491 articles, and identified 72 articles for full review. Nine articles were selected for inclusion. Of these nine articles, five referenced West African countries including Liberia, Guinea and Sierra Leone, and experiences linked to the Ebola virus disease. Two articles focused on South Africa and Kenya, while the other two articles discussed more general experiences and pitfalls of ethics review during PHEs in Africa more broadly. We found no articles published on ethics review processes in Africa before the 2014 Ebola outbreak, and only a few before the COVID-19 outbreak. Although guidelines on protocol review and approval processes for PHEs were more frequently discussed after the 2014 Ebola outbreak, these did not focus on Africa specifically.
Conclusions
There is a gap in the literature about ethics review processes and preparedness within Africa during PHEs. This paper underscores the importance of these processes to inform practices that facilitate timely, context-relevant research that adequately recognises and reinforces human dignity within the quest to advance scientific knowledge about diseases. This is important to improve fast responses to PHEs, reduce mortality and morbidity, and enhance the quality of care before, during, and after pandemics.
Peer Review reports
The severe acute respiratory syndrome coronavirus 2 (SARS-CoV-2) accounted for over 624 million infections and over 6.5 million deaths globally in late 2022, and caused the coronavirus disease 2019 (COVID-19) pandemic [ 1 ]. The COVID-19 pandemic forced governments, multilateral public health organisations, humanitarian organisations, and academic institutions to undertake research urgently to understand SARS-CoV-2 and respond to the pandemic [ 2 , 3 , 4 , 5 , 6 , 7 ]. Research is still needed to generate knowledge on SARS-CoV-2 transmission, to develop vaccines and treatment, and to understand the long-term impacts of infection [ 3 , 8 ]. This research needs to explore the clinical, biomedical, social, and ethical aspects of COVID-19, including by recognising and incorporating local knowledge systems that are crucial for actualising the scientific and social value of this pursuit.
The conduct of research – especially during a public health emergency (PHE) where the research involves human participants – requires robust regulation against the backdrop of historical injustices and irresponsible practices, and what is often a well-intentioned ‘rush’ for solutions in public health interventions [ 9 , 10 , 11 , 12 ]. For these reasons, international ethical principles and guidelines are continuously developed, revised, and promoted to ensure that public health research is ethically sound, scientifically relevant, and robust and that human rights are upheld [ 5 , 7 , 10 , 13 , 14 , 15 ].
Africa has experienced different infectious disease outbreaks in the last decade, including Ebola virus disease (EVD), HIV/AIDS, yellow fever, Lassa fever, Rift Valley fever, and Mpox, all of which pose serious public health challenges on the continent. Similar to other globally recognised infectious diseases, such as Severe Acute Respiratory Syndrome (SARS) and Middle East Respiratory Syndrome (MERS), these infectious diseases have raised global attention towards research that aims to understand, treat and/or eradicate them [ 16 , 17 ]. Although humanitarian disasters, social and political instabilities and violent conflicts equally disrupt public health research and interventions, infectious disease outbreaks create even deeper shocks that can further cripple national, regional, and global economies and health systems.
Conducting research during PHEs (used hereafter to designate highly transmittable, infectious and deadly diseases officially designated as epidemics and pandemics) and natural disasters presents particular practical and ethical challenges. Within the context of pandemics such as COVID-19, the role of ethics review committees (ERCs) (used interchangeably in this paper with research ethics committees (RECs)/institutional review boards (IRBs) and national research ethics committees (NRECs)) – hereafter as RECs – is critical [ 5 , 7 , 13 , 18 ]. The urgency to understand COVID-19 prompted the development of a plethora of studies to quickly address the emergency, including observational, interventional, clinical, and human challenge studies.
This uncertain situation led to increased efforts to establish or strengthen research collaborations and partnerships as well as community engagement in and for research related purposes [ 19 ]. Considerations involved in establishing these partnerships and collaborations included funding priorities, decisions on which science or field of research and which sites or countries to fund. COVID-19 revived the equity concerns discussed within the decolonisation of global health literature and are still discussed and critiqued within and beyond social science [ 20 ]. The COVID-19 pandemic created another avenue for old systems of inequitable research practices to become entrenched, but also opened up platforms for debates about equity and equal partnerships in research, especially through prioritising and respecting local knowledge and contextual peculiarities [ 21 , 22 ].
While the scramble for solutions through research was important, so too are the processes of governing and overseeing the quality, rigour, and ethics of the proposed studies. Pandemic and epidemic contexts – like humanitarian disasters, sociopolitical instability, and violent conflict situations – cause complex and difficult dilemmas within the health system, and negatively impact efforts to establish and implement interventions. These complexities may be wide ranging in the quest for scientific breakthroughs. Maintaining the primacy of human rights and dignity in these situations can be challenging.
Documented ethical issues in conducting research during PHEs include preparing RECs for accelerated review of studies, for instance through the establishment of ad hoc committees [ 3 , 6 , 23 , 24 , 25 , 26 , 27 , 28 , 29 , 30 ]; ensuring appropriate research designs for scientific validity, social value and fair selection of participants [ 31 ]; promoting inclusive and adequate stakeholder engagement and informed consent processes [ 2 , 32 , 33 , 34 ]; dealing with the specific ethical conundrum of clinical trials and human challenge studies during emergencies [ 35 , 36 , 37 , 38 , 39 , 40 , 41 ]; supporting appropriate data collection, storage and future use, including those relating to children [ 33 , 42 , 43 , 44 , 45 ]; and maintaining mechanisms for ethics review whether in person or virtual [ 40 , 46 , 47 , 48 , 49 , 50 , 51 ]. However, few studies specifically consider ethics review processes, procedures and governance frameworks for epidemics and public health emergencies in Africa [ 52 ].
In this paper, we examine the landscape of ethics review processes in Africa during PHEs. Our objectives were to identify the context and content (where possible) of such processes and identify emerging issues. We also aimed to identify gaps in the literature on this topic in and for Africa.
We conducted a scoping review to explore existing literature reporting on ethical review processes and how they were structured, articulated, and managed in Africa within the context of PHEs. This is an important area in research given the challenges that PHEs could pose to ethics review processes supporting the timely conduct of scientifically rigorous and ethically sound research. We chose to undertake a scoping review because very little was documented about ethics review processes during PHEs in Africa. Scoping reviews are often exploratory [ 53 ], flexible and can combine sources based on both qualitative and quantitative analysis. Scoping reviews are descriptive and can be used to rapidly explore the field, identify research gaps [ 53 , 54 ].
Based on our interest in ethics review processes in Africa and their importance for effective, robust, and ethical governance of research during PHEs, this scoping review was important in assessing the mechanisms for ethics review in Africa, and in describing their processes for undertaking protocol reviews during PHEs. Arksey and O’Malley identify five stages of the methodological framework for scoping reviews: identifying the research question; identifying relevant studies; study selection; charting the data; and collating, summarising, and reporting the results [ 54 ]. We adapt this framework for our paper, outlining the research question(s), the search strategy, study screening and selection, data analysis, and findings.
Research question(s)
The broad question we address in this review is: What are the emerging ethical and practical issues within ethics review processes, frameworks, and procedures during PHEs? This is followed by two sub-questions, namely: What is the landscape of ethical review processes during PHEs? And how have ethical review processes been structured and executed during PHEs?
Search Strategy
We searched four electronic databases: Web of Science, PUBMED, MEDLINE Complete, and CINAHL (12 August − 12 September 2021, and 30 March 2023). Our main focus was on articles showing African experiences of PHEs. We followed Arksey and O’Malley’s scoping review guidance and framework, keeping the search terms flexible enough to accommodate as many articles as possible within the broader scope of the review [ 54 ]. We considered all materials available in these databases including unpublished work (like pre-prints), reports and commentaries.
We used the Preferred Reporting Items for Systematic Reviews and Meta-Analyses extension for Scoping Reviews (PRISMA-ScR) to guide the selection process [ 55 ]. Table 1 shows the search terms used, while Table 2 indicates our inclusion and exclusion criteria.
Study screening and selection
We imported all retrieved entries into Rayyan, an online systematic review management tool. KO and AH used Rayyan to automatically identify and exclude duplicates and conducted an initial screening by title, abstract, and full content review. This was followed by a second screening in which only articles from, reporting on, or referencing, the African context were included. Where there were disagreements, senior team members BN, NN, DK, and JS provided adjudication. We exported the data from Rayyan into Microsoft Excel and classified them by their Rayyan identification numbers, article title, author name, year of publication, country reference, disease reference, broad topic covered, paper type, and approach. These were initially selected for the second phase of the review and screened using the inclusion criteria set out in Table 2 . The actual studies included in the study were largely peer-reviewed publications, reflection/discussion papers and essays – all of which are allowed within the context of a scoping review [ 53 , 54 ].
Data analysis
In line with Arksey and O’Malley [ 54 ] and Peters et al. [ 53 ], we conducted a descriptive thematic analysis. The broad thematic areas covered included the country or regional context within which ethics review processes were identified; the description of ethics review processes and regulatory frameworks identified or mentioned in the articles; and the considerations identified by RECs during review processes, ranging from study design to informed consent, collaborative partnerships, and engagement. These themes were drawn from our review objectives and emphasised based on the level of attention afforded them by the papers reviewed.
We located 4536 potential papers from our initial search. After removing 3045 duplicates, they were reduced to 1491, which we screened. A further 1207 were excluded after reviewing titles and abstracts for relevance to the review question(s). We then conducted two rounds of full-text review of the remaining 284 articles, first excluding 212 articles that did not meet our criteria, and then excluding 63 of the remaining 72 because they were not about Africa. Nine articles were included in the final review. Figure 1 shows the PRISMA flowchart.
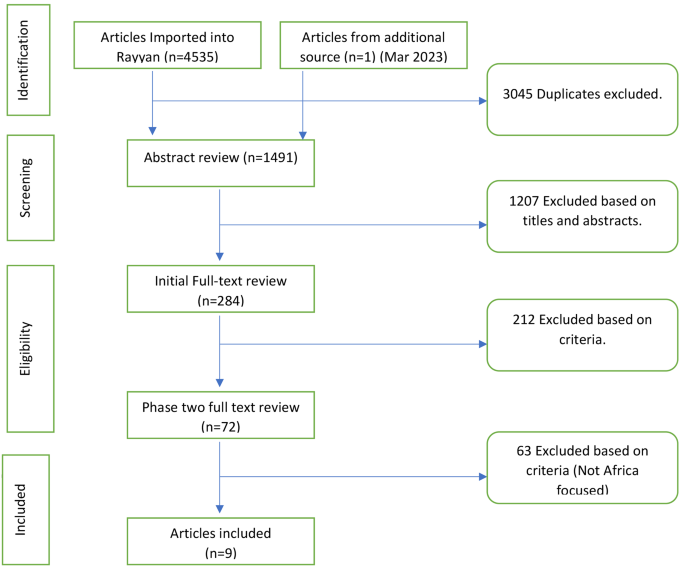
PRISMA Flowchart of Study selection
As shown in Table 3 , most (6/9) of the included articles are discussion papers published in peer-reviewed journals [ 23 , 56 , 57 , 58 , 59 , 61 ]. Two original research articles were included: a qualitative methods paper [ 62 ] and a mixed method paper with a strong qualitative element [ 52 ]. Six articles covered issues related to ethics review and EVD considerations [ 23 , 56 , 57 , 59 , 60 , 61 ]; three covered issues linked to COVID-19, including (informed) consent [ 58 ], stakeholder engagement [ 62 ] and research and ethics review [ 52 ]; three commented on virtual modalities for review (meetings), consultations or obtaining consent [ 23 , 52 , 58 ]; and five described review timelines during public health disasters [ 52 , 57 , 59 , 60 , 61 ].
Most articles were drawn from or referenced West African countries including Liberia, Guinea, and Sierra Leone, either individually ( [ 57 , 61 ]) or as a collective [ 23 , 56 , 59 ]. These were the countries most affected by EVD between 2014 and 2016. One article drew on South African experience [ 58 ] and another on Kenyan experience [ 52 ] during the COVID-19 pandemic. The remaining two articles reference Africa broadly [ 62 ] or extrapolated from an African experience to make comments about ethics review processes during PHEs across low- and middle-income countries [ 60 ].
Thematic areas in the African ethics review process landscape
In this section, we describe two broad thematic areas emerging from the scoping review: A) The processes, procedures and frameworks established or drawn upon by RECs to facilitate timely and robust reviews under PHEs. Process-related sub-themes describe the preparatory and challenging components of these REC processes. B) The considerations that were highlighted or flagged by RECs or the articles pertaining to research being conceptualised, planned for, or conducted in, the context of PHEs. The sub-themes described under these considerations emphasise ideas around the nature of proposed studies, including important ethical and practical concerns within PHE-related research.
A) Processes, procedures and frameworks for ethics review during PHE
Preparing for (accelerated and robust) ethical reviews during outbreaks.
The reviewed articles describe different aspects of processes, procedures, and frameworks set up or implemented during PHEs. Five studies describe experiences of establishing or reviewing clinical trial(s) or intervention studies and some the procedural aspects of ethics review [ 52 , 56 , 57 , 59 , 61 ]. Three articles emphasise preparing review bodies [ 23 , 52 ] or their members in LMICs [ 60 ] for reviewing study proposals during outbreaks through skills audit and training. Saxena et al. [ 23 ] discuss preparing committees as a priority outcome of the 2018 workshop organised between the World Health Organisation Global Health Ethics Team and the African coalition for Epidemic Research, Response and Training (ALERRT) [ 23 ]. The articles describe the need for accelerating review processes, audits to identify and address competency gaps among REC members through training, and approaches to review studies in the event of future (and possibly deadly) infectious disease outbreaks [ 23 , 52 , 60 ].
Regulatory and procedural issues for accelerated reviews
The reviewed articles show some of the important steps undertaken to facilitate accelerated reviews. These include the World Health Organisation Ethics Research Committee (WHO-ERC) development of and reliance on Standard Operating Procedures (SOP) – or Rules of Procedures – for accelerated review during emergency periods [ 23 , 56 ]; establishing the protocol review subcommittee; and ensuing training for specialised subject-area reviews. Alirol and colleagues [ 56 ] highlighted the importance of the WHO-ERC Rules of Procedure, noting that “the EVD outbreak was the first-time accelerated review was implemented” [ 56 ]. These rules provided the framework for sensitising WHO-ERC members on the plans by the WHO to rapidly review Ebola disease-related studies. The established WHO-ERC subcommittee was populated by volunteers recruited on short notice. The newly established system designed to accelerate reviews and protocol assessment was characterised by regular monthly meetings (face to face or teleconferences) and task allocations [ 56 ].
Saxena et al. highlighted another priority issue for preparedness: pre-review of generic (non-context specific) research protocols by RECs [ 23 ]. The generic protocols were to be developed outside of a period of infectious disease outbreaks, facilitating applications for review early during an outbreak. These protocols are easier to review by REC and can be adapted, thus increasing speed without compromising quality [ 23 ]. Reflecting on their experiences of submitting research protocols for review during the COVID-19 pandemic, researchers in Kenya supported the idea of pre-reviewing generic protocols in non-emergency times to accelerate research review and implementation during public health emergencies [ 52 ].
Membership composition of review committees
Within the EVD context, Alirol and colleagues [ 56 ] highlight that setting up the review of intervention and preventative studies, especially clinical trials of therapeutic products, required the WHO-ERC to draw on guidelines of the Council for International Organisations of Medical Sciences (CIOMS) concerning conducting research with human participants, especially during disasters and public health emergencies [ 56 ]. This WHO-ERC was constituted as a 27-member committee consisting of experts “in clinical research, drug development, social sciences, [and] legal affairs”, including “a lay member” [ 56 ]. “Between 6 and 8 members are from Geneva-based universities or international organisations” [ 56 ]. Schopper and colleagues [ 59 ] also highlight that the Medecins Sans Frontieres Ethics Review Board (MSF-ERB) contributed and worked with other institutions towards the design and review of (intervention) study protocols, including clinical trials. This was in line with the International Health Regulation’s (IHR) declaration about EVD and the WHO permission for the ethical use of unregistered interventions in the treatment of Ebola patients [ 59 ].
Multiple review processes
Six articles describe processes for undertaking double or multiple ethics reviews within collaborative/partnership research which is jointly funded [ 23 , 52 , 57 , 59 , 60 , 61 ]. For example, the Ebola-Tx clinical trials study funded by a European Union grant was sponsored by the Institute of Tropical Medicine (ITM) and implemented at the MSF Ebola Treatment Centres (ETC) in Guinea [ 57 ]. De-Crop et al. [ 57 ] highlight that in this study, the initial process involved forming a research consortium comprising 17 institutions led by the ITM. Consequently, the study protocol had to go to multiple RECs from the study country (Guinea) and the sponsor country (Belgium) and to the institutional committees of collaborating institutions such as ITM, MSF, WHO, and LSHTM. Although other collaborators did not demand the submission of the protocol for assessment, the scientific Commission of the National Ebola Coordination in Guinea considered the scientific rationale of the study before issuing an initial approval for the study in Guinea [ 57 ]. The requirements for these review processes included the protocol (to be reviewed by coordinators from Belgium and Guinea), informed consent documents, a no-fault insurance certificate, CVs of the scientific coordinator and country PI, and case report forms [ 57 ]. Although much of the documentation required was similar, there were some differences in the content and modalities of submission across institutions, essentially resulting in multiple ethics review process, with implications for the timing of the trial.
Similarly, the Partnership for Research on Ebola Vaccines in Liberia ( PREVAIL ) was a collaboration between the USA and the Liberian government to establish and implement clinical trials under the EVD context in Liberia [ 61 ]. Reviews were therefore required from the different country bodies of the funders and country where the trial was implemented. Doe-Anderson and colleagues [ 61 ] highlighted that the protocol was submitted to two regulatory authorities in each partner country: the Federal Drug Authority and the IRB of the National Cancer Institute (NCI) at the National Institutes of Health (NIH) in the United States and the Liberian Medicines and Health Products Regulatory Authority (LMHRA) and National Research Ethics Board (NREB) [ 61 ].
Schopper et al. also report elements of multiple reviews for studies reviewed by the MSF-ERB and other institutions, including Oxford University and London School of Hygiene and Tropical Medicine [ 59 ]. They note: “Of the 27 protocols reviewed by the MSF-ERB, 11 were in addition reviewed by a national EC only, while 7 were reviewed by a national EC and one or several ECs/IRBs from other international institutions or academic centres” [ 59 ](p.52–54).
The multiple review processes undertaken for PHE studies are often intended to support partners, funders and institutions involved in collaborations to achieve the rapid but robust protocol reviews before their implementation [ 23 ].
Bottlenecks around multiple reviews
Five articles highlight drawbacks associated with multiple reviews, especially within a drive to achieve expeditious and scientifically robust reviews during outbreaks [ 23 , 52 , 57 , 59 , 60 ]. Protocol submission requests, specific REC comments, revisions, and resubmissions – requiring researchers’ replies to specific REC queries – often impacted timelines for the review process and study implementation [ 57 , 59 , 60 ]. The varying capacities and processes of different review committees, in terms of review turnaround times, for example, were highlighted as a major challenge for multiple reviews since researchers had to respond to reviewers’ comments on different versions of submitted protocols [ 52 ]. Although multiple review processes aim to ensure robust ethical standards and quality review, these did not insulate the process from possible debilitating complexities, hence the emphasis on the adoption of joint reviews and coordination to harmonise the process and circumvent some of these inherent complexities [ 23 , 59 , 60 ].
