An official website of the United States government
Official websites use .gov A .gov website belongs to an official government organization in the United States.
Secure .gov websites use HTTPS A lock ( Lock Locked padlock icon ) or https:// means you've safely connected to the .gov website. Share sensitive information only on official, secure websites.
- Publications
- Account settings
- Advanced Search
- Journal List

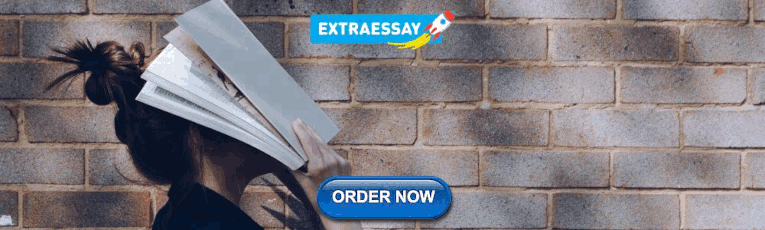
Stress research during the COVID-19 pandemic and beyond
Lena sophie pfeifer, katrin heyers, sebastian ocklenburg, oliver t wolf.
- Author information
- Article notes
- Copyright and License information
Corresponding author at: Lena Sophie Pfeifer (Cognitive Psychology, Ruhr University Bochum, Universitätsstraße 150, Room: IB 6/77, 44780, Bochum, Germany).
Shared senior-authorship.
Received 2021 Aug 5; Revised 2021 Sep 22; Accepted 2021 Sep 24; Issue date 2021 Dec.
Since January 2020 Elsevier has created a COVID-19 resource centre with free information in English and Mandarin on the novel coronavirus COVID-19. The COVID-19 resource centre is hosted on Elsevier Connect, the company's public news and information website. Elsevier hereby grants permission to make all its COVID-19-related research that is available on the COVID-19 resource centre - including this research content - immediately available in PubMed Central and other publicly funded repositories, such as the WHO COVID database with rights for unrestricted research re-use and analyses in any form or by any means with acknowledgement of the original source. These permissions are granted for free by Elsevier for as long as the COVID-19 resource centre remains active.
Graphical abstract
Schematic representation of challenges and perspectives for stress research during the COVID-19 pandemic. Stress researchers are forced to adapt established experimental procedures to current environmental demands or to develop new ones. Technologies that help adjust stress research to requirements of the COVID-19 pandemic, however, are not only temporary solutions but bear the potential to advance stress research far beyond the current situation. They give new perspectives to stress researchers by allowing research in new contexts, on special samples, and with new experimental variations. Created with BioRender.com.
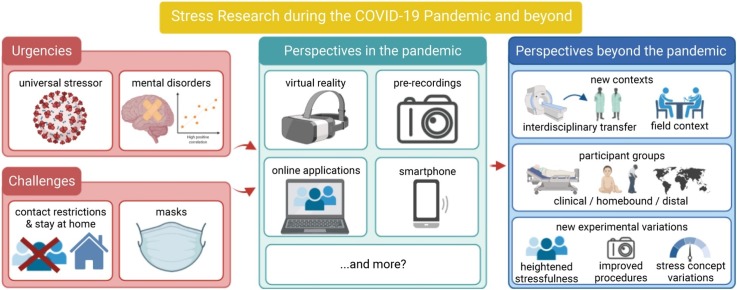
Keywords: Stress research, COVID-19, Corona virus, Social-evaluative threat, Trier social stress test (TSST), Online studies, Ecological field research, Chronic stress, Virtual reality, Stress-related mental disorders
The COVID-19 pandemic confronts stress researchers in psychology and neuroscience with unique challenges. Widely used experimental paradigms such as the Trier Social Stress Test feature physical social encounters to induce stress by means of social-evaluative threat. As lockdowns and contact restrictions currently prevent in-person meetings, established stress induction paradigms are often difficult to use. Despite these challenges, stress research is of pivotal importance as the pandemic will likely increase the prevalence of stress-related mental disorders. Therefore, we review recent research trends like virtual reality, pre-recordings and online adaptations regarding their usefulness for established stress induction paradigms. Such approaches are not only crucial for stress research during COVID-19 but will likely stimulate the field far beyond the pandemic. They may facilitate research in new contexts and in homebound or movement-restricted participant groups. Moreover, they allow for new experimental variations that may advance procedures as well as the conceptualization of stress itself. While posing challenges for stress researchers undeniably, the COVID-19 pandemic may evolve into a driving force for progress eventually.
1. Introduction
Feeling stressed is familiar to all of us as in our everyday life we encounter numerous situations that may be perceived as stressful (e.g., having to give an oral presentation at work, acting under time pressure, or facing the next exam). Stress is often defined as a state in which external demands exceed internal resources, causing the organism to initiate a neuroendocrine stress response ( Lazarus, 1993 ). First, this leads to activation of the sympathetic nervous system (SNS) which causes catecholamines such as adrenaline and noradrenaline to be secreted by the adrenal medulla and physiological parameters such as heart rate, blood pressure, or sweating to increase ( Goldstein, 1987 ; Joëls and Baram, 2009 ; Mason, 1968 ). Second, the hypothalamus-pituitary-adrenal cortex (HPA) axis is activated ( Aguilera, 2011 ). This initiates a hormonal cascade which terminates in the release of glucocorticoids such as cortisol that target cells across the whole body ( de Kloet et al., 2005 ). Glucocorticoids have been shown to affect various cognitive processes like decision-making ( Shields et al., 2016 ; Starcke and Brand, 2016 ), memory ( Wolf, 2009 ), or extinction learning and its relapse ( Meir Drexler et al., 2019 ). The induced changes are assumed to help the organism to meet current environmental demands. The acute stress response is hence considered an adaptive coping mechanism. However, when stress is experienced too strongly or too frequently, it is likely to harm the organism ( Epel et al., 2018 ; McEwen, 1998 ). While substantial progress has been made during the last decades, the exact mechanisms and the complex interactions between genetic and environmental risk factors across the life span are still insufficiently understood ( Ehlert et al., 2001 ; Zänkert et al., 2019 ).
The ongoing COVID-19 pandemic can be characterized as a universal and chronic stressor affecting people worldwide and across all sections of society. Hence, it has the potential to cause a public mental health crisis of unprecedented dimensions ( Pfefferbaum and North, 2020 ). Taking into account the ongoing increase in the prevalence of mental health problems ( Baxter et al., 2014 ; Cohen and Janicki-Deverts, 2012 ; DeVries and Wilkerson, 2003 ), this review article (1) outlines the urgent need to conduct experimental stress research using standardized stressors during the current COVID-19 pandemic; (2) discusses conceptual as well as methodological challenges the discipline is confronted with in this peculiar situation; and (3) reviews trends, perspectives, and technological advances in order to solve the conflict between the urgent need and the procedural challenges for stress research during the COVID-19 pandemic and beyond.
2. The need for stress research during the current COVID-19 pandemic
In recent years, the prevalence of stress-related mental disorders has been following an upward trend ( Baxter et al., 2014 ; Cohen and Janicki-Deverts, 2012 ; DeVries and Wilkerson, 2003 ), causing both individual burden and financial and social problems for society as a whole ( Hassard et al., 2018 ; Trautmann et al., 2016 ). In general, stress has been discussed as a crucial factor in etiological diathesis-stress models of mental disorders ( Cohen et al., 2016 ; Cohen et al., 2007 ; Ottenweller et al., 1989 ) such as posttraumatic stress disorder (PTSD; Yehuda, 2002 ) and anxiety disorders ( Sandín and Chorot, 1993 ; Shin and Liberzon, 2010 ), depression ( Colodro-Conde et al., 2018 ; Musliner et al., 2015 ), or neurodevelopmental ( Gunnar and Quevedo, 2007 ; Huizink et al., 2003 ) and psychotic disorders ( Thompson et al., 2007 ).
Assuming that stress is of crucial relevance in the development of these mental disorders, it must be emphasized that the COVID-19 pandemic may function as an additional stressor. This hypothesis is based on the fact that the pandemic is novel, unpredictable, and uncontrollable (three key features of stressors) ( Mason, 1968 ). Moreover, the political measures imposed to prevent the virus from further spreading may also be perceived as stressful. The latter has been confirmed by public surveys ( Groarke et al., 2020 ; Kowal et al., 2020 ; Qiu et al., 2020 ). In particular, many individuals express concerns about COVID-19 infections, altered everyday life routines, and great uncertainty regarding future developments ( Amirkhan, 2021 ; Hagger et al., 2020 ; Mahmud et al., 2021 ). Among others, health care workers ( Bohlken et al., 2020 ; Chew et al., 2020 ; Spoorthy et al., 2020 ) and individuals who have lost their jobs or face financial problems due to the pandemic report increased levels of stress ( Achdut and Refaeli, 2020 ; Blustein et al., 2020 ). Similarly, people who experience loneliness on account of strict contact restrictions ( Brooks et al., 2020 ; Groarke et al., 2020 ) as well as parents struggling with childcare with schools and kindergartens being closed ( Brown et al., 2020 ) were revealed to be under extreme stress. Therefore, the COVID-19 pandemic, due to being chronic and universal, must be considered a unique stressor that will have severe implications for health and wellbeing.