Guidance documents for outbreak reviews
Studies also reference different international and national guidelines, proposals, consultation outcomes, recommendations, and frameworks, broadly governing the design and implementation of the studies. This includes guidance on ethics review processes and procedures for the studies being reviewed, whether clinical trials, experimental studies, intervention studies or other types of studies. For example, the Ebola-Tx trial processes relied on the 2014 WHO “consultation on vaccines and therapies” [ 57 ]. This consultation resulted in a consensus on the imperative for the rapid development of study protocols for effectively testing vaccines and therapies that demonstrate promise to be used for interventions. To facilitate trust in this process, multiple institutional reviews and harmonising such processes across the different ethics committees were viewed as crucial [ 57 ].
De Vries and colleagues draw on national (South African) and international guidelines to discuss important questions and applications of informed consent while designing, reviewing and implementing COVID-19-related studies and interventions [ 58 ]. Folayan and colleagues focused on elements of the “Good Participatory Practices for Emergency Pathogens” (GPP-EP) to discuss the centrality of stakeholder engagement in designing and implementing COVID-19 clinical trials in SSA [ 62 ]. Hinga et al. highlighted the development of institutional and national-level guidelines for review of research protocols during the COVID-19 pandemic in Kenya, which included guidelines for protecting participants and research staff from infection during data collection and guidance on remote submission and review of protocols [ 52 ]. The fundamental role of, and reliance on, ethical and practical documentation guidelines is arguably considered vital to the efficiency and transparency of ethics review processes. Adapting these to unique outbreak circumstances and contexts is a major aspect of the review process.
Review timelines
Timelines for the review and approval of protocols varied within the different reviewed articles. In the case of the WHO-ERC, an average of six working days was reported for the WHO-ERC teams to review protocols submitted under the context of the EVD [ 56 ]. This was different from the reviews conducted by the MSF-ERB, which reported over 30 days between initial request and final approval [ 59 ]. In the latter, there was an initial average response time of 12.4 days from initial submission to replies from the investigator. Although this timing reduced to 1–4 days, the influx of more protocols increased the MSF-ERB workload [ 59 ]. In Kenya, the review of research protocols during the COVID-19 pandemic was faster than during the pre-pandemic period. However, internally set targets for review turnaround times during the pandemic were generally not met; there was a 5-day delay by the national review committee in providing initial feedback on new research protocols [ 52 ]. The PREVAIL study took less than 30 days to obtain all required approvals after intentional strides to address the concerns raised around conducting vaccine trials on people [ 61 ], and the Ebola Tx Trial study took 55 days [ 57 ]. Bain and colleagues critiqued the conventional system of ethics reviews, which took between 24 and 44 days, as counterproductive to the goal of gaining an understanding of new infectious diseases [ 60 ].
B) Considerations identified during review processes
Appropriateness of the proposed study design.
Five articles discuss the appropriateness of different study designs in the context of PHEs [ 56 , 57 , 59 , 60 , 61 ]. The study design was reviewed in relation to the need for scientific validity, social value, and minimising risk while maximising benefits during PHEs and infectious disease outbreaks [ 56 , 60 ].
Randomisation in clinical trials and experimental intervention studies for EVD was highlighted as particularly challenging, with RECs proposing a change of design for all participants to receive the experimental intervention treatment [ 56 , 57 , 59 , 61 ]. For the COVID-19 pandemic, RECs highlighted the need to account for loss to follow-up while calculating study power given the significant disruption and uncertainty [ 52 ]. The PREVAIL Vaccine trial considered both a RCT and ring design but chose the RCT design because it provided “… the greatest likelihood of providing more definitive results, and could potentially lead to rapid licensure and availability of effective vaccines” [ 61 ]. The MSF-ERB determined a priori to use the ring design as community engagement consultations (through MSF) revealed that randomisation was unacceptable, as it represented a “lottery system” for receiving the intervention (in the clinical trial) despite high Ebola mortality in the community [ 57 ]. For the WHO-ERC, protocols that provided strong arguments for the benefit-risk ratio assumption were accepted if they came from the Ebola-affected countries [ 56 ].
Formative research to inform the development of protocols and appropriate research designs during PHEs.
Linked to ensuring the appropriateness of research design to context, four papers noted the importance of formative research during PHEs [ 59 , 60 , 61 , 62 ]. Formative research allows consideration of crucial and sensitive components of the social contexts, cultural norms and practices and potential misgivings, fears and sensitivities to be considered in the design and conduct of clinical trials [ 60 , 62 ].The feasibility of undertaking formative research was raised, linked to safety as well as time for approvals [ 59 ]. Nevertheless, Bain and colleagues [ 60 ] emphasised the importance of formative research during emergencies, especially to inform randomised trials. They promote the use of rapid anthropological research methods during disasters as warranting the attention of RECs, the response team, and researchers.
Study population and involving vulnerable populations in research and intervention.
The study population within protocols under review during PHEs was discussed in four articles [ 56 , 57 , 59 , 61 ]. RECs definitions of risk and benefits, and how consent should be obtained, influenced their different views regarding the inclusion or exclusion of vulnerable populations [ 57 , 59 ]. One REC suggested that researchers should provide alternative methods of participation for individuals without smartphones to prevent unfair exclusion of participants during the COVID-19 pandemic [ 52 ]. The PREVAIL study excluded pregnant women, lactating mothers, and children because of the inadequacy of safety data [ 61 ]. The WHO and MSF RECs however emphasised the importance of including pregnant women, children and unaccompanied minors unless their exclusion was justified based on data demonstrating greater risk than standard of care [ 56 , 59 ]. Although neither REC rejected protocols that excluded these vulnerable populations, these examples highlight essential ethical study design considerations during PHEs.
Addressing informed consent in study protocols
Informed consent was referenced in most of the articles reviewed [ 56 , 57 , 58 , 59 , 60 , 62 ], with discussions on delayed, proxy and waived consent [ 56 , 58 ]. The WHO-ERC waived consent for two protocols aimed at retrieving anonymised information from patient records [ 56 ].
Some papers emphasised the need to guard against scenarios of situational coercion in participants’ recruitment, including scenarios where a third party – husbands, parents, or local chiefs – may influence an individual’s participation [ 56 , 62 ]. Contexts of deadly outbreaks such as the EVD can facilitate scenarios where people are tacitly coerced to participate in a study without adequate information and informed consent [ 56 ]. The WHO-ERC thus emphasised ensuring that information documents are simplified in the language of participants and well explained [ 56 ]. In the Ebola-Tx study, the REC required the researchers to provide clarity around considerations of consent related to minors and unaccompanied minors [ 57 ]. The consent of parents in this context (for minors) and other third party actors, such as local chiefs, is designed to protect potential participants but can lead to coerced consent in communities and families [ 62 ]. The training and capacitation of researchers, implementers, and REC members were therefore emphasised [ 60 ], and the involvement of the community in developing informed consent documents recommended [ 56 , 58 , 62 ].
Prioritising stakeholder engagement
Four articles referenced the importance of stakeholder engagement, consultation, and involvement in the process of designing and implementing research studies [ 58 , 60 , 61 , 62 ]. Stakeholder engagement is a component of the “Good Participatory Practices for Emergency Pathogens (GPP-EP)” [ 62 ]. De Vries and colleagues [ 58 ] reflected on the importance of community engagement using new and conventional media (including social media, TV, radio, and newspapers) to facilitate information sharing and communicate findings, especially where in-person contact was difficult. The authors recommended that ethics review processes impress on researchers to ensure that “community and public engagement [are] genuine and robust, long term and include a plan for post-pandemic communication of research results and plans for long term sample and data storage” [ 58 ].
The PREVAIL study used the concept of social mobilisation and communication (SMC) to emphasise multistakeholder engagement in the planning, recruitment for, and implementation of clinical trials [ 61 ]. This aimed to better understand local perceptions of and attitudes towards the EVD against the backdrop of views that EVD was man-made, externally curated, and transmitted to populations through clinical trials [ 61 ]. Indeed, deep-seated myths, mistrust, and suspicion about interventions, the government and the disease(s) require not only education but also advocacy and consultations to manage (and subsequently implement) research without widespread disapprovals from local populations [ 58 , 59 , 60 , 61 , 62 ]. Study protocols therefore had to reflect strategies for stakeholder engagement, and RECs had to emphasise and request that study designers – PIs – and sponsors address these issues during review processes [ 58 , 60 ].
Demonstrating collaborative partnerships within study protocols
Three articles showed the need for protocols submitted for review during PHEs to demonstrate equitable collaboration and partnerships between external and local researchers [ 56 , 60 , 61 ]. Collaborating in research during PHE has important implications for ethics review including the need for proposed studies to truly reflect the local context of research and interventions. However, a number of factors can contribute to a lack of collaboration between local and external researchers including marginalisation of local actors and researchers [ 60 ]. Thus, the WHO-ERC requested protocols to clarify the involvement of (and nature of collaboration with) local scientists and local actors for better contextual analysis and engagement with communities and people within the context of EVD research and interventions [ 56 ]. The emphasis by the WHO-ERC emanates from documented reports of heightened levels of mistrust within some African countries about externally funded research linked to infectious diseases outbreaks [ 59 , 60 , 61 ]. Such mistrust is traced to historical injustices and experiences such as polio-related side effects in Nigeria and other issues such as local attitudes towards blood and linked misgivings about the collection and storage of people’s blood samples [ 59 , 60 , 61 ].
This is relevant considering the critique of the poorly established nature of collaborative frameworks and partnerships between researchers and institutions from donor and local contexts, especially as regards the management and recognition of ethics approvals [ 60 ]. While double or multiple, but harmonised, ethics review of multiple site study protocols is generally acceptable [ 56 , 57 , 59 , 60 ], situations of unequal collaborative activities elongate the review process. This makes it largely difficult for timely review because the externally imposed institutional guidelines and processes lack adequate relevance in the context of research implementation, leading to missed research opportunities [ 60 ].
Data/Sample sharing and future use
Data and sample collection, storage and sharing remain very sensitive issues in the context of research and interventions during PHEs. These often challenge the concept of equitable partnerships in research collaborations between institutions in the Global North and those in Africa, and accounts for the attention that research review processes pay to them. In this review, these issues were predominantly referenced by three articles [ 23 , 58 , 59 ]. Studies found it unethical to impose a blanket ban on data and sample sharing but proposed that critical ethical questions must be raised. It was proposed that RECs could establish modalities that can be used to review protocols in the area of data sharing. On the one hand, this would entail the requesting research sponsors and PIs to submit preliminary data and sampling sharing plans on how data generated will be shared. On the other hand, these applicants can be requested to submit full-data sharing plans when resubmitting their (now full) application [ 23 ].
Accordingly, de Vries et al. argue that RECs must establish guidelines that clearly define the types of research data that can be used in the context of imperfect informed consent – bearing in mind that there is a high likelihood of such situations during disease outbreaks [ 58 ]. While debates remain about how ethical (or not) it is to use samples collected during the COVID-19 pandemic for broad population genomic studies or to interrogate questions completely unrelated to the condition [ 58 ] (p. 638), the studies highlighted the importance of RECs to guide the modalities for data sharing and use through engagements with PIs and study sponsors within the process of reviewing proposals during pandemics. The process would ensure that researchers clearly outline plans and justifications for storage, sharing and future use of data and/or samples in their ethics clearance application [ 56 , 58 ]. It would also ensure a clear description of whether samples and data will be stored and shared, who they will be shared with (with or without restrictions), and what they will be used for in the future [ 56 , 58 ].
In the case of blood samples,, Schopper and colleagues contend that the ethics review process must be used to ensure that protocols explicitly indicate if blood samples collected during the research study will be destroyed or stored for future use [ 59 ], and that this information must be clear in information sheets and consent forms and the patients/participants must explicitly indicate their choice/decision. For example, in all the studies that they reviewed, the WHO-ERC explicitly requested for clarifications on sample and data ownership, data sharing policy, processes for decisions on future use of samples and appropriate participant information [ 56 ]. Nevertheless, in view of the urgency of the EVD, the WHO-ERC approved studies where researchers demonstrated commitment to put appropriate agreements/processes in place [ 56 ].
The articles reviewed provided several recommendations pertaining to ensuring preparedness, efficiency and effectiveness of ethics review processes. Central among these was the need for the harmonisation of review processes through mechanisms such as joint review committees [ 57 ] – with representatives of individual ethics committees [ 56 ] – in cases of double, multiple and/or multi-site reviews [ 23 , 56 , 59 ]. This mechanism would enhance direct dialogue between the different ethics committees and reduce duplication or contradictory reviews [ 23 , 56 , 57 , 59 ]. Capacity building, upskilling, and training for REC members, partner institutions, and local investigators was also recommended; while the need to emphasise or institute the use of generic protocols that could be considered in the event of emergencies was buttressed to reduce time spent on long arduous processes [ 23 , 56 , 59 ]. Finally, authors of the papers reviewed recommended the need to simplify ethics review guidelines, clarify and agree on terminologies used in generic protocols [ 23 , 60 ], flexibility with REC processes, and the need for governments and donors to provide adequate resources which would allow for more anthropological research processes [ 59 ].
This review explored the landscape of ethics review processes, procedures, and frameworks in Africa. We identified that within the African context, there is a gap in the published literature on research ethics review processes during PHEs, including protocol review processes, preparedness, and priority-setting. While the Ebola virus disease provided the basis for more active engagement with the issue of oversight and governance for ethics review processes, little has been published on ethics review processes, and frameworks during PHEs within the African landscape. This is an important area of interest because there are studies, commentaries and opinion pieces on intervention or response strategies and activities in the event of PHEs such as the recent COVID-19 pandemic or disasters in general; and for research to be undertaken, it must first be approved by a REC.
Nuanced reliance of RECs on international guidelines for research during PHEs
We found that the ethics review processes relied on, and referenced, existing international ethical guidelines and principles for managing research, including during PHEs [ 5 , 14 , 15 ]. Most of the reviewed articles demonstrated that the processes undertaken, established, or adopted within the context of the PHEs within which they emerged, were rooted in these international principles relevant to the conduct of pandemic research. This is in line with different global studies where emphasis has been placed on international guidelines and guiding principles on ethical conduct of research processes [ 16 , 25 , 63 , 64 ].
Bain and colleagues, however, critiqued the pervasiveness of the existing international guidelines on the basis of the contextual appropriateness of some of these guidelines [ 60 ]. This is especially important in terms of their potential negative impacts on timely and swift but robust and context-sensitive ethics review processes for study protocols during PHEs or disasters. They note that without quick turnaround review times, much could be lost with regards to the knowledge that could be gained from data collected at crucial – not redundant – contexts of the infectious disease outbreak. These issues point to what many commentaries perceive as a top-down approach to pandemic preparedness that have potential ramifications for ethics review processes. One might describe this as a hegemonic dependence on international guidelines that are not locally developed or truly reflective of local content and context. Such outlooks echo aspects of decolonisation literature that lay emphasis on privileging internationally imposed systems, including those of knowledge production, within strides to prepare for and manage research processes during PHEs in Africa [ 20 , 21 , 22 , 65 ].
However, while these international frameworks and guidelines provided the impetus and inspiration for many of the structures laid out within these processes during PHEs, they were not the only sources of guidance. Due to the importance of context-specificity, different country and REC contexts, the REC membership had to rely on their uniqueness to develop appropriate processes (or adapt existing ones) and guidelines to address their needs. For example, despite the importance of the Good Clinical Practice to South Africa, the country has national principles and legislations guiding the conduct of research involving human subject which are adapted to particular situations such as the COVID-19 context (these include the National Health Act No. 61 of 2003; the National Department of Health Guidelines on Ethics in Health Research, and South Africa Health Products Regulatory Authority (SAPHRA) South Africa Good Clinical Practice.) Thus, while international guidelines remained quite central to the mechanisation of ethics review processes and frameworks within Africa, reliance on them has been nuanced, and they have been – are being or have to be – applied contextually.
Similarity of process(es) for PHEs ethical research governance
Additionally, the studies reviewed share similarities in terms of processes set in motion or recommended to be put in place to facilitate accelerated review in current PHEs or in preparation for future experiences. The need for rapid, expedited ethics review of study protocols to facilitate a speedy investigation into disease pathogenesis as well as therapeutics and interventions required to curb the spread and impacts of the diseases was a cross-cutting theme in the papers reviewed [ 60 ]. A central idea here entailed constituting ex-temporal committees or processes of review, including joint and multiple reviews [ 23 , 56 ] to reduce the propensity for long and duplicated reviews [ 3 , 4 ]. Such a framework, which allows for representatives of individual RECs to form part of the ad hoc committee, allows for a central review and approval process for which the final decision will be reported back via the same channel, thus reducing the time for the review of articles.
This process contributes to crucial recommendations, such as the development or amendment of the SOP pertaining to the conduct of research, and considerations of the context-specificity of PHEs. Other recommendations include enhancing flexibility for how ethics review processes work, the manner of coordination and harmonisation required in cases of multisite studies, joint reviews, and collaborative activities to strengthen the review processes, robustness, and speed of reviews [ 3 , 24 , 48 ]. The idea of establishing ad hoc committees is not universally endorsed, as some contend that ad hoc committee processes unnecessarily impede the desired expedition of reviews and defeats the purpose for which it was proposed or recommended [ 30 ]. Indeed, steps undertaken to expedite the review process do not often yield the intended result – a point confirmed by De Crop and colleagues’ experience of the Ebola Tx Trial study [ 57 ].
Relevance of the content of REC reviews for ethical principles during PHEs research
Interrogating the content of the ethics review processes, we also found that issues such as study design, the nature of informed consent – especially how information is communicated, and consent obtained – and the importance of stakeholder engagement and collaborative partnerships were high on the agenda of most of the Africa-specific literature reviewed. This is in line with the requirements emphasised within COVID-19 institutional research contexts and perspectives, and beyond. A COVID-19 research review intervention in New Jersey (USA) ensured that proposals that lacked clear research questions, methodology and research designs were rejected (internally); they were therefore not allowed to proceed for REC approval [ 66 ]. At the core of this emphasis is the need for study protocols, and research review processes to strengthen, not minimise, important ethical principles – from respect for persons, the imperative to do no harm, maximise benefit and minimise risks under beneficence, to demonstrating fairness and justice particularly in the selection of study participants – during infectious disease outbreaks [ 66 , 67 , 68 ]. These findings are also captured in the broader literature on the implementation of research during pandemics and disasters [ 47 , 69 , 70 ], as well as the ethical considerations that researchers and sponsors must bear in mind in their design and implementation of studies that involve human subjects [ 4 , 43 , 65 ], especially those from vulnerable communities and populations [ 70 ].
This emphasis reiterates the push against prioritising the pursuit of scientific knowledge above respect for human rights, dignity, and autonomy, especially within the frame of respecting participants and their autonomy, the processes of seeking consent, and the information contained in the consent forms during PHEs [ 7 , 9 , 14 , 67 ]. With regards to informed and voluntary consent and participation, the role of ethics review processes to institutionalise human rights and dignity is revealed within the contents of REC recommendations and areas of emphasis in the articles in our review. This aligns with the dominant literature and debates about what consent is and how it should be obtained, how much information should be shared about interventions (proven and unproven), risks and benefits, and who can give consent during PHEs research [ 5 , 15 , 16 , 63 , 67 , 71 ]. These study designs, methodological and other ethical considerations serve to strengthen the research process against the reoccurrence of any likeness of the historical injustices such as the experiments by the Nazi physicians, the Thalidomide case study, and the Tuskegee Syphilis study [ 9 , 67 ]. They echo the view that COVID-19 “should not be viewed as an opening to opportunistically reduce participant protection” [ 67 ] (p. 9).
In the context of PHE, many study participants and communities could be vulnerable, desperate to participate in any research that offers some hope of therapy and hence the potential for exploitation can be heightened [ 7 , 71 ]. Our reviewed articles emphasised ensuring that communities/societies facing PHEs adequately understand the rationale for the research being undertaken in their context and why it is important [ 59 , 60 , 62 ]. Respect for communities and participants is especially critical at this time; literature on previous PHEs or disasters can be drawn on to inform how to ensure fairness at every stages of the research and/or research intervention activities [ 2 , 7 , 19 , 60 ]. For clinical trials, Folayan and colleagues proposed a comprehensive stakeholder engagement with interest groups who can positively or negatively impact how the proposed study or intervention would work, and emphasize the need for formative research to help identify these interest groups [ 62 ].
Social science considerations: interdisciplinary composition of RECs during PHEs
Our findings show the critical role of formative research in facilitating the ethical conduct of research, including informing research design and contextually appropriate informed consent processes, as well as ways to strengthen rigour of the research and acceptable consent processes [ 59 , 60 ]. Learning from previous experiences, there is now a marked emphasis, including within multilateral humanitarian institutions such as the WHO, in adequately incorporating social science into biomedical research [ 71 ].
In addition, insights from social scientists are important within the REC review processes, especially during PHEs, given the socio-behavioural aspects of the spread and containment of infectious diseases. As some commentators and contexts showed within the COVID-19 research context where community engagement and support for research was needed [ 67 , 68 ], flexibility within RECs mechanisms is crucial, such as bigger, inclusive, interdisciplinary, and collaborative review teams [ 67 ]. This is important because of the drive to achieve a balance between a biomedical research focus, the promotion of ethical principles, and support for good health outcomes through the skills, competence and reach that members, especially social scientists, bring to the review of research during PHEs and beyond [ 43 , 72 , 73 ]. Social science research approaches contribute within research designs, as independent studies, enhancing contextual understandings of the people, participants, or targets of research (and interventions) [ 60 , 65 ]. This is more crucial within environments riddled with mistrust of health practitioners and researchers in particular, as well as strong cultural beliefs and interpretations of and engagement with medical practices and modalities from the Global North [ 59 , 60 , 71 , 74 , 75 , 76 ].
Study limitations
An important limitation of this scoping review is that our search strategy was aimed to identify African experiences however, findings were largely limited to certain regions due to the available literature during our search timeframe. Also, while the diversity of focus within the articles reviewed demonstrates different components of the ethics review processes and frameworks required for ethical conduct of research during PHEs, many of the arguments made in this review are inferred. Essentially, we argued that authors’ emphasis on certain components of the ethical conduct of research implies a recommendation for what RECs should consider in their engagement with research protocols during the context of a PHE. Hence, a reading of some of the articles reviewed will point to discussions, for example, about the importance of stakeholder engagement and various aspects of informed consent application within the establishment and execution of studies during COVID-19 [ 58 , 62 ]. While the components remain crucial for ethics review processes, they were not discussed in-depth by the authors in that sense, hence our inferential conclusions.
This scoping review reveals that much more needs to be done around ethics review processes and procedures within Africa for better preparedness and response to emerging and future pandemics. This is needed so that timely context-relevant research can be undertaken but also in a manner that adequately recognises and reinforces the dignity of people in the quest to gain more understanding of diseases. This requires training and capacity building for REC members, reviewing the make-up and competencies of RECs to handle specific cases during PHEs, and for governments and study funders to make funds more flexible and durable to allow for training and more ingenious approaches to research review during public health emergencies.
Furthermore, drawing from the findings of this review, especially those around the dearth of literature on ethics review processes within Africa during PHEs, we recommend more documentation of the experiences linked to planning and implementation of ethics review processes. Donors can facilitate this by supporting the development of reflections on these experiences to be documented and duly published. Also, aligning with Bain and colleagues [ 61 ], deepening mechanisms that would ensure continued and stronger social science contributions not only within community engagement components but in the review processes would prove crucial for strengthening the review processes during PHEs.
Data availability
All the data generated or analysed during this scoping review have been included in this manuscript.
World Health Organization. COVID-19 weekly epidemiological update, edition 115, 26 October 2022. 2022.
Xafis V, Schaefer GO, Labude MK, Zhu YJ, Hsu L. The Perfect Moral Storm: diverse ethical considerations in the COVID-19 pandemic. Asian Bioeth Rev. 2020;12(2):65–83.
Article Google Scholar
Aarons D. Research in epidemic and emergency situations: a model for collaboration and expediting ethics review in two Caribbean countries. Dev World Bioeth. 2018;18(4):375–84.
Aarons D. Addressing the challenge for expedient ethical review of research in disasters and disease outbreaks. Bioethics. 2019;33(3):343–6.
World Health Organization. Ethical standards for research during public health emergencies: distilling existing guidance to support COVID-19 R&D. Geneva: WHO; 2020.
Google Scholar
Tamariz L, Hendler F, Wells J, Anderson A, Bartlett S. A call for Better, not faster, Research Ethics Committee Reviews in the Covid-19 era. Ethics Hum Res. 2021;43(5):42–4.
Nuffield Council on Bioethics. Research in global health emergencies: ethical issues. London: Nuffield Council on Bioethics; 2020.
Ohaeri C, Thomas DR, Salmon J, Cottrell S, Lyons J, Akbari A et al. Comparative risk of cerebral venous sinus thrombosis (CVST) following COVID-19 vaccination or infection: a national cohort study using linked electronic health records. Hum Vaccines Immunotherapeutics. 2022:2127572.
Rice TW. The historicthical, and legal background of human-subjects research. Respir Care. 2008;53(10).
Kapumba BM, Desmond N, Seeley J. A chronological discourse analysis of ancillary care provision in guidance documents for research conduct in the global south. BMC Med Ethics. 2022;23(1):51.
Burgess T, Rennie S, Moodley K. Key ethical issues encountered during COVID-19 research: a thematic analysis of perspectives from South African research ethics committees. BMC Med Ethics. 2023;24(1):11.
Voit K, Skuban-Eiseler T, Orzechowski M, Steger F. Informed consent in COVID-19-Research: an ethical analysis of clinical studies performed during the pandemic. 2023;11(12):1793.
Nuffield Councilon Bioethics. Fair and equitable access to COVID-19 treatments and vaccines. Nuffield Council on Bioethics London; 2020.
Council for International Organizations of Medical Sciences (CIOMS). International ethical guidelines for health-related research involving humans. Fourth Edition ed. Geneva: CIOMS. 2016. 122 p.
World Health Organization. Guidance for managing ethical issues in infectious disease outbreaks. Geneva: WHO; 2016. p. 66.
Ma X, Wang Y, Gao T, He Q, He Y, Yue R, et al. Challenges and strategies to research ethics in conducting COVID-19 research. J evidence-based Med. 2020;13(2):173–7.
Neumann G, Kawaoka Y. Which Virus Will Cause Next Pandemic? Viruses. 2023;15(1):199.
Hummel P, Adam T, Reis A, Littler K. Taking stock of the availability and functions of National Ethics Committees worldwide. BMC Med Ethics. 2021;22(1):56.
Tindana P, De Vries J, Kamuya D. Ethical challenges in community engagement practices in research during the COVID-19 pandemic in Africa [version 1; peer review: 1 approved, 1 approved with reservations]. 2020;3(23).
Fofana MO. Decolonising global health in the time of COVID-19. Glob Public Health. 2021;16(8–9):1155–66.
Ndlovu-Gatsheni SJ. Geopolitics of power and knowledge in the COVID-19 pandemic: decolonial reflections on a Global Crisis. 2020;36(4):366–89.
Willows TM, Oliwa J, Onyango O, Mkumbo E, Maiba J, Schell CO et al. COVID-19 and unintended steps towards further equity in global health research. BMJ Global Health. 2023;8(6).
Saxena A, Horby P, Amuasi J, Aagaard N, Kohler J, Gooshki ES et al. Ethics preparedness: facilitating ethics review during outbreaks - recommendations from an expert panel. BMC Med Ethics. 2019;20.
Kieny M-P, Rägo L. Regulatory policy for research and development of vaccines for public health emergencies. Expert Rev Vaccines. 2016;15(9):1075–7.
Ijkema R, Janssens M, van der Post JAM, Licht CM. Ethical review of COVID-19 research in the Netherlands; a mixed-method evaluation among medical research ethics committees and investigators. PLoS ONE. 2021;16(7).
Hunt M, Tansey CM, Anderson J, Boulanger RF, Eckenwiler L, Pringle J, et al. The challenge of Timely, Responsive and Rigorous Ethics Review of Disaster Research: views of Research Ethics Committee members. PLoS ONE. 2016;11(6):e0157142.
Eckenwiler L, Pringle J, Boulanger R, Hunt M. Real-time responsiveness for Ethics Oversight during Disaster Research. Bioethics. 2015;29(9):653–61.
Packenham JP, Rosselli RT, Ramsey SK, Taylor HA, Fothergill A, Slutsman J, et al. Conducting Science in disasters: recommendations from the NIEHS Working Group for Special IRB Considerations in the review of Disaster Related Research. Environ Health Perspect. 2017;125(9):094503.
Packenham JP, Rosselli R, Fothergill A, Slutsman J, Ramsey S, Hall JE, et al. Institutional Review Board Preparedness for Disaster Research: a practical Approach. Curr Environ Health Rep. 2021;8(2):127–37.
Sisa I, Mena B, Teran E. The negative impact of ad hoc committees for ethical evaluation: the case of COVID-19-related research in Ecuador. Dev World Bioeth. 2021;21(1):3–6.
Lynch H, Lundin D, Meagher E. Ethical Inclusion of Health Care Workers in Covid-19 Research. Ethics Hum Res. 2021;43(2):19–27.
Beach MC, Lederman HM, Singleton M, Brower RG, Carrese J, Ford DE, et al. Desperate Times: protecting the public from Research without Consent or Oversight during Public Health emergencies. Ann Intern Med. 2020;173(11):926–8.
AHC-MEDIA. COVID-19 pandemic changed informed consent for Biobanking: New Common Rule exception used. IRB Advisor. 2020;20(11):1–2.
van der Graaf R, Hoogerwerf M, de Vries M. The ethics of deferred consent in times of pandemics. Nat Med. 2020;26(9):1328–30.
Jamrozik E, Selgelid MJ. Human infection challenge studies in endemic settings and/or low-income and middle-income countries: key points of ethical consensus and controversy. J Med Ethics. 2020;46(9):601–9.
Jamrozik E, Selgelid M. COVID-19 human challenge studies: ethical issues. Lancet Infect Dis. 2020;20(8):e198–203.
Jamrozik E, Littler K, Bull S, Emerson C, Kang G, eep, et al. Key criteria for the ethical acceptability of COVID-19 human challenge studies: report of a WHO Working Group. Vaccine. 2021;39(4):633–40.
Hsu NS, Hendriks S, Ramos KM, Grady C. Ethical considerations of COVID-19-related adjustments to clinical research. Nat Med. 2021;27(2):191–3.
Hashem H, Abufaraj M, Tbakhi A, Sultan I. Obstacles and considerations related to clinical Trial Research during the COVID-19 pandemic. Front Med. 2020;7.
Dal-Ré R, Bekker L, Gluud C, Holm S, Jha V, Pol, et al. Ongoing and future COVID-19 vaccine clinical trials: challenges and opportunities. The Lancet Infectious diseases; 2021.
Richards AD. Ethical guidelines for deliberately infecting volunteers with COVID-19. J Med Ethics. 2020;46(8):502–4.
Beauvais M, Knoppers B. Coming out to play: privacy, Data Protection, Children’s Health, and COVID-19 research. Front Genet. 2021;12:659027.
Lapid MI, Meagher KM, Giunta HC, Clarke BL, Ouellette Y, Armbrust TL, et al. Ethical challenges in COVID-19 Biospecimen Research: perspectives from Institutional Review Board members and bioethicists. Mayo Clin Proc. 2021;96(1):165–73.
Nicol D, Chalmers D, Critchley C, Eckstein L, Nielsen J, Otlowski M. Australian perspectives on the Ethical and Regulatory Considerations for Responsible Data Sharing in response to the COVID-19 pandemic. J Law Med. 2020;27(4):829–38.
Singh S, Cadigan R, Moodley K. Challenges to biobanking in LMICs during COVID-19: time to reconceptualise research ethics guidance for pandemics and public health emergencies? J Med Ethics. 2021.
Agrawal V, Nath C, ishwar, Mishra SK. Ethics committee meeting by video-conferencing during Covid-19. Indian J Med Ethics. 2020:1–2.
Aung MN, Murray V, Kayano R. Research Methods and Ethics in Health Emergency and Disaster Risk Management: the result of the Kobe Expert Meeting. Int J Environ Res Public Health. 2019;16(5).
Bavdekar S. Ethics committees: actions during pandemic and lockdown situations. J Postgrad Med. 2020;66(3):119–22.
De Vos E, Ethical PPE. Overseeing Research in the time of COVID-19. Narrative Inq Bioeth. 2021;11(1):69–70.
Faust A, Sierawska A, Krüger K, Wisgalla A, Hasford J, Strech D. Challenges and proposed solutions in making clinical research on COVID-19 ethical: a status quo analysis across German research ethics committees. BMC Med Ethics. 2021;22(1):96.
Yagi K, Maeda K, Sakaguchi S, Chuma M, Sato Y, Kane C, et al. Status of Institutional Review Board meetings conducted through web Conference systems in Japanese National University hospitals during the COVID-19 pandemic: Questionnaire Study. J Med Internet Res. 2020;22(11):e22302.
Hinga A, Jeena L, Awuor E, Kahindi J, Munene M, Kinyanjui S, et al. Pandemic preparedness and responsiveness of research review committees: lessons from review of COVID-19 protocols at KEMRI Wellcome Trust Research Programme in Kenya. Wellcome open Res. 2022;7:75.
Peters MDJ, Marnie C, Tricco AC, Pollock D, Munn Z, Alexander L, et al. Updated methodological guidance for the conduct of scoping reviews. JBI Evid Synthesis. 2020;18(10):2119–26.
Arksey H, O’Malley L. Scoping studies: towards a methodological framework. Int J Soc Res Methodol. 2005;8(1):19–32.
PRISMA Extension for Scoping Reviews (PRISMA-ScR). Checklist and Explanation. Ann Intern Med. 2018;169(7):467–73.
Alirol E, Kuesel A, Guraiib M, de la Fuente-Núñez V, Saxena A, Gomes M. Ethics review of studies during public health emergencies - the experience of the WHO ethics review committee during the Ebola virus disease epidemic. BMC Med Ethics. 2017;18(1):43.
De Crop M, Delamou A, re, Griensven JV, Ravinetto R. Multiple ethical review in North-South collaborative research: the experience of the Ebola-Tx trial in Guinea. Indian J Med Ethics. 2016;1(2):76–82.
de Vries J, Burgess T, Blockman M, Ntusi NAB. Research on COVID-19 in South Africa: guiding principles for informed consent. South Afr Med J. 2020;110(7):635–9.
Schopper D, Ravinetto R, Schwartz L, Kamaara E, Sheel S, Segelid MJ, et al. Research Ethics Governance in Times of Ebola. Public Health Ethics. 2017;10(1):49–61.
Bain LE, Ngwain CG, Nwobegahay J, Sumboh JG, Nditanchou R, Awah PK. Research Ethics Committees (RECs) and epidemic response in low and middle income countries. Pan Afr Med J. 2018;31.
Doe-Anderson J, Baseler B, Driscoll P, Johnson M, Lys J et al. Beating the odds: Successful establishment of a Phase II/III clinical research trial in resource-poor Liberia during the largest-ever Ebola outbreak. Contemporary Clinical Trials Communications. 2016;4:68–73.
Folayan MO, Brown B, Haire B, Babalola CP, Ndembi N. Considerations for stakeholder engagement andCOVID-19 related clinical trials’ conduct in sub-saharan Africa. Dev World Bioeth. 2021;21(1):44–50.
Emanuel EJ, Wendler D, Killen J, Grady C. What makes clinical research in developing countries ethical? The benchmarks of ethical research. J Infect Dis. 2004;189(5):930–7.
Mezinska S, Kakuk P, Mijaljica G, Waligóra M, O’Mathúna DP. Research in disaster settings: a systematic qualitative review of ethical guidelines. BMC Med Ethics. 2016;17:62.
Watson S. Don’t cross the line, you’re a researcher and not an educator’: incorporating indigenous researchers’ moral perspectives to improve ethical protocols in health research. Soc Sci Med. 2022;315:115503.
Paleoudis EG, Jacobs LG, Friedman T, Fittizzi C, Sawczuk I, Aschner J. Implementing a review process to facilitate and prioritize COVID-19 research: staying one step ahead of the pandemic. J Empir Res Hum Res Ethics. 2021;16(3):188–92.
Fernandez Lynch H, Dickert NW, Zettler PJ, Joffe S, Largent EA. Regulatory flexibility for COVID-19 research. J law Biosci. 2020;7(1):lsaa057.
Sisk BA, DuBois J. Research Ethics during a pandemic: a call for normative and empirical analysis. Am J Bioeth. 2020;20(7):82–4.
Barron Ausbrooks CY, Barrett EJ, Martinez-Cosio M. Ethical issues in Disaster Research: lessons from Hurricane Katrina. Popul Res Policy Rev. 2009;28(1):93–106.
Falb K, Laird B, Ratnayake R, Rodrigues K, Annan J. The ethical contours of research in crisis settings: five practical considerations for academic institutional review boards and researchers. Disasters. 2019;43(4):711–26.
Schopper D, Upshur R, Matthys F, Singh JA, Bandewar SS, Ahmad A, et al. Research Ethics Review in Humanitarian contexts: the experience of the Independent Ethics Review Board of Médecins Sans Frontières. PLoS Med. 2009;6(7):e1000115.
Bavel JJV, Baicker K, Boggio PS, Capraro V, Cichocka A, Cikara M, et al. Using social and behavioural science to support COVID-19 pandemic response. Nat Hum Behav. 2020;4(5):460–71.