2.1. Epidemiological and clinical stress research
Recent studies have revealed heightened levels of anxiety and depression during the ongoing pandemic, with specific factors such as social or lower economic resources influencing stress perception ( Bueno-Notivol et al., 2021 ; Ettman et al., 2020 ; Kowal et al., 2020 ). Holman et al. (2020) , at an early but critical phase of the unfolding pandemic in the U.S., used a probability-based approach in a nationally representative sample to predict the progression of mental disorders. The authors reported that (1) individuals with pre-existing health problems, (2) individuals exposed to secondary stressors, and (3) individuals with increased exposure to COVID-19 related media coverage are particularly at risk for adverse mental health outcomes. Along these lines, Boyraz and Legros (2020) and Bridgland et al. (2021) highlight that the pandemic may even constitute a traumatic experience which could increase the prevalence of PTSD.
In the past, events such as 9/11 ( Laugharne et al., 2007 ; Lowell et al., 2018 ; Neria et al., 2011 ; Yehuda, 2002 ), school shootings ( Rossin-Slater et al., 2020 ), or natural disasters such as earthquakes ( Garfin et al., 2014 ) or hurricanes ( Ironson et al., 1997 ) have caused surges in mental health problems. The Great Recession in 2007–2009 led to an increase in mental health issues ( Margerison-Zilko et al., 2016 ). For pandemics, data on previous epidemics such as the Middle East Respiratory Syndrome (MERS; e.g., Jeong et al., 2016 ) or the Severe Acute Respiratory Syndrome (SARS; e.g., Chan et al., 2006 ) reveal an increase in mental health problems. Based on these findings, one can conclude that the COVID-19 pandemic as a universal and long-lasting stressor will have comparably devastating consequences. Kickbusch et al. (2020) even call it “[….] the biggest threat in living memory to health and wellbeing, social welfare, and the global economy”, implying that it is of greater destructive power than every former crisis of the more recent past. To illustrate the implications of the COVID-19 pandemic on society and research, Fig. 1 provides a schematic diagram of stressors encountered in laboratory contexts and in everyday life, along with their relevance for basic, clinical, and epidemiological stress research.
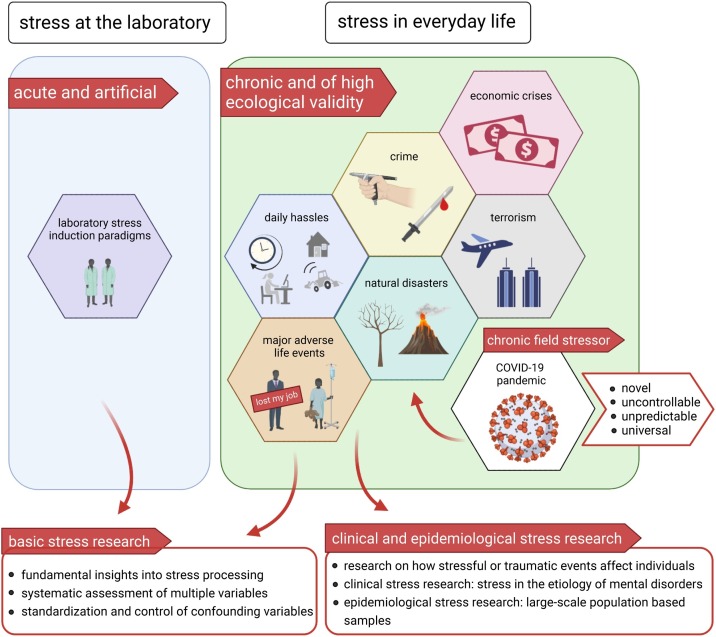
Schematic illustration of how stress can be induced in the laboratory or experienced in everyday life, and of their relevance for basic and clinical or epidemiological stress research. In the laboratory, researchers make use of standardized stress induction paradigms that are acute and well-controlled, but also rather artificial. In everyday life, we are confronted with various stressors that are rather chronic and of interest for basic as well as clinical and epidemiological stress research. Basic stress research acknowledges field stressors because of their high ecological validity. Therefore, some events of stressful or traumatic nature have already been subject of stress research. The COVID-19 pandemic can be considered a chronic field stressor that bears a potential for clinical and epidemiological as well as for basic stress research. Created with BioRender.com.
Other approaches, however, reverse the causality and suggest that stress, or more specifically stress overload, serves to explain an unsolved variance in the actual spread of COVID-19. Given that infections were distributed unequally over different countries and social classes, Amirkhan (2021) , for example, hypothesized stress to increase the individual susceptibility for COVID-19 infection. In line with this, evidence has been accumulating on chronic stress impairing immune function thereby increasing the risk for and the severity and duration of infectious diseases or other illness episodes ( Glaser and Kiecolt-Glaser, 2005 ).
To conclude, various lines of research confirm a relation between stress and adverse health outcomes under conditions of COVID-19, highlighting the urgent need to consider the pandemic a public health priority ( Ettman et al., 2020 ; Pfefferbaum and North, 2020 ). This also entails high societal relevance of stress research under these extraordinary circumstances. On the one hand, epidemiological and clinical perspectives are needed to understand the impact of the COVID-19 pandemic ( Daly and Robinson, 2021 ; Ettman et al., 2020 ; Khan et al., 2020 ). On the other hand, basic stress research is of special relevance in the current pandemic, as will be outlined below.
2.2. Basic stress research
In addition to epidemiological and clinical stress research during the pandemic, we argue that basic stress research can provide fundamental insights that are crucial for clinical applications. In this context, basic research already yielded studies on acute laboratory stress that had direct or indirect consequences for clinical practice. For example, Pruessner et al. (1997) and Kirschbaum et al. (1995) provided first evidence that cortisol response kinetics characterized by lacking habituation towards repeated stress exposure might represent a marker for health. Buske-Kirschbaum et al. (2003) and Buske-Kirschbaum et al. (2010) supported this idea by showing blunted cortisol responsiveness in atopic diseases. Finally, such findings can be integrated in theoretical considerations on allostatic load introduced by McEwen (1998) .
Along these lines, clinical approaches to stress during COVID-19 should be paralleled by basic research integrating relevant information into a general consent on fundamental mechanisms. Clinical conditions typically manifest progressively, often starting with rather subtle symptoms ( Myin-Germeys et al., 2009 ; van Os et al., 2009 ). Considering psychopathological outcomes related to COVID-19 to be an extreme on a wider continuum, it would be simplistic to only focus on people with manifest clinical symptoms. Instead, it seems conceivable that even individuals not meeting diagnostic criteria may have undergone subclinical and latent changes ( Khan et al., 2020 ). These may be caused by stress-related changes in brain function and brain structure. In this context, previous works has suggested associations between chronic stress and reduced volume in prefrontal and limbic regions ( Ansell et al., 2012 ; Berretz et al., 2021 ) and altered functional connectivity within frontoparietal brain circuits ( Liston et al., 2009 ).
Salomon et al. (2020) investigated stress-related brain plasticity at an early stage of the pandemic in Israel using magnet resonance imaging (MRI). The authors scanned healthy participants before and after the outbreak of the pandemic and analyzed volumetric alterations in the brain relative to control participants scanned twice under pre-pandemic conditions. Salomon et al. (2020) found volumetric increases in brain regions implicated in the neural circuits of stress and anxiety. Importantly, these findings confirm that the COVID-19 pandemic is capable of inducing changes on a neural level not only for those who were actually infected with the virus ( Crunfli et al., 2020 ; Lu et al., 2020 ).
Pandemic-related changes might also manifest as slight changes of habits on a behavioral level. For instance, alcohol consumption has been shown to have increased in some countries ( Calina et al., 2021 ; Ingram et al., 2020 ). Such maladaptive ways of coping with stress can themselves adversely affect individual health and increase the risk for contracting COVID-19 and/or developing other physical or mental illnesses ( Gouin, 2011 ).
By trying to unravel subtle as well as severe alterations caused by adverse conditions during the COVID-19 pandemic, basic stress research can complement epidemiological or clinical approaches. Assuming that stress is a significant factor in the ontogenesis of mental disorders, its effects are likely mediated by physiological or endocrine processes of the HPA axis or the SNS ( Ehlert et al., 2001 ; Zänkert et al., 2019 ). Specific pathways, however, are rarely assessed in large-scale population studies as it is costly and laborious to collect physiological markers such as saliva samples from larger cohorts ( Adam and Kumari, 2009 ; Friedman et al., 1988 ). Basic stress research can close this gap because it can assess multiple physiological and neural stress markers. A combination of comprehensive assessment of the stress response and the fundamental strengths of laboratory research (e.g., control of confounding variables, standardization of experimental procedures) has been successful in identifying sources of intra- and interindividual variability ( Ehlert et al., 2001 ; Zänkert et al., 2019 ). Taking these factors into account may clarify how and why individuals differ in their vulnerability to stress-related health problems ( Epel et al., 2018 ; McEwen, 1998 ).
With regard to how COVID-19 related basic stress research may enrichen our general understanding of human stress processing, it is still debated whether chronic levels of increased stress affect acute stress processing ( Kudielka and Wüst, 2010 ; Lam et al., 2019 ; Matthews et al., 2001 ). A growing body of literature indicates that chronic or cumulative stress leads to insensitivity towards acute stressors as reflected in a dampened stress response ( Fries et al., 2005 ; Lam et al., 2019 ; Matthews et al., 2001 ; Sandner et al., 2020 ). However, systematic studies on this matter are as yet sparse, as it is ethically not justified to expose participants to chronic stress and thereby put them at risk for poor mental health outcomes for research purposes only.