WHO Technical Advisory Group on Behavioral Insights and Sciences for Health. Behavioural and social sciences are critical for pandemic prevention, preparedness and response: WHO; 2022 [updated 23 February 2022. https://www.who.int/news-room/commentaries/detail/behavioural-and-social-sciences-are-critical-for-pandemic-prevention-preparedness-and-response .
Geissler PW, Kelly A, Imoukhuede B, Pool R, editors. Substantial transactions and an ethics of kinship in recent collaborative malaria vaccine trials in the Gambia. New York: Oxford: Berghahn Books; 2012.
Geissler PW, Kelly A, Imoukhuede B, Pool R. He is now like a brother, I can even give him some blood’ – relational ethics and material exchanges in a malaria vaccine ‘trial community’ in the Gambia. Soc Sci Med. 2008;67(5):696–707.
Sulemane N, Armocida B, Valente M, Formenti B, Barigazzi S, Ussai S, et al. Vaccines hesitancy in Africa: how COVID-19 pandemic may affect malaria vaccination campaigns. J Prev Med Hyg. 2022;63(1):E1–3.
Download references
Acknowledgements
The authors are grateful to the social science core team at the Africa Health Research Institute for their support in the development of this piece of research. We thank Manono Luthuli, Zakhele Xulu, Dumisile Mthethwa, Anita Edwards and Khethokuhle Nkosi for their intensive contribution to the planning and execution of the Ethics in COVID-19 study that birthed this paper.
The research described in this paper was funded by the African Academy of Science research grant (AAS-CRD01). The AHRI research was funded in whole or in part by the Wellcome Trust (Wellcome Strategic Core award: grant number 201433/Z/16/A). The KEMRI-Wellcome Trust research was funded in whole or part by the Wellcome Trust core grant (203077/Z/16/Z). For the purpose of open access, the authors have applied a CC BY public copyright licence to any author-accepted manuscript version arising from this submission. The funders did not play any role in the design of the study; the collection, analysis, and interpretation of data; or the writing of this manuscript.
Author information
Authors and affiliations.
Africa Health Research Institute, KwaZulu-Natal, Durban, South Africa
Kingsley Orievulu, Busisiwe Nkosi, Nothando Ngwenya & Janet Seeley
School of Nursing and Public Health, University of KwaZulu-Natal, Durban, South Africa
Kingsley Orievulu, Nothando Ngwenya & Janet Seeley
Centre for Africa China Studies, University of Johannesburg, Johannesburg, South Africa
Kingsley Orievulu
KEMRI Wellcome Trust Research Programme, Kilifi, Kenya
Alex Hinga, Sassy Molyneux, Samson Kinyanjui & Dorcas Kamuya
University of Toledo, Ohio, Toledo, USA
Busisiwe Nkosi
Global Health and Development Department, London School of Hygiene and Tropical Medicine, London, UK
Janet Seeley
West Africa Centre for Cell Biology and Infectious Pathogens, University of Ghana, Accra, Ghana
Anthony Akanlu
Department of Health Policy, Planning and Management, School of Public Health, College of Health Sciences, University of Ghana, Accra, Ghana
Paulina Tindana
Centre for Tropical Medicine and Pandemic Sciences Institute, Nuffield Department of Medicine, University of Oxford, Oxford, UK
Sassy Molyneux, Samson Kinyanjui & Dorcas Kamuya
You can also search for this author in PubMed Google Scholar
Contributions
KO, AH and DK conceptualised and designed the review. KO and AH coordinated the data search process. KO and AH contributed to the analysis of the results and drafted the manuscript. BN, NN, PT, AA, SM, SK, JS and DK critically reviewed the manuscript. All authors contributed to the revision of the manuscript and approved the final manuscript.
Corresponding author
Correspondence to Kingsley Orievulu .
Ethics declarations
Ethics approval and consent to participate.
Not applicable.
Consent for publication
Competing interests.
The authors declare no competing interests.
Additional information
Publisher’s note.
Springer Nature remains neutral with regard to jurisdictional claims in published maps and institutional affiliations.
Rights and permissions
Open Access This article is licensed under a Creative Commons Attribution 4.0 International License, which permits use, sharing, adaptation, distribution and reproduction in any medium or format, as long as you give appropriate credit to the original author(s) and the source, provide a link to the Creative Commons licence, and indicate if changes were made. The images or other third party material in this article are included in the article’s Creative Commons licence, unless indicated otherwise in a credit line to the material. If material is not included in the article’s Creative Commons licence and your intended use is not permitted by statutory regulation or exceeds the permitted use, you will need to obtain permission directly from the copyright holder. To view a copy of this licence, visit http://creativecommons.org/licenses/by/4.0/ . The Creative Commons Public Domain Dedication waiver ( http://creativecommons.org/publicdomain/zero/1.0/ ) applies to the data made available in this article, unless otherwise stated in a credit line to the data.
Reprints and permissions
About this article
Cite this article.
Orievulu, K., Hinga, A., Nkosi, B. et al. A scoping review of ethics review processes during public health emergencies in Africa. BMC Med Ethics 25 , 63 (2024). https://doi.org/10.1186/s12910-024-01054-8
Download citation
Received : 09 October 2023
Accepted : 06 May 2024
Published : 22 May 2024
DOI : https://doi.org/10.1186/s12910-024-01054-8
Share this article
Anyone you share the following link with will be able to read this content:
Sorry, a shareable link is not currently available for this article.
Provided by the Springer Nature SharedIt content-sharing initiative
- Ebola virus disease
- Ethics review
- Pandemic research
- Public health emergency
- Research ethics committee
- Scoping review
- Sub-saharan Africa
BMC Medical Ethics
ISSN: 1472-6939
- General enquiries: [email protected]
- Open access
- Published: 14 May 2024
Rain, rain, go away, come again another day: do climate variations enhance the spread of COVID-19?
- Masha Menhat 1 ,
- Effi Helmy Ariffin ORCID: orcid.org/0000-0002-8534-0113 2 ,
- Wan Shiao Dong 3 ,
- Junainah Zakaria 2 ,
- Aminah Ismailluddin 3 ,
- Hayrol Azril Mohamed Shafril 4 ,
- Mahazan Muhammad 5 ,
- Ahmad Rosli Othman 6 ,
- Thavamaran Kanesan 7 ,
- Suzana Pil Ramli 8 ,
- Mohd Fadzil Akhir 2 &
- Amila Sandaruwan Ratnayake 9
Globalization and Health volume 20 , Article number: 43 ( 2024 ) Cite this article
506 Accesses
3 Altmetric
Metrics details
The spread of infectious diseases was further promoted due to busy cities, increased travel, and climate change, which led to outbreaks, epidemics, and even pandemics. The world experienced the severity of the 125 nm virus called the coronavirus disease 2019 (COVID-19), a pandemic declared by the World Health Organization (WHO) in 2019. Many investigations revealed a strong correlation between humidity and temperature relative to the kinetics of the virus’s spread into the hosts. This study aimed to solve the riddle of the correlation between environmental factors and COVID-19 by applying RepOrting standards for Systematic Evidence Syntheses (ROSES) with the designed research question. Five temperature and humidity-related themes were deduced via the review processes, namely 1) The link between solar activity and pandemic outbreaks, 2) Regional area, 3) Climate and weather, 4) Relationship between temperature and humidity, and 5) the Governmental disinfection actions and guidelines. A significant relationship between solar activities and pandemic outbreaks was reported throughout the review of past studies. The grand solar minima (1450-1830) and solar minima (1975-2020) coincided with the global pandemic. Meanwhile, the cooler, lower humidity, and low wind movement environment reported higher severity of cases. Moreover, COVID-19 confirmed cases and death cases were higher in countries located within the Northern Hemisphere. The Blackbox of COVID-19 was revealed through the work conducted in this paper that the virus thrives in cooler and low-humidity environments, with emphasis on potential treatments and government measures relative to temperature and humidity.
• The coronavirus disease 2019 (COIVD-19) is spreading faster in low temperatures and humid area.
• Weather and climate serve as environmental drivers in propagating COVID-19.
• Solar radiation influences the spreading of COVID-19.
• The correlation between weather and population as the factor in spreading of COVID-19.
Graphical abstract
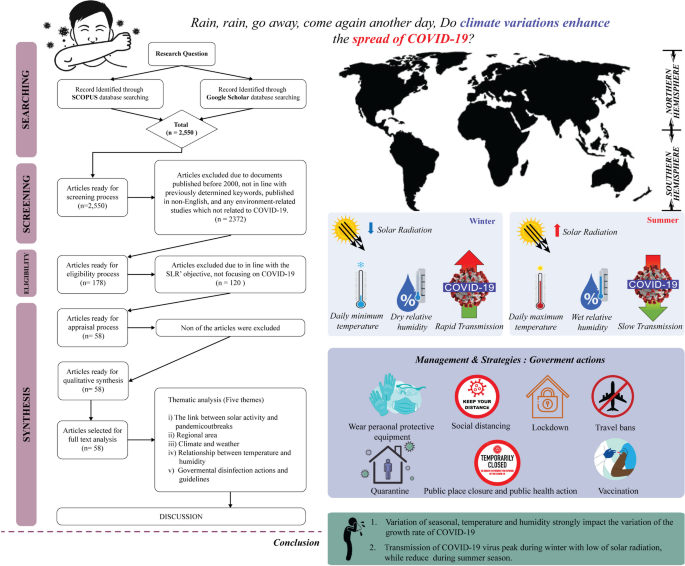
Introduction
The revolution and rotation of the Earth and the Sun supply heat and create differential heating on earth. The movements and the 23.5° inclination of the Earth [ 1 ] separate the oblate-ellipsoid-shaped earth into northern and southern hemispheres. Consequently, the division results in various climatic zones at different latitudes and dissimilar local temperatures (see Fig. 1 ) and affects the seasons and length of a day and night in a particular region [ 2 ]. Global differential heating and climate variability occur due to varying solar radiation received by each region [ 3 ]. According to Trenberth and Fasullo [ 4 ] and Hauschild et al. [ 5 ] the new perspective on the issue of climate change can be affected relative to the changes in solar radiation patterns. Since the study by Trenberth and Fasullo [ 4 ] focused on climate model changes from 1950 to 2100, it was found that the role of changing clouds and trapped sunlight can lead to an opening of the aperture for solar radiation.
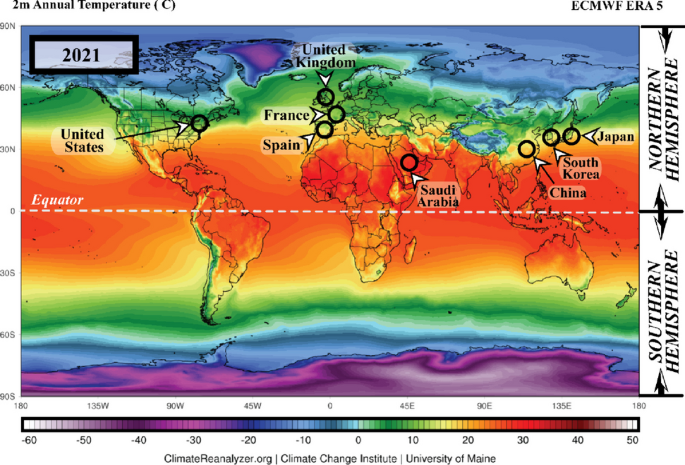
The annual average temperature data for 2021 in the northern and southern hemispheres ( Source: meteoblue.com ). Note: The black circles mark countries with high Coronavirus disease 2019 (COVID-19) infections
Furthermore, the heat from sunlight is essential to humans; several organisms could not survive without it. Conversely, the spread of any disease-carrying virus tends to increase with less sunlight exposure [ 6 ]. Historically, disease outbreaks that led to epidemic and pandemic eruptions were correlated to atmospheric changes. Pandemic diseases, such as the flu (1918), Asian flu (1956–1958), Hong Kong flu (1968), and recently, the coronavirus disease 2019 (COVID-19) (2019), recorded over a million death toll each during the winter season or minimum temperature conditions [ 7 ]. The total number of COVID-19 cases is illustrated in Fig. 2 .
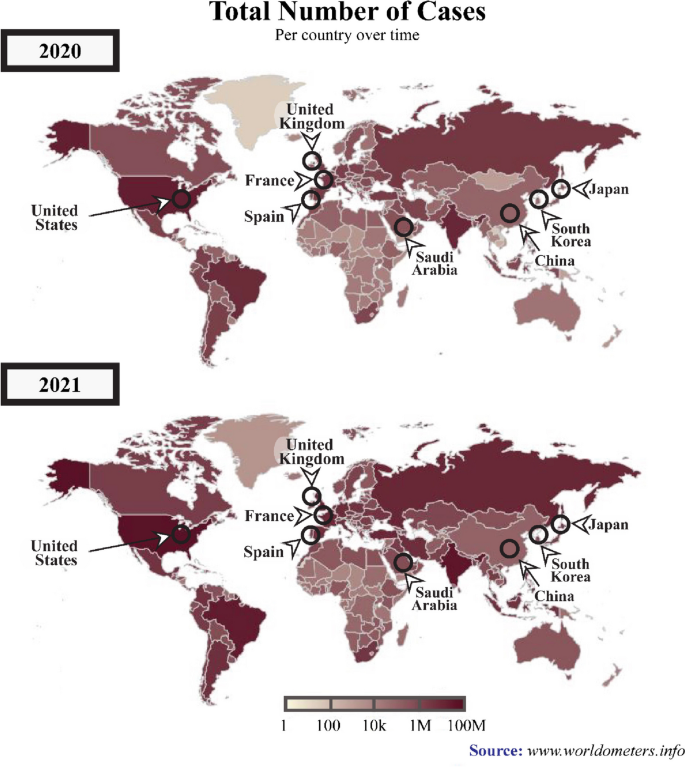
A graphical representation of the total number of COVID-19 cases across various periods between 2020 and 2021. ( Source : www.worldometers.info ). Note: The black circles indicate countries with high numbers COVID-19-infections
In several previous outbreaks, investigations revealed a significant association between temperature and humidity with a particular focus on the transmission dynamics of the infection from the virus into the hosts [ 8 , 9 , 10 ]. Moreover, disease outbreaks tended to heighten in cold temperatures and low humidity [ 11 ]. Optimal temperature and sufficient relative humidity during evaporation are necessary for cloud formation, resulting in the precipitated liquid falling to the ground as rain, snow, or hail due to the activity of solar radiation balancing [ 4 ].
Consequently, the radiation balancing processes in the atmosphere are directly linked to the living beings on the earth, including plants and animals, and as well as viruses and bacterias. According to Carvalho et al. [ 12 ]‘s study, the survival rate of the Coronaviridae Family can decrease during summer seasons. Nevertheless, numerous diseases were also developed from specific viruses, such as influenza, malaria, and rubella, and in November 2019, a severe health threat originated from a 125 nm size of coronavirus, had resulted in numerous deaths worldwide.
Transmission and symptoms of COVID-19
The COVID-19, or severe acute respiratory syndrome coronavirus 2 (SARS-CoV-2), is an infectious disease caused by a newly discovered pathogenic virus from the coronavirus family, the novel coronavirus (2019-nCoV) [ 13 ]. The first case was recorded in Wuhan, China, in December 2019 [ 14 ]. The pathogenic virus is transmitted among humans when they breathe in air contaminated with droplets and tiny airborne particles containing the virus [ 14 , 15 , 16 , 17 , 18 ].
According to the World Health Organization (WHO), the most common symptoms of COVID-19 infection include fever, dry cough, and tiredness. Nevertheless, older people and individuals with underlying health problems (lung and heart problems, high blood pressure, diabetes, or cancer) are at higher risk of becoming seriously ill and developing difficulty breathing [ 19 ]. The COVID-19 was initially only predominant in China but rapidly spread to other countries globally. The remarkably swift acceleration of the number of infections and mortality forced WHO to declare COVID-19 a global public health emergency on the 30th of January 2020, which was later declared as a pandemic on the 11th of March 2020 [ 20 ].
Since no vaccine was available then, WHO introduced the COVID-19 preventative measures to reduce the chances of virus transmission. The guideline for individual preventative included practising hand and respiratory hygiene by regularly cleaning hands with soap and water or alcohol-based sanitisers, wear a facemask and always maintaining at least a one-meter physical distance [ 21 ]. Nevertheless, the worldwide transmission of COVID-19 has resulted in fear and forced numerous countries to impose restrictions rules, such as lockdown, travel bans, closed country borders, restrictions on shipping activities, and movement limitations, to diminish the spread of COVID-19 [ 22 ].
According to WHO, by the 2nd of December 2020, 63,379,338 confirmed cases and 1,476,676 mortalities were recorded globally. On the 3rd of December 2021, 263,655,612 confirmed cases and deaths were recorded, reflecting increased COVID-19 infections compared to the previous year. The American and European regions documented the highest COVID-19 patients with 97,341,769 and 88,248,591 cases, respectively (see Fig. 2 ), followed by Southeast Asia with 44,607,287, Eastern Mediterranean accounted 16,822,791, Western Pacific recorded 6,322,034, and Africa reported the lowest number of cases at 6,322,034 [ 19 ].
Recently, an increasing number of studies are investigating the association between environmental factors (temperature and humidity) and the viability, transmission, and survival of the coronavirus [ 23 , 24 , 25 , 26 ]. The results primarily demonstrated that temperature was more significantly associated with the transmission of COVID-19 [ 27 , 28 , 29 ] and its survival period on the surfaces of objects [ 30 ]. Consequently, the disease was predominant in countries with low temperature and humidity [ 31 ], which was also proven by Diao et al. [ 32 ]‘s study demonstrating higher rates of COVID-19 transmission in China, England, Germany, and Japan.
A comprehensive systematic literature review (SLR) is still lacking despite numerous research on environmental factors linked to coronavirus. Accordingly, this article aimed to fill the gap in understanding and identifying the correlation between environmental factors and COVID-19 by analysing existing reports. Systematically reviewing existing literature is essential to contribute to the body of knowledge and provide beneficial information for public health policymakers.
Methodology
The present study reviewed the protocols, formulation of research questions, selection of studies, appraisal of quality, and data abstraction and analysis.
The protocol review
The present SLR was performed according to the reporting standards for systematic evidence syntheses (ROSES) and followed or adapted the guidelines as closely as possible. Thus, in this study, a systematic literature review was guided by the ROSES review protocol (Fig. 3 ). Compared to preferred reporting items for systematic review and meta-analysis (PRISMA), ROSES is a review protocol specifically designed for a systematic review in the conservation or environment management fields [ 33 ]. Compared to PRISMA, ROSES offers several advantages, as it is tailored to environmental systematic review, which reduces emphasis on quantitative synthesis (e.g. meta-analysis etc.) that is only reliable when used with appropriate data [ 34 ].