The issue of chronic stress was extensively discussed by McEwen (1998) . In the laboratory, he found evidence for four scenarios in which the allostatic response that is adaptive under normal conditions is initiated (1) too often, (2) too long, (3) not at all, or (4) lacks environmental adaptation. Assuming that the COVID-19 pandemic may force the organism to show one or more of these maladaptive response patterns, it can be considered a unique opportunity to advance our general knowledge of chronic stress.
Similarly, everyday life typically confronts us with stressors that persist over longer periods of time and lack a clear beginning or ending ( Epel et al., 2018 ). Therefore, naturalistic stressors resemble those conditions leading to allostatic load as suggested by McEwen (1998) . Investigating chronic stress is thus of higher ecological validity than solely focusing on its acute counterpart. Such ecological validity would further be enhanced by the fact that COVID-19 constitutes a real-world field stressor that is personally relevant for the designated individual ( Rohleder et al., 2007 ). As under normal conditions, basic stress researchers are restricted to experimental stress induction that is rather artificial in nature, it has often been questioned to what extent laboratory findings expand to real world scenarios ( Johnston et al., 2008 ; Rohleder et al., 2007 ; Turner et al., 1990 ; van Doornen and van Blokland, 1992 ). Taken together, the COVID-19 pandemic can be considered an excellent opportunity to assess stress in a field context.
Some researchers have already recognized this potential and operationalized the COVID-19 pandemic as an independent variable in ongoing experiments. For example, Somma et al. (2021) had collected data from a range of behavioral tests assessing asymmetry of visual-spatial attention in a larger sample right before COVID-19 quarantine in Italy. They repeated their measurement in a subsample several months later and demonstrated that quarantine further lateralized their participants’ performance outcomes. This shows that the COVID-19 pandemic had a significant influence on visual-spatial information processing. Further studies could clarify in how far it also affected other cognitive and behavioral domains and how putative alterations could relate to mental health and wellbeing in general.
Despite its high ecological validity, the COVID-pandemic may not meet all quality criteria for experimental research. In particular, the pandemic as well as other field stressors do not represent uniformly standardized stressors and thus bear many confounding variables ( Amirkhan, 2021 ; Bueno-Notivol et al., 2021 ; Kowal et al., 2020 ). Therefore, stress researchers are still reliant on laboratory stress induction. Decades of well-controlled laboratory research have led to the development of highly reliable experimental paradigms for stress induction, such as the Trier Social Stress Test (TSST; Allen et al., 2017 ; Dickerson and Kemeny, 2004 ; Goodman et al., 2017 ; Kirschbaum et al., 1993 ). Unfortunately, it is these well-established protocols that cause stress researchers to face huge challenges during the COVID-19 pandemic.
3. Challenges for stress research during the current COVID-19 pandemic
Most laboratory stress research paradigms designed for human participants rely on exposing them to situations containing elements of social-evaluative threat. In comparison to other features of a stressor, the social-evaluative component is considered particularly crucial ( Dickerson and Kemeny, 2004 ). Its importance is explained by the Social Self Preservation Theory ( Dickerson et al., 2004 ; Kemeny et al., 2004 ). According to this theory, humans (as well as primates and other animals) do not only strive to preserve their physical wellbeing but also their social self and their social status. Any threat to one’s social integrity is registered by the so-called social self-preservation system which provokes specific biological responses to combat the threat. These biological responses are suggested to be supported by the HPA axis ( Dickerson et al., 2004 ; Dickerson and Kemeny, 2004 ; Kemeny et al., 2004 ). Given that in our everyday life, stress is mostly experienced in social contexts ( von Dawans et al., 2021 ), social-evaluative threat accounts for a substantial portion of the ecological validity of laboratory stress induction paradigms.
For this reason, most commonly used stress induction paradigms include some sort of social-evaluative threat, which is per definition most effectively produced in human interactions such as in-person social encounters. For example, in the TSST, participants must perform a mock job interview and solve challenging arithmetic tasks while being judged by a panel of reviewers who do not provide any feedback ( Kirschbaum et al., 1993 ). As the TSST has been shown to reliably produce significant release of cortisol ( Kirschbaum et al., 1993 ), this paradigm demonstrates the effectiveness of explicit social-evaluative components for successful stress induction. Other paradigms which include social evaluation are the Socially Evaluated Cold-Pressor Test (SECPT; Schwabe et al., 2008 ), the Simple Singing Stress Procedure (SSSP; Le et al., 2020 ), and the Maastricht Acute Stress Test (MAST; Smeets et al., 2012 ). Since these common stress induction paradigms depend on the social-evaluative element, it is difficult to use them during COVID-19 due to contact restrictions and stay-at-home practices ( Table 1 ).
Most commonly used stress induction paradigms in their feasibility under circumstances of the COVID-19 pandemic as assessed by the following three criteria: (1) Apart from any contact with the experimenter, do they include a social interaction for stress inductive purposes that requires a physical social encounter? (2) Are facial expressions of the source of the social-evaluative threat important for the paradigm’s stress inductive potential so that face masks may restrict their success? (3) Do they require the participant to come to the laboratory? In (4), we evaluate if the paradigms are feasible with unchanged stress inductive potential under conditions of the COVID-19 pandemic.
Abbreviations: TSST = Trier Social Stress Test; CPT = Cold-Pressor Test; SEPCT = Socially Evaluated Cold-Pressor Test; SSSP = Simple Singing Stress Procedure; PASAT-C = Paced Auditory Serial Addition Task – Computer Version; MMST = Mannheim Multicomponent Stress Test; MAST = Maastricht Acute Stress Test; MIST = Montreal Imaging Stress Test; SET = Social-Evaluative Threat Paradigm; MISTiC = Minnesota Imaging Stress Test in Children; IMPRESS = Imaging Paradigm for Evaluative Social Stress.
*Note that the TSST might still be conducted during the COVID-19 pandemic with adaptations made to the procedures. These may include that all people involved (experimenter, panel members, participants) wear face masks or keep an adequate distance (using shields, different rooms, windows and intercom systems). For such adaptations, however, future research needs to clarify in how far they alter the effectivity of the TSST.
**Note that even though facial expressions are not crucial for stress induction in the SSSP as the participant is not confronted with a panel and the experimenter is standing behind the participant during the singing procedure, face masks may severely impair participants while singing.
***Note that in the IMPRESS paradigm, participants are confronted with a panel that may convey social-evaluative threat via facial expressions but that the panel is pre-recorded. Therefore, under conditions of the COVID-19 pandemic, it may be possible to show a video that was recorded under pre-pandemic conditions in which panel members were not required to wear masks.
Of note, stress induction paradigms such as the TSST may still be applied in research laboratories during the pandemic, with adaptations made due to COVID-19 ( Table 1 ). For example, it seems feasible to conduct a TSST setting an adequate distance between participant and panel by using shields, different rooms, windows or intercom systems and by ensuring that all people involved wear face masks. To the best of our knowledge, several laboratories opted to adapt TSST-procedures in such a way implementing shields and face wearing for people involved including panel members (Heinrichs, 2021, pers communication; Pützer and Wolf, 2021, pers communication; Rohleder, 2021, pers communication). Even though such adaptations may probably not render the TSST ineffective, it is conceivable that they alter the paradigm’s impact. In particular, wearing of face masks might not be an ideal solution. For example, it has been shown that they impair social communication ( Calbi et al., 2021 ; for a review on masks affecting face reading, see Pavlova and Sokolov, 2021 ). Hence, it is reasonable to assume that masks covering facial expressions may also alter social-evaluative components in stress induction procedures.
As far as we can conclude from the literature, this hypothesis has yet not been experimentally tested. However, there is evidence in children supporting this assumption. For example, Guarnera et al. (2015) report that neutral expressions were better discriminated when the mouth region of a face was shown. In the TSST, the panel is instructed to maintain a neutral expression in order to not provide any feedback ( Kirschbaum et al., 1993 ). Therefore, the mouth region – that is covered by face masks – may be of great importance to effectively convey this attitude. On the contrary, one may argue that face masks may even help panel members to not show any feedback and to keep their neutral attitude. Along these lines, one may, however, still doubt whether face masks may be considered neutral themselves as for many people they may represent the epitome of the COVID-19 pandemic and hence be associated with negative emotions and unpleasant memories. Future research should empirically test in how far TSST-procedures adapted for mask wearing may alter the effectiveness of the paradigm.
Of course, there are stress induction paradigms without an explicit social-evaluative component such as the Paced Auditory Serial Addition Task - Computer Version (PASAT-C; Lejuez et al., 2003 ), the Mannheim Multicomponent Stress Test (MMST; Reinhardt et al., 2012 ) or the Cold-Pressor Test (CPT; first described by Hines and Brown (1932) ). In turn, these paradigms are also less powerful in activating HPA-related parameters of the stress response. However, these paradigms, among all others, also require a presence at the laboratory and are thus still difficult to apply at the time of a pandemic ( Table 1 ).
As a result, the COVID-19 pandemic has challenged stress researchers to re-evaluate their methodological repertoire regarding the need for presence at the laboratory ( Kirschbaum, 2021 ). As Table 1 illustrates, stress researchers can either choose between paradigms that feature a social-evaluative component in form of an in-person social encounter (TSST, SECPT, SSSP, MAST) or paradigms that lack social-evaluative elements (CPT, PASAT-C, MMST).
While the significance of psychosocial components for stress induction is undisputed, this does not necessarily imply that in-person encounters are needed to implement social-evaluative threat. Along these lines, alternative approaches for laboratory stress induction are needed during COVID-19. Once established, such approaches may also stimulate the field beyond the pandemic.