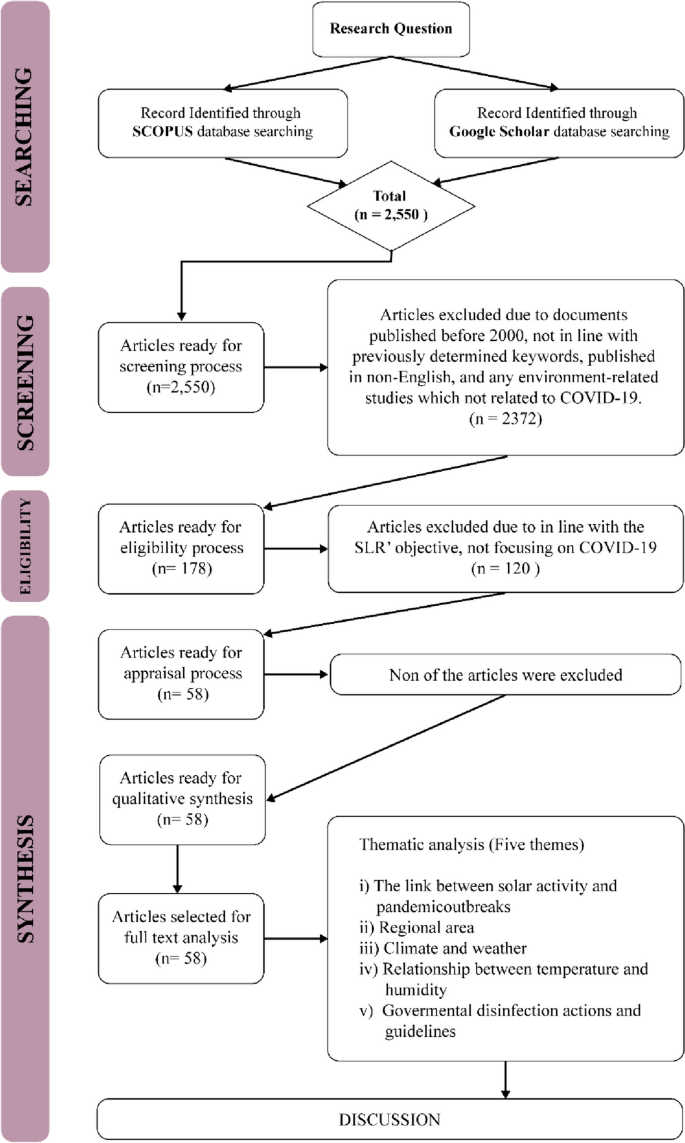
The flow diagram guide by ROSES protocol and Thematical Analysis
The current SLR started by determining the appropriate research questions, followed by the selection criteria, including the review, specifically on the keywords employed and the selection of journals database. Subsequently, the appraisal quality process and data abstraction and analysis were conducted.
Formulation of research questions
The entire process of this SLR was guided by the specific research questions, while sources to be reviewed and data abstraction and analysis were in line with the determined research question [ 35 , 36 ]. In the present article, a total of five research questions were formed, namely:
What the link between solar activity and COVID-19 pandemic outbreaks?
Which regions were more prone to COVID-19?
What were the temporal and spatial variabilities of high temperature and humidity during the spread of COVID-19?
What is the relationship between temperature and humidity in propagating COVID-19?
How did the government’s disinfection actions and guidelines can be reducing the spread of COVID-19?
Systematic searching strategies
Selection of studies.
In this stage of the study, the appropriate keywords to be employed in the searching process were determined. After referring to existing literature, six main keywords were chosen for the searching process, namely COVID-19, coronavirus, temperature, humidity, solar radiation and population density. The current study also utilised the boolean operators (OR, AND, AND NOT) and phrase searching.
Scopus was employed as the main database during the searching process, in line with the suggestion by Gusenbauer and Haddaway [ 37 ], who noted the strength of the database in terms of quality control and search and filtering functions. Furthermore, Google Scholar was selected as the supporting database. Although Halevi et al. [ 38 ] expressed concerns about its quality, Haddaway et al. [ 39 ] reported that due to its quantity, Google Scholar was suitable as a supporting database in SLR studies.
In the first stage of the search, 2550 articles were retrieved, which were then screened. The suitable criteria were also determined to control the quality of the articles reviewed [ 40 ]. The criteria are: any documents published between 2000 to 2022, documents that consist previously determined keywords, published in English, and any environment-related studies that focused on COVID-19. Based on these criteria, 2372 articles were excluded and 178 articles were proceeded to the next step namely eligibility. In the eligibility process, the title and the abstract of the articles were examined to ensure its relevancy to the SLR and in this process a total of 120 articles were excluded and only 58 articles were processed in the next stage.
Appraisal of the quality
The study ensured the rigor of the chosen articles based on best evidence synthesis. In the process, predefined inclusion criteria for the review were appraised by the systematic review team based on previously established guidelines and the studies were then judged as being scientifically admissible or not [ 40 ]. Hence, by controlling the quality based on the best evidence synthesis, the present SLR controls its quality by including articles that are in line with the inclusion criteria. It means that any article published within the timeline (in the year 2000 and above), composed of predetermined keywords, in English medium, and environment-related investigations focusing on COVID-19 are included in the review. Based on this process, all 58 articles fulfilled all the inclusion criteria and are considered of good quality and included in the review.
Data abstraction and analysis
The data abstraction process in this study was performed based on five research questions (please refer to 2.2, formulation of research questions). The data that was able to answer the questions were abstracted and placed in a table to ease the data analysis process. The primary data analysis technique employed in the current study was qualitative and relied on thematic analysis.
The thematic technique is a descriptive method that combines data flexibly with other information evaluation methods [ 41 ], aiming to identify the patterns in studies. Any similarities and relationships within the abstracted data emerge as patterns. Subsequently, suitable themes and sub-themes would be developed based on obtained patterns [ 42 ]. Following the thematic process, five themes were selected in this study.
Background of the selected articles
The current study selected 58 articles for the SLR. Five themes were developed based on the thematic analysis from the predetermined research questions: the link between solar activity and pandemic outbreaks, regional area, climate and weather, the relationship between temperature and humidity, and government disinfection action guidelines. Among the articles retrieved between 2000 and 2022; two were published in 2010, one in 2011, four in 2013, three in 2014, two in 2015, six in 2016 and 2017, respectively, one in 2018, six in 2019, twelve in 2020, eight in 2021, and seven in 2022.
Temperature- and humidity-related themes
The link between solar activity and pandemic outbreaks.
Numerous scientists have investigated the relationship between solar activities and pandemic outbreaks over the years ([ 43 ]; A [ 27 , 44 , 45 ].). Nuclear fusions from solar activities have resulted in minimum and maximum solar sunspots. Maximum solar activities are characterised by a high number of sunspots and elevated solar flare frequency and coronal mass injections. Minimum solar sunspot occurrences are identified by low interplanetary magnetic field values entering the earth [ 1 ].
A diminished magnetic field was suggested to be conducive for viruses and bacteria to mutate, hence the onset of pandemics. Nonetheless, Hoyle and Wickramasinghe [ 46 ] reported that the link between solar activity and pandemic outbreaks is only speculative. The literature noted that the data recorded between 1930 and 1970 demonstrated that virus transmissions and pandemic occurrences were coincidental. Moreover, no pandemic cases were reported in 1979, when minimum solar activity was recorded [ 47 ].
Chandra Wickramasinghe et al. [ 48 ] suggested a significant relationship between pandemic outbreaks and solar activities as several grand solar minima, including Sporer (1450–1550 AD), Mounder (1650–1700 AD), and Dalton (1800–1830) minimums, were recorded coinciding with global pandemics of diseases, such as smallpox, the English sweat, plague, and cholera pandemics. Furthermore, since the Dalton minimum, which recorded minimum sunspots, studies from 2002 to 2015 have documented the reappearance of previous pandemics. For example, influenza subtype H1N1 1918/1919 episodically returned in 2009, especially in India, China, and other Asian countries. Zika virus, which first appeared in 1950, flared and became endemic in 2015, transmitted sporadically, specifically in African countries. Similarly, SARS-CoV was first recorded in China in 2002 and emerged as an outbreak, MERS-CoV, in middle east countries a decade later, in 2012.
In 2020, the World Data Centre Sunspot Index and Long-term Solar Observations ( http://sidc.be ) confirmed that a new solar activity was initiated in December 2019, during which a novel coronavirus pandemic also occurred, and present a same as the previous hypothesis. Nevertheless, a higher number of pandemic outbreaks were documented during low minimum solar activities, including Ebola (1976), H5N1 (Nipah) (1967–1968), H1N1 (2009), and COVID-19 (2019–current). Furthermore, Wickramasinghe and Qu [ 49 ] reported that since 1918 or 1919, more devastating and recurrent pandemics tend to occur, particularly after a century. Consequently, within 100 years, a sudden surge of influenza was recorded, and novel influenza was hypothesised to emerge.
Figure 4 demonstrates that low minimum solar activity significantly reduced before 2020, hence substantiating the claim that pandemic events are closely related to solar activities. Moreover, numerous studies (i.e. [ 43 ], Chandra [ 46 , 47 , 48 ]) reported that during solar minimums, new viruses could penetrate the surfaces of the earth and high solar radiation would result in lower infection rates, supporting the hypothesis mentioned above.
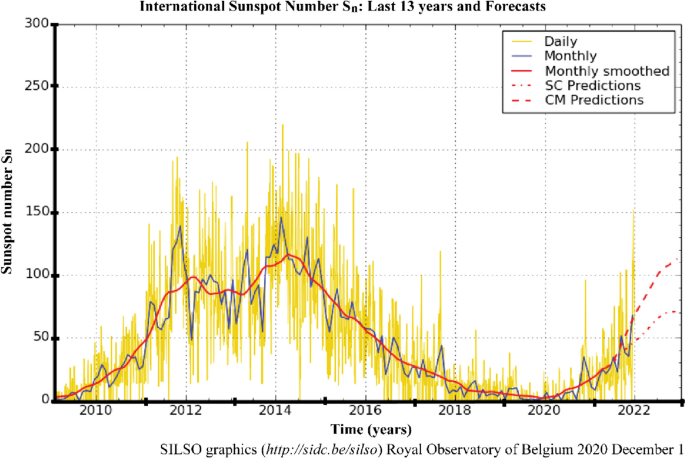
The number of sunspots in the last 13 years. Note : The yellow curve indicates the daily sunspot number and the 2010–2021 delineated curve illustrates the minimum solar activity recorded (source: http://sidc.be/silso )
Regional area
In early December 2019, Wuhan, China, was reported as the centre of the severe acute respiratory syndrome coronavirus 2 (SARS-CoV-2) outbreak [ 50 ]. Chinese health authorities immediately investigated and controlled the spread of the disease. Nevertheless, by late January 2020, the WHO announced that COVID-19 was a global public health emergency. The upgrade was due to the rapid rise in confirmed cases, which were no longer limited to Wuhan [ 28 ]. The disease had spread to 24 other countries, which were mainly in the northern hemisphere, particularly the European and Western Pacific regions, such as France, United Kingdom, Spain, South Korea, Japan, Malaysia, and Indonesia [ 51 , 52 ]. The migration or movement of humans was the leading agent in the spread of COVID-19, resulting in an almost worldwide COVID-19 pandemic [ 53 ].
The first hotspots of the epidemic outspread introduced by the Asian and Western Pacific regions possessed similar winter climates with an average temperature and humidity rate of 5–11 °C and 47–79%. Consequently, several publications reviewed in the current study associated the COVID-19 outbreak with regional climates (i.e. [ 1 , 29 , 54 , 55 ]) instead of its close connection to China. This review also discussed the effects of a range of specific climatological variables on the transmission and epidemiology of COVID-19 in regional climatic conditions.
America and Europe documented the highest COVID-19 cases, outnumbering the number reported in Asia [ 19 ] and on the 2nd of December 2020, the United States of America (USA) reported the highest number of confirmed COVID-19 infections, with over 13,234,551 cases and 264,808 mortalities (Da S [ 56 ].). The cases in the USA began emerging in March 2020 and peaked in late November 2020, during the wintertime in the northern hemisphere (December to March) [ 53 ]. Figure 5 demonstrates the evolution of the COVID-19 pandemic in several country which represent comparison two phase of summer and one phase of winter. Most of these countries tend to increase of COVID cases close to winter season. Then, it can be worsening on phase two of summer due to do not under control of human movement although the normal trend it is presenting during winter phase.
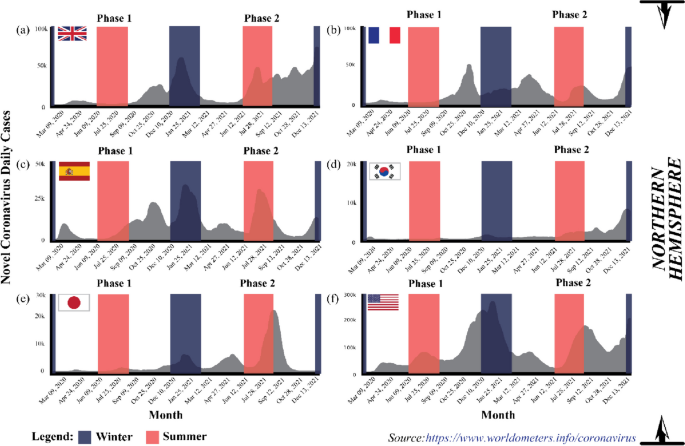
The evolution of the COVID-19 pandemic from the 15th of February 2020 to the 2nd of December 2020 ( Source: https://www.worldometers.info/coronavirus )
The coronavirus spread aggressively across the European region, which recorded the second highest COVID-19 confirmed cases after America. At the end of 2020, WHO reported 19,071,275 Covid-19 cases in the area, where France documented 2,183,275 cases, the European country with the highest number of confirmed cases, followed by the United Kingdom (1,629,661 cases) and Spain (1,652,801 cases) [ 19 ]. Europe is also located in the northern hemisphere and possesses a temperate climate.
The spatial and temporal transmission patterns of coronavirus infection in the European region were similar to America and the Eastern Mediterranean, where the winter season increased COVID-19 cases. Typically, winter in Europe occurs at the beginning of October and ends in March. Hardy et al. [ 57 ] also stated that temperature commonly drops below freezing (approximately − 1 °C) when snow accumulates between December to mid-March, resulting in an extreme environment. Figure 5 indicates that COVID-19 cases peaked in October when the temperature became colder [ 21 ]. Similarly, the cases were the highest in the middle of the year in Australia and South Asian countries, such as India, that experience winter and monsoon, respectively, during the period.
In African regions, the outbreak of COVID-19 escalated rapidly from June to October before falling from October to March, as summer in South Africa generally occurs from November to March, while winter from June to August. Nevertheless, heavy rainfall generally transpires during summer, hence the warm and humid conditions in South Africa and Namibia during summer, while the opposite happens during winter (cold and dry). Consequently, the outbreak in the region recorded an increasing trend during winter and subsided during the summer, supporting the report by Gunthe et al. [ 58 ]. Novel coronavirus disease presents unique and grave challenges in Africa, as it has for the rest of the world. However, the infrastructure and resources have limitations for Africa countries facing COVID-19 pandemic and the threat of other diseases [ 59 ].
Conclusively, seasonal and regional climate patterns were associated with COVID-19 outbreaks globally. According to Kraemer et al. [ 60 ], they used real-time mobility data in Wuhan and early measurement presented a positive correlation between human mobility and spread of COVID-19 cases. However, after the implementation of control measures, this correlation dropped and growth rates became negative in most locations, although shifts in the demographics of reported cases were still indicative of local chains of transmission outside of Wuhan.
Climate and weather
The term “weather” represents the changes in the environment that occur daily and in a short period, while “climate” is defined as atmospheric changes happening over a long time (over 3 months) in specific regions. Consequently, different locations would experience varying climates. Numerous reports suggested climate and weather variabilities as the main drivers that sped or slowed the transmission of SARS-CoV-2 worldwide [ 44 , 61 , 62 , 63 ].
From a meteorological perspective, a favourable environment has led to the continued existence of the COVID-19 virus in the atmosphere [ 64 ]. Studies demonstrated that various meteorological conditions, such as the rate of relative humidity (i.e. [ 28 ]), precipitation (i.e. [ 65 ]), temperature (i.e. [ 66 ]), and wind speed factors (i.e. [ 54 ]), were the crucial components that contributed to the dynamic response of the pandemic, influencing either the mitigation or exacerbation of novel coronavirus transmission. In other words, the environment was considered the medium for spreading the disease when other health considerations were put aside. Consequently, new opinions, knowledge, and findings are published and shared to increase awareness, thus encouraging preventive measures within the public.
The coronavirus could survive in temperatures under 30 °C with a relative humidity of less than 80% [ 67 ], suggesting that high temperatures and lower relative humidity contributed to the elicitation of COVID-19 cases [ 18 , 51 , 58 , 68 ]. Lagtayi et al. [ 7 ] highlighted temperature as a critical factor, evidently from the increased transmission rate of MERS-Cov in African states with a warm and dry climate. Similarly, the highest COVID-19 cases were recorded in dry temperate regions, especially in western Europe (France and Spain), China, and the USA, while the countries nearer to the equator were less affected. Nevertheless, the temperature factor relative to viral infections depends on the protein available in the viruses. According to Chen and Shakhnovich [ 69 ], there is a good correlation between decreasing temperature and the growth of proteins in virus. Consequently, preventive measures that take advantage of conducive environments for specific viruses are challenging.
Precipitation also correlates with influenza [ 43 ]. A report demonstrated that regions with at least 150 mm of monthly precipitation threshold level experienced fewer cases than regions with lower precipitation rates. According to Martins et al. [ 70 ], influenza and COVID-19 can be affected by climate, where virus can be spread through the respiratory especially during rainfall season. The daily spread of Covid-19 cases in tropical countries, which receive high precipitation levels, are far less than in temperate countries [ 27 ]. Likewise, high cases of COVID-19 were reported during the monsoon season (mid-year) in India during which high rainfall is recorded [ 71 ]. Moreover, the majority of the population in these regions has lower vitamin D levels, which may contribute to weakened immune responses during certain seasons [ 27 ].
Rainfall increases the relative atmospheric humidity, which is unfavourable to the coronaviruses as its transmission requires dry and cold weather. Moreover, several reports hypothesised that rain could wash away viruses on object surfaces, which is still questioned. Most people prefer staying home on rainy days, allowing less transmission or close contact. Conversely, [ 72 ] exhibited that precipitation did not significantly impact COVID-19 infectiousness in Oslo, Norway due the location in northern hemisphere which are during winter season presenting so cold.
Coşkun et al. [ 54 ] and Wu et al. [ 29 ] claimed that wind could strongly correlate with the rate of COVID-19 transmission. Atmospheric instability (turbulent occurrences) leads to increased wind speed and reduces the dispersion of particulate matter (PM 2.5 and PM 10 ) in the environment and among humans. An investigation performed in 55 cities in Italy during the COVID-19 outbreak proved that the areas with low wind movement (stable atmospheric conditions) possessed a higher correlation coefficient and exceeded the threshold value of the safe level of PM 2.5 and PM 10 . Resultantly, more individuals were recorded infected with the disease in the regions. As mentioned in Martins et al. [ 70 ] the COVID-19 can be affected by climate and the virus can be spread through respiratory which is the virus moving in the wind movement.
The relationship between temperature and humidity
Climatic parameters, such as temperature and humidity, were investigated as the crucial factors in the epidemiology of the respiratory virus survival and transmission of COVID-19 ([ 61 ]; S [ 73 , 74 ].). The rising number of confirmed cases indicated the strong transmission ability of COVID-19 and was related to meteorological parameters. Furthermore, several studies found that the disease transmission was associated with the temperature and humidity of the environment [ 55 , 64 , 68 , 75 ], while other investigations have examined and reviewed environmental factors that could influence the epidemiological aspects of Covid-19.
Generally, increased COVID-19 cases and deaths corresponded with temperature, humidity, and viral transmission and mortality. Various studies reported that colder and dryer environments favoured COVID-19 epidemiologically [ 45 , 76 , 77 ]. As example tropical region, the observations indicated that the summer (middle of year) and rainy seasons (end of the year) could effectively diminish the transmission and mortality from COVID-19. High precipitation statistically increases relative air humidity, which is unfavourable for the survival of coronavirus, which prefers dry and cold conditions [ 32 , 34 , 78 , 79 ]. Consequently, warmer conditions could reduce COVID-19 transmission. A 1 °C increase in the temperature recorded a decrease in confirmed cases by 8% increase [ 45 ].
Several reports established that the minimum, maximum, and average temperature and humidity correlated with COVID-19 occurrence and mortality [ 55 , 80 , 81 ]. The lowest and highest temperatures of 24 and 27.3 °C and a humidity between 76 and 91% were conducive to spreading the virulence agents. The propagation of the disease peaked at the average temperature of 26 °C and humidity of 55% before gradually decreasing with elevated temperature and humidity [ 78 ].
Researchers are still divided on the effects of temperature and humidity on coronavirus transmission. Xu et al. [ 26 ] confirmed that COVID-19 cases gradually increased with higher temperature and lower humidity, indicating that the virus was actively transmitted in warm and dry conditions. Nevertheless, several reports stated that the spread of COVID-19 was negatively correlated with temperature and humidity [ 10 , 29 , 63 ]. The conflicting findings require further investigation. Moreover, other factors, such as population density, elderly population, cultural aspects, and health interventions, might potentially influence the epidemiology of the disease and necessitate research.
Governmental disinfection actions and guidelines
The COVID-19 is a severe health threat that is still spreading worldwide. The epidemiology of the SAR-CoV-2 virus might be affected by several factors, including meteorological conditions (temperature and humidity), population density, and healthcare quality, that permit it to spread rapidly [ 16 , 17 ]. Nevertheless, in 2020, no effective pharmaceutical interventions or vaccines were available for the diagnosis, treatment, and epidemic prevention against COVID-19 [ 73 , 82 ]. Consequently, after 2020 the governments globally have designed and executed non-pharmacological public health measures, such as lockdown, travel bans, social distancing, quarantine, public place closure, and public health actions, to curb the spread of COVID-19 infections and several studies have reported on the effects of these plans [ 13 , 83 ].
The COVID-19 is mainly spread via respiratory droplets from an infected person’s mouth or nose to another in close contact [ 84 ]. Accordingly, WHO and most governments worldwide have recommended wearing facemasks in public areas to curb the transmission of COVID-19. The facemasks would prevent individuals from breathing COVID-19-contaminated air [ 85 ]. Furthermore, the masks could hinder the transmission of the virus from an infected person as the exhaled air is trapped in droplets collected on the masks, suspending it in the atmosphere for longer. The WHO also recommended adopting a proper hand hygiene routine to prevent transmission and employing protective equipment, such as gloves and body covers, especially for health workers [ 86 ].
Besides wearing protective equipment, social distancing was also employed to control the Covid-19 outbreak [ 74 , 87 ]. Social distancing hinders the human-to-human transmission of the coronavirus in the form of droplets from the mouth and nose, as evidenced by the report from Sun and Zhai [ 88 ]. Conversely, Nair & Selvaraj [ 89 ] demonstrated that social distancing was less effective in communities and cultures where gatherings are the norm. Nonetheless, the issue could be addressed by educating the public and implementing social distancing policies, such as working from home and any form of plague treatment.
Infected persons, individuals who had contact with confirmed or suspected COVID-19 patients, and persons living in areas with high transmission rates were recommended to undergo quarantine by WHO. The quarantine could be implemented voluntarily or legally enforced by authorities and applicable to individuals, groups, or communities (community containment) [ 90 ]. A person under mandatory quarantine must stay in a place for a recommended 14-day period, based on the estimated incubation period of the SARS-CoV-2 [ 19 , 91 ]. According to Stasi et al. [ 92 ], 14-days period for mandatory quarantine it is presenting a clinical improvement after they found 5-day group and 10-day group can be decrease number of patient whose getting effect of COVID-19 from 64 to 54% respectively. This also proven by Ahmadi et al. [ 43 ] and Foad et al. [ 93 ], quarantining could reduce the transmission of COVID-19.
Lockdown and travel bans, especially in China, the centre of the coronavirus outbreak, reduced the infection rate and the correlation of domestic air traffic with COVID-19 cases [ 17 ]. The observations were supported by Sun & Zhai [ 88 ] and Sun et al. [ 94 ], who noted that travel restrictions diminished the number of COVID-19 reports by 75.70% compared to baseline scenarios without restrictions. Furthermore, example in Malaysia, lockdowns improved the air quality of polluted areas especially in primarily at main cities [ 95 ]. As additional, Martins et al. [ 70 ] measure the Human Development Index (HDI) with the specific of socio-economic variables as income, education and health. In their study, the income and education levels are the main relevant factors that affect the socio-economic.
A mandatory lockdown is an area under movement control as a preventive measure to stop the coronavirus from spreading to other areas. Numerous governments worldwide enforced the policy to restrict public movements outside their homes during the pandemic. Resultantly, human-to-human transmission of the virus was effectively reduced. The lockdown and movement control order were also suggested for individuals aged 80 and above or with low or compromised immunities, as these groups possess a higher risk of contracting the disease [ 44 ].
Governments still enforced movement orders even after the introduction of vaccines by Pfizer, Moderna, and Sinovac, as the vaccines only protect high-risk individuals from the worst effects of COVID-19. Consequently, in most countries, after receiving the first vaccine dose, individuals were allowed to resume life as normal but were still required to follow the standard operating procedures (SOP) outlined by the government.
The government attempted to balance preventing COVID-19 spread and recovering economic activities, for example, local businesses, maritime traders, shipping activities, oil and gas production and economic trades [ 22 , 96 ]. Nonetheless, the COVID-19 cases demonstrated an increasing trend during the summer due to the higher number of people travelling and on vacation, primarily to alleviate stress from lockdowns. Several new variants were discovered, including the Delta and Omicron strains, which spread in countries such as the USA and the United Kingdom. The high number of COVID-19 cases prompted the WHO to suggest booster doses to ensure full protection.
As mentioned in this manuscript, the COVID-19 still uncertain for any kind factors that can be affected on spreading of this virus. However, regarding many sources of COVID-19 study, the further assessment on this factor need to be continue to be sure, that we ready to facing probably in 10 years projection of solar minimum phase can be held in same situation for another pandemic.
The sun has an eleven-year cycle known as the solar cycle, related to its magnetic field, which controls the activities on its surface through sunspots. When the magnetic fields are active, numerous sunspots are formed on its surface, hence the sun produces more radiation energy emitted to the earth. The condition is termed solar maximum (see Fig. 6 , denoted by the yellow boxes). Alternatively, as the magnetic field of the sun weakens, the number of sunspots decreases, resulting in less radiation energy being emitted to the earth. The phenomenon is known as the solar minimum (see Fig. 6 , represented by the blue boxes).
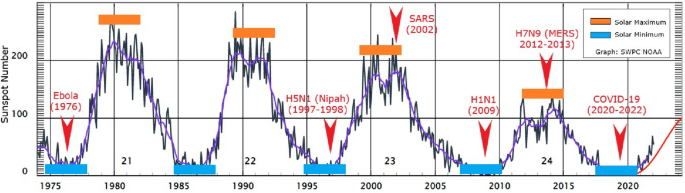
The emergence and recurrence of pandemics every 5 years in relation to solar activities ( Source: www.swpc.noaa.gov/ ). Note: The yellow boxes indicate the solar maximum, while the blue boxes represent the solar minimum
The magnetic field of the sun protects the earth from cosmic or galactic cosmic rays emitted by supernova explosions, stars, and gamma-ray bursts [ 97 ]. Nevertheless, galactic cosmic rays could still reach the earth during the solar minimum, the least solar radiation energy period. In the 20th and early 21st centuries, several outbreaks of viral diseases that affected the respiratory system (pneumonia or influenza), namely the Spanish (1918–1919), Asian (1957–1958) and Hong Kong (1968) flu, were documented. Interestingly, the diseases that claimed numerous lives worldwide occurred at the peak of the solar maximum.
Figure 6 illustrates the correlation between the number of sunspots and disease outbreaks from 1975 to 2021, including COVID-19, that began to escalate in December 2019. Under the solar minimum conditions, the spread of Ebola (1976), H5N1 (1997–1998), H1N1 (2009), and COVID-19 (2019-2020) were documented, while the solar maximum phenomenon recorded SARS (2002) and H7N9 (2012–2013) or MERS outbreaks. Nonetheless, solar activity through the production of solar sunspots began to decline since the 22nd solar cycle. Accordingly, further studies are necessary to investigate the influence such solar variations could impart or not on pandemic development.
Despite the findings mentioned above, the sun and cosmic radiations could influence the distribution or outspread of disease-spreading viruses. The rays could kill the viruses via DNA destruction or influence their genetic mutations, which encourage growth and viral evolution. Nevertheless, the connection between radiation and the evolutionary process requires further study by specialists in the field it is become true or not.
The spread of viral diseases transpires naturally in our surroundings and occurs unnoticed by humans. According to records, the spread of pandemic diseases, including the Black Death (fourteenth century) and the Spanish flu (1919), was significantly influenced by the decline and peak of solar activities. Furthermore, in the past 20 years, various diseases related to the influenza virus have been recorded. According to the pattern observed, if all diseases were related to the solar cycle (solar maximum and minimum), the viral diseases would reoccur every 5 to 6 years since they first appeared between 1995 and 2020. Accordingly, the next pandemic might occur around 2024 or 2025 and need to have a proper study for prove these statements. Nonetheless, the activities on the surface of the sun have been weakening since the 23rd solar cycle and it can be proven later after the proper study can be make it.
The beginning of the COVID-19 spread, only several countries with the same winter climate with an average temperature of 5–11 °C and an average humidity rate of 47–79% located at latitudes 30–50 N reported cases. The areas included Wuhan distribution centres in China, the United Kingdom, France, Spain, South Korea, Japan, and the USA (see Fig. 5 ). Other than biological aspects, the higher number of confirmed cases recorded in colder environments was due to the human body secreting less lymphoproliferative hormone, leading to decreased immunogenicity effects and increased risk of infection [ 24 ]. Consequently, the virus could attack and rapidly infect humans during the period [ 1 , 54 ].
The lymphoproliferative response is a protective immune response that plays a vital role in protecting and eradicating infections and diseases. On the other hand, staying in warm conditions or being exposed to more sunlight would lower the risks of infection. According to Asyary and Veruswati [ 98 ], sunlight triggers vitamin D, which increases immunity and increases the recovery rates of infected individuals.
Researchers believe that viruses could survive in the environment for up to 3 to 4 years or even longer. The survival rate of the microorganisms is relatively high, which is related to their biological structures, adaptability on any surfaces, and transmission medium to spread diseases. Viruses possess simple protein structures, namely the spike, membrane, and envelope protein; therefore, when they enter living organisms (such as through the respiratory system), the viruses are easily transmitted.
Once they have entered a host, the viruses duplicate exponentially and swarm the lungs. Subsequently, after the targeted organs, such as the lungs, are invaded, the viruses attack the immune system and create confusion in protective cells to destroy healthy cells. The situation is still considered safe in younger and healthy individuals as their immune systems could differentiate and counter-attack the viruses, curing them. Nonetheless, in elders and individuals with several chronic diseases, most of their protective cells are dead, hence their immune system is forced to work hard to overcome the infection. Pneumonia and death tend to occur when the situation is overwhelming [ 85 ]. Consequently, the viruses are harmful to humans as they could multiply in a short period, enter the blood, and overrun the body.
The coronavirus could attach to surfaces without a host, including door knobs and steel and plastic materials. The microorganisms could survive alone, but virologists have yet to determine how long. If someone touches any surface with the virus, the individual would then be infected. The situation would worsen if the infected person contacted numerous people and became a super spreader. A super spreader does not exhibit any symptoms and continuously transmits the virus without realising it. An infected individual transmits the coronavirus via droplets from coughs or sneezes. Nevertheless, scientists have yet to determine if coronavirus is spread via airborne or droplets, hence requiring thorough evaluation [ 99 ].
The COVID-19 virus mutates over time, and it can be changing any times. Mutations alter the behaviour and genetic structure of the virus, resulting in a new strain. Numerous research have been conducted to procure vaccines and anti-viral medications, but mutations have led to evolutionary disadvantages. The novel strains are more infectious than the original ones. As of November 2020, approximately six new coronavirus strains have been detected, each displaying different transmission behaviours [ 100 ].
Recent studies demonstrated that the mutated viruses exhibit little variability, allowing scientists to produce viable vaccines [ 71 ]. Furthermore, different types of vaccines are manufactured by different countries, which could be advantageous. Currently, most countries also recommend booster doses to attain extra protection after receiving the mandatory two vaccine doses. In same time, the social and physical interactions between humans also necessitate to be aware.
The COVID-19 virus is primarily transmitted through droplets produced by an infected person. Accordingly, physical distancing, a one-metre minimum distance between individuals [ 19 ], and following the SOP might prevent or avoid spreading the disease. Moreover, self-quarantine, school closures, working from home, cancelling large events, limiting gatherings, and avoiding spending long periods in crowded places are essential strategies in enforcing physical distancing at a community level. The policies are essential precautions that could reduce the further spreading of coronavirus and break the chain of transmission.
Government support also need to control the spread of COVID-19 with the strict SOP. The SOP enforcement in public places would enhance adherence to the new practice among the public and the community, aiding in curbing disease transmission. Practising limited meetings and social gatherings, avoiding crowded places, workplace distancing, preventing non-necessary travels of high-risk family members, especially those with chronic disease, and adhering to the recommended SOP could reduce coronavirus outbreaks. Nonetheless, individual awareness is also necessary to achieve COVID-19 spread prevention.
Many researchers are focused on identifying the primary drivers of pandemic outbreaks. Seasonal, temperature, and humidity differences significantly impacted COVID-19 growth rate variations. It is crucial to highlight the potential link between the recurrence of pandemics every 5 years and solar activities, which can influence temperature and humidity variations. Notable variations in COVID-19 mortality rates were observed between northern and southern hemisphere countries, with the former having higher rates. One hypothesis suggests that populations in the northern hemisphere may receive insufficient sunlight to maintain optimal vitamin D levels during winter, possibly leading to higher mortality rates.
The first COVID-19 case was detected in Wuhan, China, which is in the northern hemisphere. The number of cases rapidly propagated in December during the winter season. At the time, the temperature in Wuhan was recorded at 13–18 °C. Accordingly, one theory proposes that the survival and transmission of the coronavirus were due to meteorological conditions, namely temperatures between 13 and 18 °C and 50–80% humidity.
Daily rainfall directly impacts humidity levels. The coronavirus exhibited superior survival rates in cold and dry conditions. Furthermore, transmissible gastroenteritis (TGEV) suspensions and possibly other coronaviruses remain viable longer in their airborne states, which are more reliably collected in low relative humidity than in high humidity. Consequently, summer rains would effectively reduce COVID-19 transmission in southern hemisphere regions.
In southern hemisphere regions, the summer seasons are accompanied by a high average temperature at the end and beginning of the year. Countries with temperatures exceeding 24 °C reported fewer infections. As temperatures rise from winter to summer, virus transmission is expected to decline. Nonetheless, the activities and transmission of the virus were expected to decrease during winter to summer transitions, when the countries would be warmer. The peak intensity of infections strongly depends on the level of seasonal transmissions.
Social distancing plays a critical role in preventing the overload of healthcare systems. Many respiratory pathogens, including those causing mild common cold-like syndromes, show seasonal fluctuations, often peaking in winter. This trend can be attributed to increased indoor crowding, school reopening, and climatic changes during autumn.
The spread of COVID-19 to neighbouring regions can be attributed to population interactions. Migration patterns, such as the movement from northern to southern regions during the warmer months, have significant epidemiological impacts. This trend mirrors the behavior of influenza pandemics where minor outbreaks in spring or summer are often followed by major waves in autumn or winter.
Availability of data and materials
Not applicable.
Abbreviations
Novel coronavirus
Coronavirus disease 2019
Deoxyribonucleic acid
Swine influenza
Influenza A virus subtype H5N1
Asian Lineage Avian Influenza A(H7N9) Virus
Middle East respiratory syndrome
Middle East respiratory syndrome Coronavirus
Particulate matter
Preferred Reporting Items for Systematic Reviews and Meta-Analyses
RepOrting standards for Systematic Evidence Syntheses
Severe Acute Respiratory Syndrome
Severe Acute Respiratory Syndrome Coronavirus
Syndrome coronavirus 2
Systematic literature review
Standard operating procedure
Transmissible gastroenteritis Virus
United States of America
World Health Organization
Apanovich I. Climate and man. Opposition or natural stage of the earth’s evolution? Norwegian journal of development of the international. Science. 2019;26(25):12–27.
Google Scholar
Borah P, Singh MK, Mahapatra S. Estimation of degree-days for different climatic zones of north-East India. Sustain Cities Soc. 2015;14(1):70–81.
Article Google Scholar
Chen D, Chen HW. Using the Köppen classification to quantify climate variation and change: an example for 1901-2010. Environmental Development. 2013;6(1):69–79.
Trenberth KE, Fasullo JT. Global warming due to increasing absorbed solar radiation. Geophys Res Lett. 2009;36
Hauschild MZ, Huijbregts MAJ, Guinée L, Lane J, Fantke P, Zelm v R, et al. Life Cycle Impact Assessment – The Complete World of Life Cycle Assessment; 2015. p. 345.
Book Google Scholar
Nakada LYK, Urban RC. COVID-19 pandemic: environmental and social factors influencing the spread of SARS-CoV-2 in São Paulo. Brazil Environmental Science and Pollution Research. 2021;28(30):40322–8.
Article CAS PubMed Google Scholar
Lagtayi, R., Lairgi, L., Daya, A., & Khouya, A. (2021). The impact of the average temperature, humidity, wind speed, altitude and population density on daily COVID-19 infections’ evolution. January, 9094.
Majumder MS, Liu D, Poirier C, Mandl KD, Lipsitch M, The MS. The role of absolute humidity on transmission rates of the COVID-19 outbreak; 2020.
Wiersinga WJ, Rhodes A, Cheng AC, Peacock SJ, Prescott HC. Pathophysiology, transmission, diagnosis, and treatment of coronavirus disease 2019 (COVID-19): A review. JAMA - Journal of the American Medical Association. 2020;324(8):782–93.