4. Perspectives for stress research during the current COVID-19 pandemic
As outlined above, it is their social-evaluative component that mainly impedes the use of established stress induction paradigms under conditions of the COVID-19 pandemic. Even though we mentioned experimental approaches that lack this component, it is generally regarded a key to valid and ecological stress induction. Therefore, it is worth asking whether stress researchers are forced to decide between (a) conducting (less efficient) stress induction without social-evaluative threat, (b) suspending their studies until the end of the pandemic, or (c) whether there are some promising alternatives to the induction of psychosocial stress.
Recent work indicates that it is possible to deviate from in-person social encounters of participants and panel in order to create social-evaluative threat. For example, Andrews et al. (2007) demonstrated that stress reactivity can be achieved by means of an invisible TSST panel. Düsing et al. (2016) , amongst others, triggered significant elevations in cortisol levels by replacing the panel by a video camera. Of note, Düsing et al. (2016) considered their application a milder version of the TSST and accordingly expected a less pronounced increase in cortisol levels. This is in line with Dickerson and Kemeny (2004) who provided meta-analytic evidence that stronger social evaluation produces larger effects sizes. This suggests that it is not sufficient to consider differences in mean cortisol release as these do not take into account the strength of cortisol reactivity. In sum, one may conclude that HPA axis reactivity may be a function of the intensity of the social-evaluative component which one can manipulate according to individual research proposes ( Andrews et al., 2007 ). For basic stress research, it may be desirable to implement significant social-evaluative threat (feasible under the given circumstances) in order to achieve substantial cortisol reactivity.
Labuschagne et al. (2019) concluded that due to the short methodological description of the TSST in the original publication, the paradigm likely varies with respect to its actual administration in different laboratories. Although this may account for differences observed in participants’ stress responses between different laboratories and different studies, the general effectiveness of the TSST seems highly conserved. Therefore, future research should clarify which procedural components are truly central to the validity of the paradigm and which ones are rather optional or may serve as target for further adaptations. Narvaez Linares et al. (2020) , for example, provided a recent systematic overview over methodological variations of TSST applications over different laboratories and reveal several factors (e.g., number of panel members, number from which serial subtraction is started) that may impede reliability and replicability for this paradigm.
4.1. Stress research in the MRI scanner
Importantly, research contexts in which in-person encounters of participants and panel are not feasible already exist. The MRI setting - which harbors great potential to advance our knowledge about the neural mechanisms of stress processing – renders it impossible to produce social-evaluative threat by means of direct social confrontation ( Noack et al., 2019 ). However, several different solutions for neuroimaging stress induction in the scanner have been suggested ( Berretz et al., 2021 ).
For example, in the Social-Evaluative Threat Paradigm (SET; e.g., Eisenbarth et al., 2016 ), participants are instructed to prepare for a speech that will be recorded and evaluated by others that are not actually present. In the Montreal Imaging Stress Task (MIST; Dedovic et al., 2005 ), participants are asked to solve challenging arithmetic tasks under extreme time pressure while feedback on their average performance relative to the performance of alleged other participants is given. Furthermore, in the ScanSTRESS paradigm ( Streit et al., 2014 ), a panel is physically present but seated in the control room of the scanner. The panel is shown on the participant’s screen via a live video feed in order to provide individual (disapproving) feedback on the participant’s performance in arithmetic tasks. For adolescents a similar approach is implemented in the Minnesota Imaging Stress Test in Children (MISTiC; Herzberg et al., 2020 ). Highly consistent with the original TSST, after a 5 min. preparation period, participants have to give a speech introducing themselves to a jury panel shown via live video feed while lying in the scanner. Afterwards, participants have to perform a multiple-choice arithmetic task. Whereas imaging is possible during the arithmetic task of this paradigm, the speech task cannot be accompanied by scanning due to interference of scanner noise with the task. Moreover, speaking-associated movements would negatively affect scanning quality due to motion artefacts. Similarly, the recently developed Imaging Paradigm for Evaluative Social Stress (IMPRESS; Fehlner et al., 2020 ) requires participants to prepare and give a speech in front of a pre-recorded panel.
These adaptations to contextual challenges clearly suggest options for how established stress induction paradigms can be adjusted to new settings. Since the MRI setting and the current pandemic situation have in common that in-person encounters inducing social-evaluative threat are not feasible, researchers currently conducting laboratory stress research may find inspiration in the progress made in MRI-based stress research.
Importantly, for the MRI setting itself, different neuroimaging stress induction paradigms lead to overlapping but also distinct activations ( Berretz et al., 2021 ). Therefore, further research in this field should define which paradigm is suited best for the individual study purposes. To this end, it is crucial to clarify whether there is a certain stereotypic neural stress response, and if so, how discrepancies produced by different stress induction paradigms can be explained ( Berretz et al., 2021 ). In general, one can conclude that approaches to social-evaluative stress induction in the MRI context are promising and may help to advance basic laboratory stress research when it comes to remote stress induction.
4.2. Stress induction with virtual reality and pre-recordings
Regarding the TSST and its application in traditional laboratory settings, several adaptations of the procedure that aimed to render it independent of a physical panel had been published well before the onset of the COVID-19 pandemic ( Helminen et al., 2019 ; Jönsson et al., 2010 ; Zimmer et al., 2019 ). These protocols can be classified into two broader categories: virtual reality and pre-recorded applications. In both cases, social-evaluative threat is realized without any in-person social encounter. In the virtual reality TSST (TSST-VR), participants are confronted with avatars in contrast to a real panel ( Fallon et al., 2016 ; Helminen et al., 2019 ; Standard et al., 2020 ). In the context of the COVID-19 pandemic, such procedures reduce the number of people that meet at the laboratory and thereby reduce the risk for infection. However, this is still not completely without risks. For example, experimenters need to clean and disinfect VR devices which may be contaminated. In pre-recorded applications of the TSST, participants are exposed to a real panel, but this is neither presented in person nor in real-time, but in pre-recorded videos ( DeJoseph et al., 2019 ; Hawn et al., 2015 ; Smith et al., 2020 ). More details on procedural aspects of TSST-VR and pre-recorded versions will be provided below, along with information on effect sizes.
Adapting both experimental paradigms and therapeutic interventions for virtual reality has been a key objective in a number of other research fields ( Emmelkamp, 2005 ; Lohse et al., 2014 ; Slater, 2009 ). Virtual reality relies on computer-generated sensory input that resembles physical experience sufficiently enough to create the illusion of operating within real world scenarios ( Fallon et al., 2016 ). On a neuronal level, such simulations suffice to activate a system that encodes imaginations of real-world scenarios in terms of schemas so that minimal computer-generated information is needed to trigger comprehensive concepts of social-evaluative threat ( Fallon et al., 2016 ) or fear ( Diemer et al., 2014 ; Parsons and Rizzo, 2008 ). Along these lines, even the confrontation with a virtual panel should evoke social conventions ( Zimmer et al., 2019 ).
A recent meta-analysis by Helminen et al. (2019) on 13 studies using different versions of the TSST-VR providing a total sample size of n = 266 participants concluded that this procedure generally serves to successfully induce stress as indicated by a significant cortisol response. With ESsg = 0.65 (interpreted similar to effect sizes of Cohen’s d according to Helminen et al. (2019) ), the average size of this effect was moderate, suggesting that, albeit significant, the cortisol response elicited by TSST-VR seems to be smaller. In comparison, cortisol responses in the standard TSST typically reach large effect sizes. For example, it has been approximated by d = 0.925 by Allen et al. (2014) or d = 0.93 by Goodman et al. (2017) . Zimmer et al. (2019) as well as Shiban et al. (2016) who directly compared applications of the TSST in virtual reality and in vivo corroborate this. However, as the general patterns of cortisol reactivity largely resemble each other for the virtual and original versions of the TSST, these authors concluded that the TSST-VR has a similar power to the original TSST.
However, success in triggering the desired stress response might depend on technology providing the resolution needed to ensure a realistic feeling of being present in that virtual environment ( Jönsson et al., 2010 ; Montero-López et al., 2016 ). Hence, one would assume that the more input is derived from the virtual reality (i.e., the more immersive the paradigm is), the stronger emerges the feeling of being involved and spatially present within this virtual world ( Slater, 2009 ). This was also shown by Helminen et al. (2019) as effect sizes of individual studies using the TSST-VR seemed to be affected (amongst other factors) by the immersivity of the virtual environment. Specifically, these authors compared two groups of studies relying on high and low immersive environments and found greater mean effect sizes for studies using more immersive virtual realities. As a consequence, it seems desirable to opt for more immersive Computer Automatic Virtual Environment (CAVE) systems or head-mounted displays ( Helminen et al., 2019 ).
Besides low immersivity, it is detrimental when participants take the position of an “observer” to the scene and cannot interact with the panel. Even though this aspect was not examined in its influence on effect sizes of individual studies in the meta-analysis by Helminen et al. (2019) , low interactivity may severely diminish the experience of social-evaluative threat ( Fallon et al., 2016 ; Jönsson et al., 2010 ). To prevent this in the TSST-VR, avatars representing the panel are programmed to show subtle gestures. These movements as well as other interactive features are typically activated by an experimenter who remains invisible for the participant. Doing so allows matching the behavior of the avatars with the performance of the participant in order to account for some sort of interaction ( Fallon et al., 2016 ; Fich et al., 2014 ; Jönsson et al., 2010 ; Liu and Zhang, 2020 ). Some protocols do not only feature this situational flexibility for the panel’s non-verbal appearance but make further use of pre-recorded audiotapes in order to give a voice to their panel ( Jönsson et al., 2010 ; Santl et al., 2019 ; Shiban et al., 2016 ).
In contrast, Standard et al. (2020) addressed the idea of minimal interaction in a non-peer reviewed conference contribution by introducing a TSST-VR that runs independently of any experimenter. However, to the best of our knowledge, this procedure was not yet empirically tested so that it remains unclear whether it may suffice to trigger a substantial stress response.
TSST variants using pre-recorded panels are an alternative (or even add-on) to VR-based approaches. In this context, it was acknowledged that a TSST-VR might benefit from a pre-recorded panel as it resembles a real panel more than computer-generated avatars ( Kelly et al., 2007 ). However, in-vivo application of the TSST still led to higher increases in cortisol levels (90 %) than the procedure introduced by Kelly et al. (2007) (30 %).
Other studies such as Hawn et al. (2015) or Smith et al. (2020) use pre-recorded panels in non-avatar 2D environments (not considered immersive by Helminen et al. (2019) ). In comparison to a neutral condition, Hawn et al. (2015) reported their procedure to produce significant cortisol reactivity with an effect size of η p 2 = 0.11 corresponding to a medium effect of d = 0.70. However, a traditional TSST procedure triggered greater cortisol levels compared to the pre-recorded version with η p 2 = 0.18 ( Hawn et al., 2015 ) corresponding to a large effect of d = 0.94. Smith et al. (2020) , adapting the procedure to a TSST-C ( Buske-Kirschbaum et al., 1997 ) for application amongst children, produced responder rates comparable to the original version of the TSST-C ( Smith et al., 2020 ). Indeed, for adolescent participants, such TSST-variants are not new in that Westenberg et al. (2009) , for example, used the so-called Leiden Social Stress Test requiring adolescent participants to deliver a speech in front of a pre-recorded classroom of peers just a decade earlier. For their study it may be worth mentioning that participants were informed about the nature of the task in advance in order to prepare their speech at home. The delivery itself, however, was still performed at the laboratory. Interestingly, DeJoseph et al. (2019) chose the same video-based approach but conducted their study comprehensively at the children’s homes, thereby introducing a home-based procedure of the TSST (TSST-H). While traveling is typically demanded from participants when they are invited to a laboratory, this study was quite unusual in that it sent researchers to their participants’ local facilities. However, under pandemic conditions, both types of travels should be avoided. In the following, we therefore review approaches that do not involve traveling for either party.
4.3. Stress induction with online applications
Recently, online applications have further advanced the TSST protocol ( Eagle et al., 2021 ; Gunnar et al., 2020 ; Harvie et al., 2021 ; Kirschbaum, 2021 ). As outlined in Section 4.2 , one critical aspect that may reduce the success of stress induction in virtual reality or video-based applications of the TSST lies in restricted opportunities to interact with avatars or a pre-recorded panel. This issue is resolved in a recently developed online version of the TSST (TSST-OL), which implements the original protocol in a video communication software ( Gunnar et al., 2020 ). In such online adaptations of the TSST, the panel still consists of real people who convene in real time.
In detail, in the TSST-OL, the investigator arranges an online meeting that participant as well as experimenter can participate in from home. Within this meeting, Gunnar et al. (2020) conserved the original administration of the TSST-C and demonstrated that their online version was able to elicit a significant cortisol response in adolescents. However, as yet, no study has directly compared the TSST-OL with the original in-person TSST administration. Therefore, it remains unknown whether an online application is similarly effective in triggering stress reactivity. Of note, Gunnar et al. (2020) provided an effect size of d z = 0.57 for the peak in cortisol release. This is comparable with the most recent meta-analysis on the TSST-C (in the traditional application), approaching the mean effect size for cortisol reactivity with d’ = 0.47. ( Seddon et al., 2020 ). As Gunnar et al. (2020) further provide a detailed study protocol, they enable other laboratories to perform a direct comparison between online and in-person administration of the TSST. Kirschbaum (2021) – the developer of the original protocol – stated that modifications of the TSST to online settings are needed under conditions of the current COVID-19 pandemic, thereby encouraging such approaches.
Other publications are echoing the trend for online adaptations of the TSST ( Eagle et al., 2021 ; Harvie et al., 2021 ). Both similarly applied the original TSST protocol in video conference software but referred to it as an internet-delivered TSST (iTSST) instead of the TSST-OL. While Gunnar et al. (2020) exclusively included adolescents in their study, Eagle et al. (2021) and Harvie et al. (2021) further established a highly similar procedure for adult participants. However, similar to the study by Gunnar et al. (2020) , Eagle et al. (2021) lack a control condition. In contrast, Harvie et al. (2021) chose to expose all their participants to a stress as well as a control condition which was represented by an online adaptation of the placebo TSST ( Het et al., 2009 ). Even though these three studies applied different methods of statistical hypotheses testing, they do come to the shared conclusion that online adaptations of the TSST serve well to trigger a stress response. This conclusion was based on self-reports of stress or stress questionnaires ( Eagle et al., 2021 ; Gunnar et al., 2020 ; Harvie et al., 2021 ), sympathetic ( Gunnar et al., 2020 ) and parasympathetic activation ( Eagle et al., 2021 ; Harvie et al., 2021 ) as well as cortisol release ( Gunnar et al., 2020 ).
The range of parameters used by different studies to validate the TSST-OL, however, is countered by the fact that individual studies often miss important dimensions of the stress response. For example, Harvie et al. (2021) as well as Eagle et al. (2021) did not measure HPA axis related variables. This may prevent a direct comparison to previous work with the standard TSST. To address this issue, we will discuss the comprehensive assessment of stress markers in Section 4.5 . In general, for true validation of the TSST-OL, studies should strive for a multilevel assessment of the stress response including measures of self-reported stress as well as biomarkers shedding light on the overall activation under stress ( Epel et al., 2018 ).
4.4. Stress induction with smartphones
In addition to online applications using computers, smartphones have a high potential to complete the methodological repertoire used in psychological (stress) research ( Miller, 2012 ). In this context, several applications have been designed for usage among patient groups. For example, Wisniewski et al. (2019) designed a platform including a whole battery of simplified neuropsychological tests that appear in form of “games”. More specifically, Lam et al. (2021) used smartphones to monitor cognitive functioning in multiple sclerosis (MS) patients by means of an app-based version of the Symbol Digit Modalities Test (SDMT; Smith, 1982 , 1968 ). Since in both studies, the apps were compatible with common smartphone software, it seems conceivable to also apply them in healthy samples in order to address non-clinical research questions. Broader application was already intended for the iDichotic app ( Bless et al., 2013 ), a smartphone-based implementation of the dichotic listening task. This app has been used successfully in a number of studies on language lateralization in participants from all over the world ( Beste et al., 2018 ; Ocklenburg et al., 2016 ; Schmitz et al., 2018 ).
Stress induction via smartphone seems feasible in principle, given that it should be reasonably easy to run a TSST-OL in video communication software on smartphones. Compared to online adaptations, added value may lie in the increased portability of smartphones relative to laptop computers. However, small screen size might be problematic. For instance, Gunnar et al. (2020) only included participants if their devices had at least a 13-inch screen.
Nevertheless, smartphone applications allow data collection in larger samples than online versions ( Bless et al., 2013 ). Moreover, smartphone-based research can be done without the supervision of any experimenter. This perspective was also emphasized by Torous et al. (2020) , with a focus on the COVID-19 pandemic. These authors state that the current crisis requires asynchronous telehealth provided by app-based tools. For researchers, such a development may be considered beneficial as it strongly increases accessibility and scalability of data ( Torous et al., 2020 ).
However, stress researchers might not benefit from unlimited scalability as studies typically depend on a more in-depth assessment of variables whose quality might suffer. In line with that, it is widely accepted that stress should not solely be measured by behavioral or self-reported parameters ( Dickerson and Kemeny, 2004 ; Epel et al., 2018 ). Therefore, in the next paragraph, we will review techniques to ensure reliable assessment of stress markers in advanced versions of established stress induction protocols.
4.5. Beyond stress induction: assessment of the stress response
A factor that should not be omitted when evaluating new approaches to stress induction is the reliable assessment of stress markers ( Harvie et al., 2021 ). The subjective psychological dimension of the stress response is typically assessed by means of questionnaires or other self-reports ( Epel et al., 2018 ) which can easily be adapted to procedures such as the TSST-OL. Online survey software enables collection of self-reported data by means of a shared link and can be used to obtain subjective measures of stress during a video call on any device with stable internet connection.
Portable devices like smartphones further harbor the potential for more advanced self-report techniques, like ecological momentary assessment (EMA). EMA refers to a whole battery of methods and can be classified as an approach to collect data in real-time in an individual’s ecological context ( Shiffman et al., 2008 ). With regard to stress research, EMA is capable of registering random fluctuations in individual stress levels at (random or fixed) time intervals ( Kudielka et al., 2012 ; Schlotz, 2011 ; Shiffman et al., 2008 ). Sicorello et al. (2020) , for example, asked their participants over several days to complete stress-related questionnaires on their smartphones. They further monitored circumstances associated with observed variations. Sicorello et al. (2020) were able to show that the participants’ susceptibility to external events was shaped by variation in the serotonin transporter gene. Vice versa, EMA allows stress researchers to systematically track fluctuations in individual stress levels along a specific stressful event (event-based monitoring) ( Shiffman et al., 2008 ). In the context of the COVID-19 pandemic, a smartphone-based longitudinal EMA approach was used by Huckins et al. (2020) in a student sample to reveal a COVID-19 related increase in symptoms of anxiety and depression.