Zhang X, Maggioni V, Houser P, Xue Y, Mei Y. The impact of weather condition and social activity on COVID-19 transmission in the United States. J Environ Manag. 2022;302:114085.
Article CAS Google Scholar
Mäkinen TM, Juvonen R, Jokelainen J, Harju TH, Peitso A, Bloigu A, et al. Cold temperature and low humidity are associated with increased occurrence of respiratory tract infections. Respir Med. 2009;103(3):456–62.
Article PubMed Google Scholar
Carvalho FRS, Henriques DV, Correia O, Schmalwieser AW. Potential of solar UV radiation for inactivation of Coronaviridae family. Photochem Photobiol. 2021;97:213–20.
Ali I, Alharbi OM. COVID-19: disease, management, treatment, and social impact. Sci Total Environ. 2020;728:138861.
Article CAS PubMed PubMed Central Google Scholar
Jin Y, Yang H, Ji W, Wu W, Chen S, Zhang W, et al. Virology, epidemiology, pathogenesis, and control of covid-19. Viruses. 2020;12(4):1–17.
Sohrabi C, Alsafi Z, O’Neill N, Khan M, Kerwan A, Al-Jabir A, et al. World health organization declares global emergency: A review of the 2019 novel coronavirus (COVID-19). Int J Surg. 2020;76:71–6.
Article PubMed PubMed Central Google Scholar
Wang J, Tang K, Feng K, Lv W. High temperature and high humidity reduce the transmission of COVID-19; 2020a.
Wang L, Duan Y, Zhang W, Liang J, Xu J, Zhang Y, et al. Epidemiologic and clinical characteristics of 26 cases of covid-19 arising from patient-to-patient transmission in Liaocheng, China. Clinical Epidemiology. 2020b;12:387–91.
Xie J, Zhu Y. Science of the Total environment association between ambient temperature and COVID-19 infection in 122 cities from China. Sci Total Environ. 2020;724:138201.
World Health Organization. (2020a). Director-General’s opening remarks at the media briefing on COVID-19-10April 2020. https://www.who.int/dg/speeches/detail/who-director-general-s-opening-remarks-at-the-media-briefing-on-covid-19—10- april-2020.
Bostock B. South Korea is testing 200,000 members of a doomsday church linked to more than 60% of its coronavirus cases; 2020.
World Health Organization. (2020b). Health topics/coronavirus. https://www.who.int/health-topics/coronavirus#tab=tab_1.
Menhat M, Mohd Zaideen IM, Yusuf Y, Salleh NHM, Zamri MA, Jeevan J. The impact of Covid-19 pandemic: A review on maritime sectors in Malaysia. Ocean Coast Manag. 2021;209:105638.
Byun WS, Heo SW, Jo G, Kim JW, Kim S, Lee S, et al. Is coronavirus disease (COVID-19) seasonal? A critical analysis of empirical and epidemiological studies at global and local scales. Environ Res. 2021;196:110972.
Dhakal P, Pokhrel P, B. Seasonal variation and COVID-19 pandemic in Nepal. Nepal Medical Journal. 2020;3(2):77–80.
Mehmet Ş. Science of the Total environment impact of weather on COVID-19 pandemic in Turkey. 728; 2020.
Xu H, Yan C, Fu Q, Xiao K, Yu Y, Han D, et al. Science of the Total environment possible environmental effects on the spread of COVID-19 in China. Sci Total Environ. 2020;731:139211.
Rosario DKA, Mutz YS, Bernardes PC, Conte-Junior CA. Relationship between COVID-19 and weather: case study in a tropical country. Int J Hyg Environ Health. 2020;229:113587.
Wang J, Tang K, Feng K, Lin X, Lv W, Chen K, et al. Impact of temperature and relative humidity on the transmission of COVID-19: A modelling study in China and the United States. BMJ Open. 2021;11(2):1–16.
Wu Y, Jing W, Liu J, Ma Q, Yuan J, Wang Y, et al. Effects of temperature and humidity on the daily new cases and new deaths of COVID-19 in 166 countries. Sci Total Environ. 2020;729:1–7.
Casanova LM, Jeon S, Rutala WA, Weber DJ, Sobsey MD. Effects of air temperature and relative humidity on coronavirus survival on surfaces. Appl Environ Microbiol. 2010;76(9):2712–7.
Islam N, Bukhari Q, Jameel Y, Shabnam S, Erzurumluoglu AM, Siddique MA, et al. COVID-19 and climatic factors: A global analysis. Environ Res. 2021;193:110355.
Diao Y, Kodera S, Anzai D, Gomez-Tames J, Rashed EA, Hirata A. Influence of population density, temperature, and absolute humidity on spread and decay durations of COVID-19: A comparative study of scenarios in China, England, Germany, and Japan. One Health. 2021;12:100203.
Haddaway NR, Macura B, Whaley P, Pullin AS. ROSES reporting standards for systematic evidence syntheses: pro forma, flow-diagram and descriptive summary of the plan and conduct of environmental systematic reviews and systematic maps. Environ Evid. 2018;7(1):4–11.
Sharif N, Dey SK. Impact of population density and weather on COVID-19 pandemic and SARS-CoV-2 mutation frequency in Bangladesh. Epidemiol Infect. 2021:1–10.
Kraus S, Breier Dasí-Rodríguez S. El arte de elaborar una revisión bibliográfica sistemática en la investigación sobre el espíritu empresarial. Int Entrep Manag J. 2020;16:1023–42.
Xiao Y, Watson M. Guidance on conducting a systematic literature review. J Plan Educ Res. 2019;39(1):93–112.
Gusenbauer M, Haddaway NR. Which academic search systems are suitable for systematic reviews or meta-analyses? Evaluating retrieval qualities of Google scholar, PubMed, and 26 other resources. Res Synth Methods. 2020;11(2):181–217.
Halevi G, Moed H, Bar-Ilan J. Suitability of Google scholar as a source of scientific information and as a source of data for scientific evaluation—review of the literature. Journal of Informetrics. 2017;11(3):823–34.
Haddaway NR, Collins AM, Coughlin D, Kirk S. The role of google scholar in evidence reviews and its applicability to grey literature searching. PLoS One. 2015;10(9):1–17.
Littlewood C, Chance-Larsen K, McLean S. Quality appraisal as a part of the systematic review. International Journal of Physiotherapy and Rehabilitation. 2010;1(1):53–8.
Vaismoradi M, Turunen H, Bondas T. Content analysis and thematic analysis: implications for conducting a qualitative descriptive study. Nurs Health Sci. 2013;15(3):398–405.
Braun V, Clarke V. Using thematic analysis in psychology. Qual Res Psychol. 2006;3:77–101.
Ahmadi M, Sharifi A, Dorosti S, Jafarzadeh Ghoushchi S, Ghanbari N. Investigation of effective climatology parameters on COVID-19 outbreak in Iran. Sci Total Environ. 2020;729
Gupta A, Banerjee S, Das S. Significance of geographical factors to the COVID-19 outbreak in India. Modeling Earth Systems and Environment. 2020;6(4):2645–53.
Pequeno P, Mendel B, Rosa C, Bosholn M, Souza JL, Baccaro F, et al. Air transportation, population density and temperature predict the spread of COVID-19 in Brazil. PeerJ. 2020;2020(6):1–15.
Hoyle F, Wickramasinghe NC. Sunspots and influenza [6]. Nature. 1990;343(6256):304.
Wickramasinghe NC, Rocca MC, Tokoro G, Temple R. Journal of infectious diseases. Scienctific Research and Community. 2020;1(4):1–10.
Wickramasinghe NC, Steele EJ, Wainwright M, Tokoro G, Fernando M, Qu J. Sunspot cycle minima and pandemics : A case for vigilance at the present time. Journal of Astrobiology & Outreach. 2017;5:2332–519.
Wickramasinghe NC, Qu J. Are we approaching a new influenza pandemic. Virol Curr Res. 2018;2(107):2.
Guan W, Ni Z, Hu Y, Liang W, Ou C, He J, et al. Disease 2019 in China; 2020.
Bashir MF, Ma B, Bilal K, Bashir MA, Tan D, Bashir M. Correlation between climate indicators and COVID-19 pandemic in New York, USA. Sci Total Environ. 2020;728:138835.
Cucinotta D, Vanelli M. WHO declares COVID-19 a pandemic. Acta Biomed. 2020;91:157–60.
PubMed PubMed Central Google Scholar
Lin C, Lau AKH, Fung JCH, Guo C, Chan JWM, Yeung DW, et al. A mechanism-based parameterisation scheme to investigate the association between transmission rate of COVID-19 and meteorological factors on plains in China. Sci Total Environ. 2020;737:140348.
Coşkun H, Yıldırım N, Gündüz S. The spread of COVID-19 virus through population density and wind in Turkey cities. Sci Total Environ. 2021;751
Yang HY, Lee JKW. The impact of temperature on the risk of covid-19: A multinational study. Int J Environ Res Public Health. 2021;18(8)
Candido DD, Watts A, Abade L, Kraemer MUG, Pybus OG, Croda J, et al. Routes for COVID-19 importation in Brazil. Journal of Travel Medicine. 2020;27(3):1–3.
Hardy JP, Groffman PM, Fitzhugh RD, Henry KS, Welman AT, Demers JD, et al. Snow depth manipulation and its influence on soil frost and water dynamics in a northern hardwood forest. Biogeochemistry. 2001;56(2):151–74.
Gunthe SS, Swain B, Patra SS, Amte A. On the global trends and spread of the COVID-19 outbreak: preliminary assessment of the potential relation between location-specific temperature and UV index. Journal of Public Health (Germany). 2020:1–10.
Rosenthal PJ, et al. COVID-19: shining the light on Africa. Am J Trop Med Hyg. 2020;102(6):1145–8.
Kraemer MUG, et al. The effect of human mobility and control measures on the COVID-19 epidemic in China. Science. 2020;368:493–7.
Dalziel BD, Kissler S, Gog JR, Viboud C, Bjørnstad ON, Metcalf CJE, et al. Urbanization and humidity shape the intensity of influenza epidemics in U.S. cities. Science. 2018;362:75–9.
Sahoo PK, Powell MA, Mittal S, Garg VK. Is the transmission of novel coronavirus disease (COVID-19) weather dependent? J Air Waste Manage Assoc. 2020;70(11):1061–4.
Selcuk M, Gormus S, Guven M. Impact of weather parameters and population density on the COVID-19 transmission: evidence from 81 provinces of Turkey. Earth Syst Environ. 2021;5(1):87–100.
Abraham J, Turville C, Dowling K, Florentine S. Does climate play any role in covid-19 spreading?—an Australian perspective. Int J Environ Res Public Health. 2021;18(17)
Sehra ST, Salciccioli JD, Wiebe DJ, Fundin S, Baker JF. Maximum daily temperature, precipitation, ultraviolet light, and rates of transmission of severe acute respiratory syndrome coronavirus 2 in the United States. Clin Infect Dis. 2020;71(9):2482–7.
CAS PubMed Google Scholar
Rubin D, Huang J, Fisher BT, Gasparrini A, Tam V, Song L, et al. Association of Social Distancing, population density, and temperature with the instantaneous reproduction number of SARS-CoV-2 in counties across the United States. JAMA Netw Open. 2020;3(7):1–12.
Comunian S, Dongo D, Milani C, Palestini P. Air pollution and covid-19: The role of particulate matter in the spread and increase of covid-19’s morbidity and mortality. Int J Environ Res Public Health. 2020;17(12):1–22.
Tosepu R, Gunawan J, Effendy DS, Ahmad LOAI, Lestari H, Bahar H, et al. Correlation between weather and Covid-19 pandemic in Jakarta, Indonesia. Sci Total Environ. 2020;725
Chen and Shakhnovich. Thermal adaptation of viruses and Bacteria. Biophys J. 2010;98:1109–18.
Martins LD, da Silva I, Batista WV, Andrade MF, Freitas ED, Jorge Alberto Martins JA. How socio-economic and atmospheric variables impact COVID-19 and influenza outbreaks in tropical and subtropical regions of Brazil. Environ Res. 2020;191:110184.
Kulkarni H, Khandait H, Narlawar UW, Rathod P, Mamtani M. Independent association of meteorological characteristics with initial spread of Covid-19 in India. Sci Total Environ. 2021;764:142801.
Menebo MM. Science of the Total environment temperature and precipitation associate with Covid-19 new daily cases : A correlation study between weather and Covid-19 pandemic in. Sci Total Environ. 2020;737:139659.
Gupta S, Patel KK. Global Epidemiology of First 90 Days into COVID-19 Pandemic :Disease Incidence , Prevalence , Case Fatality Population Density, Urbanisation. J Health Manag. 2020;22(2):117–28.
Haque SE, Rahman M. Association between temperature, humidity, and COVID-19 outbreaks in Bangladesh. Environ Sci Pol. 2020;114:253–5.
Sharma P, Singh AK, Agrawal B, Sharma A. Correlation between weather and COVID-19 pandemic in India: an empirical investigation. J Public Aff. 2020;20(4)
Fu S, Wang B, Zhou J, Xu X, Liu J, Ma Y, et al. Meteorological factors, governmental responses and COVID-19: evidence from four European countries. Environ Res. 2021;194:110596.
Mecenas P, Bastos RT, Vallinoto AC, Normando D. Effects of temperature and humidity on the spread of COVID-19: A systematic review. PLoS One. 2020;15:1–21.
Malki Z, Atlam ES, Hassanien AE, Dagnew G, Elhosseini MA, Gad I. Association between weather data and COVID-19 pandemic predicting mortality rate: machine learning approaches. Chaos, Solitons Fractals. 2020;138:110137.
Sasikumar K, Nath D, Nath R, Chen W. Impact of extreme hot climate on COVID-19 outbreak in India. GeoHealth. 2020;4(12)
Kodera S, Rashed EA, Hirata A. Correlation between COVID-19 morbidity and mortality rates in Japan and local population density, temperature, and absolute humidity. Int J Environ Res Public Health. 2020;17(15):1–14.
Sobral MFF, Duarte GB, da Penha Sobral AIG, Marinho MLM, de Souza Melo A. Association between climate variables and global transmission oF SARS-CoV-2. Sci Total Environ. 2020;729:138997.
Patel SK, Pathak M, Tiwari R, Yatoo MI, Malik YS. A vaccine is not too far for COVID-19 coronavirus pandemic A vaccine is not too far for COVID-19. May; 2020.
Nicola M, Neill NO, Sohrabi C, Khan M, Agha M, Agha R. Evidence based management guideline for the COVID-19 pandemic - review article. Int J Surg. 2020;77:206–16.
Atangana E, Atangana A. Facemasks simple but powerful weapons to protect against COVID-19 spread: can they have sides effects? Results in Physics. 2020;19:103425.
Sarmadi M, Moghanddam VK, Dickerson AS, Martelletti L. Association of COVID-19 distribution with air quality, sociodemographic factors, and comorbidities: an ecological study of US states. Air Qual Atmos Health. 2021;14(4):455–65.
Chung CJ, Nazif TM, Wolbinski M, Hakemi E, Lebehn M, Brandwein R, et al. The restructuring of structural heart disease practice during The Covid-19 pandemic. J Am Coll Cardiol. 2020; InPress
Bukhari Q, Massaro JM, D’agostino RB, Khan S. Effects of weather on coronavirus pandemic. Int J Environ Res Public Health. 2020;17(15):1–12.
Sun C, Zhai Z. The efficacy of social distance and ventilation effectiveness in preventing COVID-19 transmission. Sustain Cities Soc. 2020;62:102390.
Nair N, Selvaraj P. Using a cultural and social identity lens to understand pandemic responses in the US and India. Int J Cross-cult Manag. 2021;21(3):545–68.
Cetron M, Landwirth J. Public health and ethical considerations in planning for quarantine. Yale J Biol Med. 2005;78(5):325–30.
Jernigan DB. Update: public health response to the coronavirus disease 2019 outbreak—United States, February 24, 2020. MMWR. Morbidity and mortality weekly report, 69. 2020.
Stasi C, Fallani S, Voller F, Silvestri C. Treatment for COVID-19: an overview. Eur J Pharmacol. 2020;889:173644.
Foad CAKK, Xun N, Pejman J, Nataraj RC. Nonlinear dynamic analysis of an epidemiological model for COVID-19 including public behavior and government action. Nonlinear Dynamics. 2020;101(3):1545–59.
Sun Z, Zhang H, Yang Y, Wan H, Wang Y. Science of the Total environment impacts of geographic factors and population density on the COVID-19 spreading under the lockdown policies of China. Sci Total Environ. 2020;746(666):141347.
Abdullah S, Mansor AA, Napi NNLM, Mansor WNW, Ahmed AN, Ismail M, et al. Air quality status during 2020 Malaysia movement control order (MCO) due to 2019 novel coronavirus (2019-nCoV) pandemic. Sci Total Environ. 2020;729:139022.
Menhat M, Yusuf Y. Factors influencing the choice of performance measures for the oil and gas supply chain - exploratory study. IOP Conference Series: Materials Science and Engineering. 2018;342(1)
Ćirkovića MM, Vukotića B. Long-term prospects: mitigation of supernova and gamma-ray burst threat to intelligent beings. Acta Astronautica. 2016;129:438–46.
Asyary A, Veruswati M. Science of the Total environment sunlight exposure increased Covid-19 recovery rates : A study in the central pandemic area of Indonesia. Sci Total Environ. 2020;729:139016.
Jayaweeraa M, Pererab H, Gunawardanaa B, Manatungea J. Transmission of COVID-19 virus by droplets and aerosols: A critical review on the unresolved dichotomy. Environ Res. 2020;188:1–18.
Leung K, Shum MHH, Leung GM, Lam TTY, Wu JT. Early transmissibility assessment of the N501Y mutant strains of SARS-CoV-2 in the United Kingdom, October to November 2020. Euro Surveill. 2020;26(1)
Download references
Acknowledgements
The authors would also like to acknowledge the Editors and an anonymous reviewer, who contributed immensely to improving the quality of this publication and a special thanks to Muhammad Hafiy Nauwal Effi Helmy, that contributed an excellent idea through singing during the COVID-19 lockdown period.
This research did not receive any specific grant from funding agencies in the public, commercial, or not-for-profit sectors.
Author information
Authors and affiliations.
Faculty of Maritime Studies, Universiti Malaysia Terengganu, 21030, Kuala Nerus, Terengganu, Malaysia
Masha Menhat
Institute of Oceanography and Environment, Universiti Malaysia Terengganu, 21030, Kuala Nerus, Terengganu, Malaysia
Effi Helmy Ariffin, Junainah Zakaria & Mohd Fadzil Akhir
Faculty of Science and Marine Environment, Universiti Malaysia Terengganu, 21030, Kuala Nerus, Terengganu, Malaysia
Wan Shiao Dong & Aminah Ismailluddin
Institute for Social Science Studies, Universiti Putra Malaysia, 43400, Serdang, Selangor, Malaysia
Hayrol Azril Mohamed Shafril
Social, Environmental and Developmental Sustainability Research Center, Faculty of Social Sciences and Humanities, Universiti Kebangsaan Malaysia, 43600, Bangi, Selangor, Malaysia
Mahazan Muhammad
Institute of Geology Malaysia, Board of Geologists, 62100, Putrajaya, Malaysia
Ahmad Rosli Othman
Executive Office, Proofreading By A UK PhD, 51-1, Biz Avenue II, 63000, Cyberjaya, Malaysia
Thavamaran Kanesan
Faculty of Engineering, Universiti Malaya, 50603, Kuala Lumpur, Malaysia
Suzana Pil Ramli
Faculty of Applied Sciences, Uva Wellassa University, Badulla, 90000, Sri Lanka
Amila Sandaruwan Ratnayake
You can also search for this author in PubMed Google Scholar
Contributions
All authors have been involved in writing this editorial and contributing to the review of the manuscript. MM and EHA contribute to conceptualization. IA and ARO have made the figure.
Corresponding author
Correspondence to Effi Helmy Ariffin .
Ethics declarations
Ethics approval and consent to participate.
Not Applicable.
Consent for publication
Competing interests.
The authors declare that they have no competing interests.
Additional information
Publisher’s note.
Springer Nature remains neutral with regard to jurisdictional claims in published maps and institutional affiliations.
Rights and permissions
Open Access This article is licensed under a Creative Commons Attribution 4.0 International License, which permits use, sharing, adaptation, distribution and reproduction in any medium or format, as long as you give appropriate credit to the original author(s) and the source, provide a link to the Creative Commons licence, and indicate if changes were made. The images or other third party material in this article are included in the article's Creative Commons licence, unless indicated otherwise in a credit line to the material. If material is not included in the article's Creative Commons licence and your intended use is not permitted by statutory regulation or exceeds the permitted use, you will need to obtain permission directly from the copyright holder. To view a copy of this licence, visit http://creativecommons.org/licenses/by/4.0/ . The Creative Commons Public Domain Dedication waiver ( http://creativecommons.org/publicdomain/zero/1.0/ ) applies to the data made available in this article, unless otherwise stated in a credit line to the data.
Reprints and permissions
About this article
Cite this article.
Menhat, M., Ariffin, E.H., Dong, W.S. et al. Rain, rain, go away, come again another day: do climate variations enhance the spread of COVID-19?. Global Health 20 , 43 (2024). https://doi.org/10.1186/s12992-024-01044-w
Download citation
Received : 27 July 2023
Accepted : 22 April 2024
Published : 14 May 2024
DOI : https://doi.org/10.1186/s12992-024-01044-w
Share this article
Anyone you share the following link with will be able to read this content:
Sorry, a shareable link is not currently available for this article.
Provided by the Springer Nature SharedIt content-sharing initiative
- Coronavirus
- Solar radiation
- Temperature
- Social distancing
Globalization and Health
ISSN: 1744-8603
- Submission enquiries: [email protected]
- General enquiries: [email protected]

Rare health condition cases surge during COVID-19 pandemic
A rare condition surged among previously unaffected groups of people during the COVID-19 pandemic and led to the deaths of nine patients, researchers say.
The condition is a type of autoimmune response linked to the body immune system mistakenly attacking itself. Intriguingly, the system being attacked normally works as a sentinel or guard, whose job it is to detect invading viruses. It is mainly seen in East Asian women and girls and was very uncommon in the UK. But researchers at the University of Leeds and Leeds Teaching Hospitals NHS trust, who were examining data on MDA5 autoimmunity in Yorkshire during the pandemic, noted an unprecedented 60 cases among mainly white men and women.
Researchers investigating the increase in cases believe that exposure to the COVID-19 virus may trigger the condition, which causes distinctive skin rashes, pneumonia and interstitial lung disease, a rapidly progressing lung inflammation with damage which is often fatal.
Principal Investigator Dennis McGonagle, Professor of Investigative Rheumatology in the University of Leeds' School of Medicine and Leeds Teaching Hospitals NHS Trust, said: "Whilst we very occasionally see this disease in the UK, this surge in cases was completely new and very different.
"It is important that physicians understand the symptoms so that patients can be quickly referred for treatment and have the best chance of a rapid and full recovery. Lives could undoubtedly be saved but there is a great need for research to try and slow or stop the rapid lung progression that occurs in some patients."
The research paper is published in The Lancet eBioMedicine .
Viruses and autoimmune response
The immune system contains a protein called MDA5, which helps detect RNA viruses like COVID-19. Normally, this protein helps trigger an immune response in the body, where more of the protein is produced to help fight off the virus. However, sometimes the immune system releases antibodies which mistakenly attack this protein, leading to MDA5 autoimmune diseases such as the rare disease described in this study. The exact cause is not well understood, but scientists believe the virus itself could trigger the response.
Autoimmune diseases seen after viral infections have similar features, such as fatigue, joint pain and swelling, skin rashes and digestive issues. During the COVID-19 pandemic, physicians saw a rare condition in children called multisystem inflammatory syndrome in children (MIS-C) where there was no evidence of active viral infection of the lungs. This MIS-C syndrome affected multiple systems in the body, including the heart, kidneys, brain, skin, eyes, and digestive organs, but usually spared the lungs. The disease seen in Yorkshire was dubbed MIP-C (pronounced "mipsy") because of its similarities to MIS-C in that it occurred around the time of the pandemic but where active infection was usually absent.
The 60 patients in the study presented at their GPs or to A&E with a range of symptoms including shortness of breath, muscle pain, rashes, and reduced blood flow to the fingers, known as Raynaud's disease. These symptoms are associated with autoimmune disease.
The patients were referred to rheumatology specialists for further testing and all were diagnosed with the condition. Of the 60 patients, 35 had received COVID-19 vaccinations, and 15 had previously tested positive for COVID-19. However, patients were not systematically tested for COVID-19, and some may have been infected, but were asymptomatic at the time. Such cases could have developed MDA5 disease due to an overreaction of their immune system to minimal exposure to the virus.
Twenty-five out of the 60 patients (41.7%) developed interstitial lung disease, and despite treatment with immunosuppressant medication, eight of those patients died. A ninth patient, who did not have interstitial lung disease, died from sepsis.
To understand the increase in cases of this rare condition, researchers collected data on the number of these tests between January 2018 and December 2022, alongside data on COVID-19 infections and vaccination, and information about each patient's symptoms. Analysis showed that just six cases of the rare condition had been diagnosed between 2018 and 2019. However, in the 3 years after 60 new cases appeared, eight cases were diagnosed in 2020; 35 cases were diagnosed in 2021 and 17 cases were diagnosed in 2022. Very few cases have occurred since then.
Patient demographics
Patients were aged between 43 and 71 years, with 36 of them being female.
- South Asian: 3
- Black Caribbean: 2
- Black African: 1
- Any other ethnic group: 4
- Asian (not Chinese): 4
- There was no ethnicity data for 14 patients.
Researchers noted that there was a strong overlap in 2021 between vaccination rates in Yorkshire and the surge in MDA5 disease cases. This peak, though, also occurred shortly after a community wave of coronavirus infection occurred in late 2021. A smaller overlap was seen between confirmed COVID-19 infection and MDA5. However, it was interesting to note that almost half (42%) of patients were not documented to have been vaccinated against COVID-19 before they tested positive for MDA5. Four children who tested positive for MDA5 were unvaccinated, pointing to the idea of an overreacting immune response after exposure to a virus.
Professor McGonagle said: "We know that vaccines can trigger an immune overreaction, but given that not all of these patients were vaccinated against COVID-19, and the increase in cases occurred when the COVID-19 virus was circulating in significant numbers, the evidence strongly suggests that the increase in cases of this rare disease is linked to exposure to the virus."
The data was generated by Dr Paula David and Dr Gabriele DeMarco from the University of Leeds' School of Medicine and the Leeds Teaching Hospitals NHS Trust, with their Trust colleague Dr Khizer Iqbal. This work was done in collaboration with Dr Pradipta Ghosh and her team at the University of California, San Diego, who showed that the MDA5 protein was found in higher amounts in patients with COVID-19 and in patients with other diseases involving MDA5. They discovered that this increase was linked to an abnormal immune response that slows the virus's ability to multiply and spread. Additionally, they found that higher levels of the MDA5 protein were associated with increased levels of Interleukin-15, a protein that activates T-cells, or immune cells. This interaction could be contributing to the autoimmune response.
The investigators say that doctors should be aware of their findings so that patients can be more quickly diagnosed and referred for treatment, which could help lessen lung damage and save lives. This is especially so in subjects with suspected pneumonia not getting better on therapy and where the rashes, muscle involvement and other disease features are absent.
Dr Paula David, the first author of the paper, said: "We think that this large, unprecedented outbreak of MDA5 disease in Yorkshire will help advance the field to better appreciate the role between viruses and autoimmunity."
Dr Saptarshi Sinha, the co-first author of the paper and the interim director of PreCSN, added: "Here at PreCSN, we enjoy the ability to help researchers such as the McGonagle group dive into big data and find patterns rapidly with precision that allows us to connect the dots. In this case, we are glad we could find a connection between a clinical presentation of autoimmune disease in the backdrop of COVID-19 at a molecular level."
- Diseases and Conditions
- Immune System
- Infectious Diseases
- COVID and SARS
- Today's Healthcare
- Lung Disease
- Immune system
- West Nile virus
- Rheumatoid arthritis
- Urinary incontinence
Story Source:
Materials provided by University of Leeds . Note: Content may be edited for style and length.
Journal Reference :
- Paula David, Saptarshi Sinha, Khizer Iqbal, Gabriele De Marco, Sahar Taheri, Ella McLaren, Sheetal Maisuria, Gururaj Arumugakani, Zoe Ash, Catrin Buckley, Lauren Coles, Chamila Hettiarachchi, Emma Payne, Sinisa Savic, Gayle Smithson, Maria Slade, Rahul Shah, Helena Marzo-Ortega, Mansoor Keen, Catherine Lawson, Joanna Mclorinan, Sharmin Nizam, Hanu Reddy, Omer Sharif, Shabina Sultan, Gui Tran, Mark Wood, Samuel Wood, Pradipta Ghosh, Dennis McGonagle. MDA5-autoimmunity and interstitial pneumonitis contemporaneous with the COVID-19 pandemic (MIP-C) . eBioMedicine , 2024; 104: 105136 DOI: 10.1016/j.ebiom.2024.105136
Cite This Page :
Explore More
- Future Climate Impacts Put Whale Diet at Risk
- Charge Your Laptop in a Minute?
- Caterpillars Detect Predators by Electricity
- 'Electronic Spider Silk' Printed On Human Skin
- Engineered Surfaces Made to Shed Heat
- Innovative Material for Sustainable Building
- Human Brain: New Gene Transcripts
- Epstein-Barr Virus and Resulting Diseases
- Origins of the Proton's Spin
- Symbiotic Bacteria Communicate With Plants
Trending Topics
Strange & offbeat.
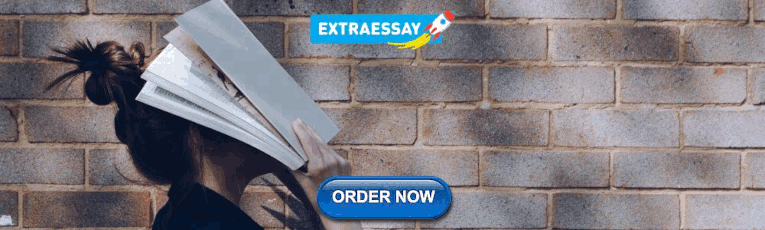
IMAGES
COMMENTS
The spread of the "Severe Acute Respiratory Coronavirus 2" (SARS-CoV-2), the causal agent of COVID-19, was characterized as a pandemic by the World Health Organization (WHO) in March 2020 and has triggered an international public health emergency [].The numbers of confirmed cases and deaths due to COVID-19 are rapidly escalating, counting in millions [], causing massive economic strain ...
Abstract. In early December 2019, an outbreak of coronavirus disease 2019 (COVID-19), caused by a novel severe acute respiratory syndrome coronavirus 2 (SARS-CoV-2), occurred in Wuhan City, Hubei Province, China. On January 30, 2020 the World Health Organization declared the outbreak as a Public Health Emergency of International Concern.
Coronavirus disease 2019 (COVID-19) is a highly contagious viral illness caused by severe acute respiratory syndrome coronavirus 2 (SARS-CoV-2). COVID-19 has had a catastrophic effect on the world, resulting in more than 6 million deaths worldwide. After the first cases of this predominantly respiratory viral illness were reported in Wuhan, Hubei Province, China, in late December 2019, SARS ...
Abstract. In early December 2019, an outbreak of coronavirus disease 2019 (COVID-19), caused by a novel severe acute respiratory syndrome coronavirus 2 (SARS-CoV-2), occurred in Wuhan City, Hubei Province, China. On January 30, 2020 the World Health Organization declared the outbreak as a Public Health Emergency of International Concern.
These papers highlight valuable research into the biology of coronavirus infection, its detection, treatment as well as into vaccine development and the epidemiology of the disease. Browse all Top ...
Long-term effects of coronavirus disease 2019 (COVID-19). The meta-analysis of the studies included an estimate for one symptom or more reported that 80% of the patients with COVID-19 have long ...
The WHO Covid-19 Research Database was maintained by the WHO Library & Digital Information Networks and was funded by COVID-19 emergency funds. The database was built by BIREME, the Specialized Center of PAHO/AMRO. Its content spanned the time period March 2020 to June 2023. It has now been archived, and no longer searchable since January 2024.
COVID research: a year of scientific milestones. Nature waded through the literature on the coronavirus — and summarized key papers as they appeared. A cell infected with particles (yellow ...
Given the urgency of the COVID-19 epidemic and the need to understand and access information about it, a scoping review was considered suitable for the situation. We therefore conducted this scoping review to help identify research gaps related to this new viral disease and propose recommendations for future research on COVID-19.
Across the world, there are multiple variants of severe acute respiratory syndrome coronavirus 2 (SARS-CoV-2), the virus that causes coronavirus disease 2019 (Covid-19).
Key Events in the Effort to Determine the Origins of the Covid-19 Pandemic. The joint WHO-China technical report published in March 2021 rated a zoonotic spillover as a "likely to very likely ...
Coronavirus (COVID-19) research. Medical, social, and behavioral science articles from Sage Sage believes in the power of the social and behavioral sciences to convert the best medical research into policies, practices, and procedures to improve - and even save - lives. This collection includes the latest medical research from Sage related ...
### What you need to know Since the emergence of SARS-CoV-2 in December 2019, there has been an unparalleled global effort to characterise the virus and the clinical course of disease. Coronavirus disease 2019 (covid-19), caused by SARS-CoV-2, follows a biphasic pattern of illness that likely results from the combination of an early viral response phase and an inflammatory second phase. Most ...
A two-dose regimen of BNT162b2 (30 μg per dose, given 21 days apart) was found to be safe and 95% effective against Covid-19. The vaccine met both primary efficacy end points, with more than a 99 ...
Abstract. Since its emergence in December 2019, corona virus disease 2019 (COVID-19) has impacted several countries, affecting more than 90 thousand patients and making it a global public threat. The routes of transmission are direct contact, and droplet and possible aerosol transmissions. Due to the unique nature of dentistry, most dental ...
To support research to combat the COVID-19 outbreak, Nature Research have opened a collection covering the biology of coronavirus infection, its detection, treatment and evolution, research into the epidemiology of emerging viral diseases, and coverage of current events. In response to the COVID-19 pandemic, Springer have made corona-related ...
The COVID-19 pandemic has had a severe impact on the mental health and wellbeing of people around the world while also raising concerns of increased suicidal behaviour. In addition access to mental health services has been severely impeded. However, no comprehensive summary of the current data on these impacts has until now been made widely ...
Complications in critically ill COVID-19 patients. One of the most common in-hospital complications seen in critically ill COVID-19 patients is barotrauma which can be in the form of a pneumothorax, pneumomediastinum or subcutaneous emphysema [Citation 2].Barotrauma has an incidence that ranges from 7.4 to 40% in patients requiring invasive mechanical ventilation (IMV), and between 4.7 and 8.1 ...
In May 2023, the United States ended the federal COVID-19 Public Health Emergency (PHE) with more than 1.1 million reported deaths. 1 American Indian or Alaska Native and Native Hawaiian or Pacific Islander individuals represent numerically small populations (constituting 1.3% and 0.3% of the total US population, 2 respectively). Their high mortality rates have minimal impact on national rates ...
In recent years, pandemics caused by pathogenic microorganisms, including the novel coronavirus (COVID-19), Zika virus, dengue fever, and Ebola virus (EBOV), have caused significant disruption and damage upon human societies [1,2].Data from China and Italy suggest a case-fatality rate of 2.3% in patients with COVID-19, with Western Europe and the US being particularly severely affected [].
The clinical features of COVID-19 are varied, ranging from asymptomatic state to acute respiratory distress syndrome and multi organ dysfunction. The common clinical features include fever (not in all), cough, sore throat, headache, fatigue, headache, myalgia and breathlessness. Conjunctivitis has also been described.
Please use one of the following formats to cite this article in your essay, paper or report: APA. Sai Lomte, Tarun. (2024, May 23). Study confirms face masks' effectiveness in reducing disease ...
As reported by the CDC, from February 12 to April 2, 2020, of 149,760 cases of confirmed COVID-19 in the United States, 2572 (1.7%) were children aged <18 years, similar to published rates in ...
The COVID-19 pandemic forced governments, multilateral public health organisations and research institutions to undertake research quickly to inform their responses to the pandemic. Most COVID-19-related studies required swift approval, creating ethical and practical challenges for regulatory authorities and researchers. In this paper, we examine the landscape of ethics review processes in ...
New research shows that ferroptosis, a form of cell death, occurs in severe COVID-19 patient lungs. Stopping it improves outcomes. In some severe cases of COVID-19, the lungs undergo extreme ...
The spread of infectious diseases was further promoted due to busy cities, increased travel, and climate change, which led to outbreaks, epidemics, and even pandemics. The world experienced the severity of the 125 nm virus called the coronavirus disease 2019 (COVID-19), a pandemic declared by the World Health Organization (WHO) in 2019. Many investigations revealed a strong correlation between ...
Date: May 23, 2024. Source: University of Leeds. Summary: A rare condition surged among previously unaffected groups of people during the COVID-19 pandemic and led to the deaths of nine patients ...
Dec 27, 2023. CME. R.T. Gandhi and Others. A 70-year-old woman with advanced HIV infection was evaluated because of cough, shortness of breath, and malaise. Eleven months earlier, she had received ...
Abstract. Background: Accurate diagnosis and management of patients with rapidly progressive dementia may be challenging during the COVID-19 pandemic, which has negatively influenced the diagnostic performances, medical resource allocation and routine care for all non-COVID-19 diseases.Case presentation: We herein present a case of a 57‐year‐old male with rapidly progressive cognitive ...
The COVID-19 pandemic, also known as the coronavirus pandemic, is a global pandemic of coronavirus disease 2019 (COVID-19) caused by severe acute respiratory syndrome coronavirus 2 (SARS-CoV-2). The novel virus was first identified in an outbreak in Wuhan , the capital of Hubei , China, in December 2019, before it spread to other areas of Asia ...