The assessment of physiological stress markers might come along with such approaches. As EMA ranges from written self-reports over telephone interviews to physiological assessments by means of sensors ( Harari et al., 2016 ; Shiffman et al., 2008 ; Wang et al., 2014 ), other stress markers like heart rate or blood pressure can be recorded, too, possibly in parallel with saliva samples ( Anjum et al., 2011 ; Foody et al., 2014 ; Steptoe et al., 2007 ). Beyond EMA-related measurement, in order to assess sympathetic parameters, Eagle et al. (2021) opted to mail ambulatory cardiac hardware to their participants’ homes and have it sent back to them. However, since this procedure may not be feasible in larger samples, other researchers opted to use wrist-worn Polar watches (e.g., Jentsch and Wolf, 2020 ) or Apple watches (e.g., Hernando et al., 2018 ), sometimes in combination with heart rate chest straps which can track a number of activity parameters ( Wang et al., 2017 ).
Importantly, such approaches depend on additional sensors or even invasive devices ( Ciman and Wac, 2016 ). As these are typically portable or even wearable, they allow usage outside the laboratory, but must be purchased and transported, nevertheless. Therefore, to enable broad usage, devices need to function computer- or smartphone-based. This opportunity was already acknowledged in a TSST-OL variant by Harvie et al. (2021) who assessed heart rate variability on top of self-reported stress by means of suitable smartphone software. A growing body of literature is debating the use of smartphone's built-in software or sensors, given that most devices are already equipped with technology capable of collecting a wealth of information on people’s behavioral lifestyle patterns ( Can et al., 2019 ; Garcia-Ceja et al., 2016 ; Harari et al., 2016 ; Miller, 2012 ). Therefore, data collected by onboard smartphone sensors may provide a proxy for individual behavior, allowing for so-called digital phenotyping ( Wisniewski et al., 2019 ).
Measurement of HPA axis-related endocrine parameters seems practicable as well. Typically, stress researchers ask participants for saliva samples to extract cortisol. It appears to be a straight-forward approach to mail collection material to participants when using online stress paradigms. For example, Gunnar et al. (2020) opted to mail all material needed for saliva collection to their participants’ homes and let them return the material after study completion. And this was not a novel idea. Friedman et al. (1988) , for example, already used it decades ago in the so-called CARDIA (Coronary Artery Risk Development in Young Adults) study. It has been shown that cortisol is relatively stable in non-frozen mailed samples ( Clements and Richard, 1998 ) or can at least be assumed to survive a regular postal delivery taking a few days at room temperature ( Garde and Hansen, 2005 ). However, it does seem critical to instruct participants well on how to properly take saliva samples in order to ensure data quality ( Adam and Kumari, 2009 ; Lucas et al., 2019 ).
Saliva also allows for the extraction of alpha amylase which is considered an indirect marker for sympathetic activation ( Nater and Rohleder, 2009 ). Indeed, Gunnar et al. (2020) , assessed both alpha amylase and cortisol from samples taken at home and mailed back to the laboratory. It is important to note that studies systematically verifying stability in non-frozen samples or under thermal constraints for alpha amylase are as yet lacking. Still, there is initial evidence for salivary alpha amylase to remain stable when stored at room temperature for at least five consecutive days ( O’Donnell et al., 2009 ).
In conclusion, the collection of saliva samples for the assessment of stress hormones seems feasible in contexts outside the laboratory. Therefore, online or smartphone-based procedures for stress induction do not eliminate the possibility to assess all central stress markers in order to ascertain the success of a given stress exposure.
4.6. Beyond stress induction: assessment of stress affecting other cognitive processes
Having reviewed tools and techniques for stress induction and measurement of the stress response in times of the COVID-19 pandemic, it is crucial to mention that most studies in basic stress research focus on stress induction or the stress response in isolation. In contrast, some experiments explore how stress, or rather alterations in endocrine or other physiological parameters initiated in response to stress, affect cognitive functions ( Shields, 2020 ; Shields et al., 2016 ). Therefore, when developing new paradigms to experimentally induce stress, these must ideally allow to investigate other cognitive functions or behavioral patterns as well. Additional tasks that are occasionally conducted before or after stress exposure must ideally be similarly applicable in an online context. Creating new technologies to do so would not only enrichen stress research but psychological research as a whole ( Grootswagers, 2020 ) as the COVID-19 pandemic challenges the entire discipline with respect to conducting experiments outside of laboratory premises. Different platforms and software already allow online collection of behavioral data within psychological experiments. For a recent methodological comparison of current options in different internet browsers regarding their feasibility for specific task demands, see Bridges et al. (2020) and Anwyl-Irvine et al. (2020) .
For the matter at hand, it appears feasible to first stress participants in an online context and to subsequently ask them complete other (cognitive) tasks online in the aftermath of stress exposure.
5. Perspectives for stress research beyond the current COVID-19 pandemic
The technologies reviewed in Sections 4.2 – 4.4 will be crucial for stress researchers during the ongoing pandemic. However, they are also likely to stimulate the field far beyond COVID-19 and will set trends for future stress research ( Fig. 2 ).
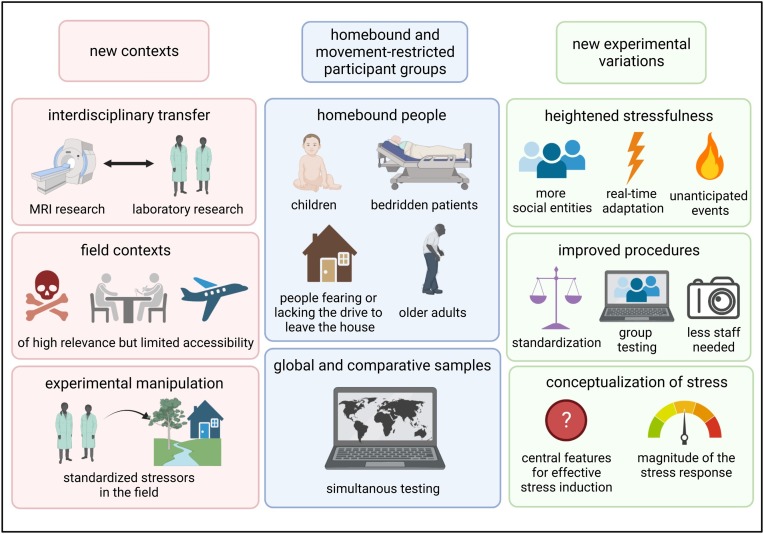
Schematic representation of opportunities of advanced stress induction procedures to advance the field beyond the COVID-19 pandemic. Adaptations of established paradigms may open (1) new contexts, (2) special samples, and (3) new experimental variations. (1) Interdisciplinary transfer may open up new avenues for psychological stress research. (2) Access to special samples such as homebound people, children, (neurological) patients, or people fearing or lacking the drive to leave their house as well as older people may be facilitated. Beyond that, global and comparative samples are accessible for simultaneous testing. (3) New experimental variations may allow manipulation of the stressfulness of established stress induction paradigms, e.g., by exposing participants to more social entities, unanticipated events, or procedures that are adjusted to individual responsiveness in real time. New technology can improve standardization and resource efficiency. The systematic manipulation of experimental variables may ultimately help advance the conceptualization of stress by identifying features that are central for stress induction and features that mediate the magnitude of the stress response. Created with BioRender.com.
5.1. New contexts
While settings such as the MRI can inspire basic stress research ( Lederbogen et al., 2011 ; Streit et al., 2014 ), this effect may not be unidirectional. New procedures to induce stress approaching the social-evaluative component without in-person contact may in return influence neuroimaging research. Exchange of new considerations may then lead to a theoretical consensus on key features of stress induction as well as their standardized implementation.
Moreover, novel approaches may allow the exploration of new contexts. The procedures reviewed in Sections 4.2 – 4.4 increase accessibility and may pave the way for research in field contexts. For example, it would be possible to investigate athletes participating in sports competitions. Such a sample was already tested by Rohleder et al. (2007) who studied professional ball room dancers. Exams are omnipresent stressors that are encountered in educational institutions around the globe. However, Merz et al. (2019) were the first ones to show that stress impairs memory retrieval in the aftermath of an oral presentation held in a naturalistic university context. Even though many people fear the confrontation with a prospective employer, stress effects in the context of job interviews or assessment centers have not been investigated in all aspects ( Feeney et al., 2015 ). Interestingly, Rockawin (2012) acknowledged that the modern technology can help applicants overcome anxiety in job interviews. In line with that, virtual reality is frequently used to simulate job interviews for candidates ( Kwon et al., 2013 ).
Previous research has been exploring other occupational applications for real world simulators and virtual reality features ( Kluge et al., 2014 ). For example, Kluge et al. (2019) found an adverse effect of social stress on monitoring processes in a simulated control task requiring multiple operating procedures. Such findings may be translated to field applications like cockpits in aviation ( Sieberichs and Kluge, 2018 ). Interestingly, mobile devices have already proven useful when it comes to guiding workers through multi-stage processes ( Kluge and Termer, 2017 ). Other workplaces setting people at risk for adverse effects on wellbeing are the health care sector ( Demerouti et al., 2000 ; Embriaco et al., 2007 ; McVicar, 2003 ; Wisetborisut et al., 2014 ) and the educational system ( Hall-Kenyon et al., 2014 ; Kelly and Berthelsen, 1995 ) as well as the stock market ( Blair‐Loy, 2009 ) or the seafaring industry ( Agterberg and Passchier, 1998 ; Iversen, 2012 ; Oldenburg et al., 2013 , 2009 ). Likewise, we see opportunities to use the TSST-OL to investigate individuals in war-affected or military regions in which the prevalence for mental disorders is higher than in other areas ( Husain et al., 2011 ; Njenga et al., 2006 ; Priebe et al., 2010 ; Summerfield, 2000 ) but which are rather inaccessible to researchers. Similarly, districts with high crime rates ( Dustmann and Fasani, 2016 ; Stafford et al., 2007 ) or poverty ( Belle, 1990 ; Das et al., 2007 ; Lund et al., 2011 ) are characterized by increased prevalence of mental health problems. Of note in this regard, the study by DeJoseph et al. (2019) that validated the TSST-H was integrated into the Family Life Project (FLP) initiated to approach families living in geographical regions of extreme poverty in the U.S.
With respect to clinical perspectives, participants could be tested in disorder-relevant, real-world fear-inducing settings such as airplanes or in elevated locations. Virtual reality or computer-based exposure therapy are already well-established ( Baños et al., 2002 ; Botella et al., 2004 ; Coelho et al., 2009 ; Krijn et al., 2004 ; Mühlberger et al., 2001 ; Tortella-Feliu et al., 2011 ). With novel approaches of stress assessment, one may query participants sitting in a doctor’s or dentist’s waiting room pre- and post-treatment to document stress and anxiety levels ( Appukuttan, 2016 ; Ramirez et al., 2017 ) or investigate techniques to reduce phobic anxiety ( Wang et al., 2017 ).
Of course, researchers have already found ways to investigate participants in special situations. However, when an experimental manipulation was desired, stress research in the field has as yet relied exclusively on real-life stressors ( Michaud et al., 2008 ). Importantly, this can impede robust conclusions since - as already discussed in Section 2.2 - naturalistic settings yield many confounding variables ( Epel et al., 2018 ). Therefore, experimental paradigms discussed in this review may be considered an opportunity to advance stress research in field contexts in two ways:
procedures evaluated in Sections 4.2 – 4.4 may encourage future studies to implement an experimental manipulation in terms of stress induction. (2) This kind of stress induction can be conducted in field contexts but still features all aspects of a standardized experimental manipulation ( Fallon et al., 2016 ; Gunnar et al., 2020 ). This might help identify features of field settings that are crucial to drive stress or anxiety ( Epel et al., 2018 ).
It is well-known that field stressors typically activate individuals more strongly than laboratory ones, possibly due to increased personal relevance and a higher intensity ( Dickerson et al., 2004 ; Dickerson and Kemeny, 2004 ; Epel et al., 2018 ; Michaud et al., 2008 ). Along these lines, the nature of the stressor rather than situational variables may be responsible for stronger stress responses in the field than the laboratory. Even though it might sound paradoxical, procedures that lack true personal relevance but are still feasible in field settings might shed light on such processes. By applying standardized stress induction paradigms such as the TSST-OL in naturalistic environments, researcher may be able to assess the weight that individual relevance gives to a stressor ( Dickerson et al., 2004 ; Dickerson and Kemeny, 2004 ).
Ultimately, such approaches may help us clarify why specific real-life stressors such as the COVID-19 pandemic affect individuals differently and may lead to mental health problems for some but not others ( Ehlert et al., 2001 ; Kudielka et al., 2009 ; Zänkert et al., 2019 ).
However, it is crucial to bear in mind that alike traditional stress induction, also online formats may cause burden and negative side effects for participants. Stress induction can lead to severe emotional responses and heightened arousal (e.g., Vors et al., 2018 ). Hence, ethical considerations should always be concerned in calculations on costs and benefits of new studies which might hold particularly true for above-mentioned examples of use in highly vulnerable individuals such as patient groups. Along these lines, we emphasize the importance for evaluation of online stress induction projects through local ethic committees as ethical votes are not lapsed due to increased practicability and applicability of online protocols. On top of that, comprehensive debriefing of participants in the aftermath of the procedure as well as opportunities for contact and communication beyond study completion are crucial to expand acceptable stress research to online settings ( Labuschagne et al., 2019 ).
5.2. Homebound and movement-restricted participant groups
Stress induction paradigms that are not bound to the laboratory setting have another advantage. They allow to access samples that cannot come to local research facilities or that are difficult to test with standard stress induction procedures. First and foremost, online applications would allow the assessment of older adults with limited movement space ( Jeon and Dunkle, 2009 ; Osmanovic-Thunström et al., 2015 ), bedridden, or homebound people ( Churproong et al., 2016 ; Ornstein et al., 2015 ; Qiu et al., 2010 ), provided they are easy to use and do not require extensive experience with digital technology. Moreover, home-based stress research also enables access to specific patient groups such as patients with neurodegenerative diseases likes MS for which a dysfunction of stress systems has been discussed as an important factor in pathogenesis as well as disease progression ( Gold et al., 2005 ). Interestingly, for such patients, previous research has established online tools or automatic internet-based programs for therapeutic purposes ( Fischer et al., 2015 ). The COVID-19 pandemic has further boosted online applications in this context. Moccia et al. (2020) , for example, reviewed different tools contributing to so-called tele-neurology which is focused on neurological examination of patients suffering from MS by means of video-based or digital technology in general.
Studies conducted at home might also be helpful when recruiting participants who fear or lack the drive to leave their homes due to severe forms of depression or anxiety disorders ( Cox et al., 2003 ; Hallam and Hafner, 1978 ; Kim et al., 2019 ; Loken et al., 2014 ; Ravesloot et al., 2016 ). Importantly, the latter aspect is especially relevant in the COVID-19 pandemic as stay-at-home practices seem to affect prevalence of anxiety and depression disorders ( Benke et al., 2020 ; Kumari and Mahla, 2021 ; Tang et al., 2020 ).
Stress research in children is particularly challenging as minors depend on parents or other family members to accompany them to the laboratory. As a result, study participation of children often fails due to logistic difficulties – a problem DeJoseph et al. (2019) combatted by visiting families at their homes. Tolep and Dougherty (2014) considered traveling to the laboratory itself a stressor, thereby questioning if testing in the laboratory actually accounts for a valid baseline of stress markers in general. The protocols reviewed in the Sections 4.2 – 4.4 may resolve such issues.
Likewise, online applications set new standards for stress research engaging in comparative cultural research because samples from all over the world are more accessible as long as they have access to a certain device with internet connection. To date, few studies have compared Scottish and Australian ( Pithers and Soden, 1998 ) or Turkish and Macedonian teachers ( Eres and Atanasoska, 2011 ) with respect to their stress levels and their coping abilities. Berry et al. (1987) reviewed publications on acculturative stress resulting from cultural changes confronting migrants, refugees, or ethnic minorities in Canada. However, these early studies solely assessed stress by means of questionnaire methods. In contrast, modern technology as well as experimental stress procedures reviewed in Section 4 allow for a more in-depth assessment. For example, different people from all over the world could meet in an online meeting and undergo a TSST-OL. Dufau et al. (2011) concluded that new technologies are capable of revolutionizing cognitive science in that suitable devices may help collect data from participants all over the world. Thereby, sampling biases originating from (1) the requirement to come to the designated research facilities, and (2) the natural homogeneity of study participants may be overcome.
Another opportunity may lie in new standards for specialized screening of desired samples. In general, many questions are as yet unanswered concerning individual differences in stress responsiveness. Online stress experiments would enable researchers to gather initial data from large samples first, and then to selectively invite only a subset of individuals to the laboratory for further testing who fulfill specific predefined criteria ( Parker et al., 2020 ). This may enable systematic and precise investigation of individuals showing a blunted or an elevated cortisol response to stress ( Kudielka et al., 2009 ; Kudielka and Wüst, 2010 ), for example.
5.3. New experimental variations
In addition to enabling stress research during the current COVID-19 pandemic, virtual reality and pre-recorded or online applications of stress induction paradigms also allow new experimental variations. From a methodological perspective, the TSST-VR or pre-recorded variations foster standardization and resource efficiency ( Fallon et al., 2016 ; Zimmer et al., 2019 ). From a conceptual point of view, TSST-VR and TSST-OL allow new directions in stress research in that key stress components can be implemented in ways that were not possible before. Such approaches may satisfy two objectives. On the one hand, the stressfulness of the actual stress inductive procedure could be modified. On the other hand, they would allow systematic manipulation in order to clarify which factors are central to stress induction and which variables determine the magnitude of the triggered stress response.
For example, in virtual reality it is not only possible to confront the participant with a panel consisting of two or three reviewers but with a whole audience ( Kothgassner et al., 2016 ; Owens and Beidel, 2015 ; Slater et al., 2006 ). This approach could determine if a participant’s stress experience might be increased with an increasing number of social entities ( Kothgassner et al., 2016 ; Montero-López et al., 2016 ). Such an approach is also possible for the TSST-OL in that a greater number of panel members might join the online meeting. Such an approach is also possible for the TSST-OL in that a greater number of panel members might join the online meeting. This would allow panel sizes of, e.g., 20 or more panel members, which would be unrealistic in the real-life TSST.
Moreover, it would be feasible to test more than one participant if they join the same online conference. This would resemble the group version of the TSST (TSST-G) which is established for laboratory stress research ( von Dawans et al., 2011 ).
It has been acknowledged that virtual reality systems may enable the assessment of physiological activation for a real-time adaptation of the virtual situation. Fallon et al. (2016) suggested adapting the intensity or the type of the social-evaluative threat in response to the autonomic activity of the participant (e.g., heart rate). The motivation would be to increase task difficulty or intensity of social-evaluative threat individually for each participant ( Fallon et al., 2016 ). However, one must bear in mind that such adjustments would also diminish the comparability and standardization of the procedure.
6. Conclusion
In conclusion, epidemiological, clinical, and basic stress research are needed to elucidate the effects of the current COVID-19 pandemic on wellbeing and the rising prevalence of mental disorders for a whole generation. With respect to experimental manipulations, the COVID-19 pandemic is a driving force to reconsider social-evaluative components and their implementation in established stress induction paradigms. We conclude that different adaptations can be made for experimental protocols such as the TSST, based on multiple available tools and technologies. Studies on stress will benefit from new approaches far beyond the COVID-19 pandemic. These will facilitate research in new contexts and with homebound and movement-restricted participant groups. Last but not least, they hold new perspectives for experimental variations in terms of flexibility to optimize protocols and experimental manipulations for individual research objectives.
KH was funded by the Deutsche Forschungsgemeinschaft (DFG, German Research Foundation) - project number GRK-2185/1 (DFG Research Training Group Situated Cognition) / Gefördert durch die Deutsche Forschungsgemeinschaft (DFG) - Projektnummer GRK-2185/1 (DFG-Graduiertenkolleg Situated Cognition).
The contribution of LSP and OTW was supported by the DFG within project B4 of the Collaborative Research Center (SFB) 874 “Integration and Representation of Sensory Processes” [project number 122679504] as well as by research unit FOR 2812 "Constructing Scenarios of the Past" [project number 419039274].
Declaration of Competing Interest
The authors report no declarations of interest.
Acknowledgements
The authors thank Jutta Peterburs for proofreading the manuscript.
- Achdut N., Refaeli T. Unemployment and psychological distress among young people during the COVID-19 pandemic: psychological resources and risk factors. Int. J. Environ. Res. Public Health. 2020;17 doi: 10.3390/ijerph17197163. [ DOI ] [ PMC free article ] [ PubMed ] [ Google Scholar ]
- Adam E.K., Kumari M. Assessing salivary cortisol in large-scale, epidemiological research. Psychoneuroendocrinology. 2009;34:1423–1436. doi: 10.1016/j.psyneuen.2009.06.011. [ DOI ] [ PubMed ] [ Google Scholar ]
- Agterberg G., Passchier J. Stress among seamen. Psychol. Rep. 1998;83:708–710. doi: 10.2466/pr0.1998.83.2.708. [ DOI ] [ PubMed ] [ Google Scholar ]
- Aguilera G. HPA axis responsiveness to stress: implications for healthy aging. Exp. Gerontol. 2011;46:90–95. doi: 10.1016/j.exger.2010.08.023. [ DOI ] [ PMC free article ] [ PubMed ] [ Google Scholar ]
- Allen A.P., Kennedy P.J., Cryan J.F., Dinan T.G., Clarke G. Biological and psychological markers of stress in humans: focus on the Trier Social Stress Test. Neurosci. Biobehav. Rev. 2014;38:94–124. doi: 10.1016/j.neubiorev.2013.11.005. [ DOI ] [ PubMed ] [ Google Scholar ]
- Allen A.P., Kennedy P.J., Dockray S., Cryan J.F., Dinan T.G., Clarke G. The trier social stress test: principles and practice. Neurobiol. Stress. 2017;6:113–126. doi: 10.1016/j.ynstr.2016.11.001. [ DOI ] [ PMC free article ] [ PubMed ] [ Google Scholar ]
- Amirkhan J.H. Stress overload in the spread of coronavirus. Anxiety Stress Coping. 2021;34:121–129. doi: 10.1080/10615806.2020.1824271. [ DOI ] [ PubMed ] [ Google Scholar ]
- Andrews J., Wadiwalla M., Juster R.P., Lord C., Lupien S.J., Pruessner J.C. Effects of manipulating the amount of social-evaluative threat on the cortisol stress response in young healthy men. Behav. Neurosci. 2007;121:871–876. doi: 10.1037/0735-7044.121.5.871. [ DOI ] [ PubMed ] [ Google Scholar ]
- Anjum B., Verma N.S., Tiwari S., Singh R., Mahdi A.A., Singh R.B., Singh R.K. Association of salivary cortisol with chronomics of 24 hours ambulatory blood pressure/heart rate among night shift workers. Biosci. Trends. 2011;5:182–188. doi: 10.5582/bst.2011.v5.4.182. [ DOI ] [ PubMed ] [ Google Scholar ]
- Ansell E.B., Rando K., Tuit K., Guarnaccia J., Sinha R. Cumulative adversity and smaller gray matter volume in medial prefrontal, anterior cingulate, and insula regions. Biol. Psychiatry. 2012;72:57–64. doi: 10.1016/j.biopsych.2011.11.022. [ DOI ] [ PMC free article ] [ PubMed ] [ Google Scholar ]
- Anwyl-Irvine A., Dalmaijer E.S., Hodges N., Evershed J.K. Realistic precision and accuracy of online experiment platforms, web browsers, and devices. Behav. Res. Methods. 2020:1–19. doi: 10.3758/s13428-020-01501-5. [ DOI ] [ PMC free article ] [ PubMed ] [ Google Scholar ]
- Appukuttan D.P. Strategies to manage patients with dental anxiety and dental phobia: literature review. Clin. Cosmet. Investig. Dent. 2016;8:35–50. doi: 10.2147/CCIDE.S63626. [ DOI ] [ PMC free article ] [ PubMed ] [ Google Scholar ]
- Baños R.M., Botella C., Perpiñá C., Alcañiz M., Lozano J.A., Osma J., Gallardo M. Virtual reality treatment of flying phobia. IEEE Trans. Inf. Technol. Biomed. 2002;6:206–212. doi: 10.1109/TITB.2002.802380. [ DOI ] [ PubMed ] [ Google Scholar ]
- Baxter A.J., Scott K.M., Ferrari A.J., Norman R.E., Vos T., Whiteford H.A. Challenging the myth of an “epidemic” of common mental disorders: trends in the global prevalence of anxiety and depression between 1990 and 2010. Depress. Anxiety. 2014;31:506–516. doi: 10.1002/da.22230. [ DOI ] [ PubMed ] [ Google Scholar ]
- Belle D. Poverty and women’s mental health. Am. Psychol. Nreia. 1990;45:385–389. doi: 10.1037//0003-066X.45.3.385. [ DOI ] [ Google Scholar ]
- Benke C., Autenrieth L.K., Asselmann E., Pané-Farré C.A. Stay-at-home orders due to the COVID-19 pandemic are associated with elevated depression and anxiety in younger, but not older adults: results from a nationwide community sample of adults from Germany. Psychol. Med. 2020:1–2. doi: 10.1017/S0033291720003438. [ DOI ] [ PMC free article ] [ PubMed ] [ Google Scholar ]
- Berretz G., Packheiser J., Kumsta R., Wolf O.T., Ocklenburg S. The brain under stress-A systematic review and activation likelihood estimation meta-analysis of changes in BOLD signal associated with acute stress exposure. Neurosci. Biobehav. Rev. 2021;124:89–99. doi: 10.1016/j.neubiorev.2021.01.001. [ DOI ] [ PubMed ] [ Google Scholar ]
- Berry J.W., Kim U., Minde T., Mok D. Comparative studies of acculturative stress. Int. Migr. Rev. 1987;21:491. doi: 10.2307/2546607. [ DOI ] [ Google Scholar ]
- Beste C., Arning L., Gerding W.M., Epplen J.T., Mertins A., Röder M.C., Bless J.J., Hugdahl K., Westerhausen R., Güntürkün O., Ocklenburg S. Cognitive control processes and functional cerebral asymmetries: association with variation in the handedness-associated gene LRRTM1. Mol. Neurobiol. 2018;55:2268–2274. doi: 10.1007/s12035-017-0485-7. [ DOI ] [ PubMed ] [ Google Scholar ]
- Blair‐Loy M. Work without end? Work Occup. 2009;36:279–317. doi: 10.1177/0730888409343912. [ DOI ] [ Google Scholar ]
- Bless J.J., Westerhausen R., Arciuli J., Kompus K., Gudmundsen M., Hugdahl K. “Right on all occasions?” - on the feasibility of laterality research using a smartphone dichotic listening application. Front. Psychol. 2013;4:42. doi: 10.3389/fpsyg.2013.00042. [ DOI ] [ PMC free article ] [ PubMed ] [ Google Scholar ]
- Blustein D.L., Duffy R., Ferreira J.A., Cohen-Scali V., Cinamon R.G., Allan B.A. Unemployment in the time of COVID-19: a research agenda. J. Vocat. Behav. 2020;119 doi: 10.1016/j.jvb.2020.103436. [ DOI ] [ PMC free article ] [ PubMed ] [ Google Scholar ]
- Bohlken J., Schömig F., Lemke M.R., Pumberger M., Riedel-Heller S.G. COVID-19-Pandemie: belastungen des medizinischen personals [COVID-19 pandemic: stress experience of healthcare workers - a short current review] Psychiatr. Prax. 2020;47:190–197. doi: 10.1055/a-1159-5551. [ DOI ] [ PMC free article ] [ PubMed ] [ Google Scholar ]
- Botella C., Osma J., Garcia-Palacios A., Quero S., Baños R.M. Treatment of flying phobia using virtual reality: data from a 1-year follow-up using a multiple baseline design. Clin. Psychol. Psychother. 2004;11:311–323. doi: 10.1002/cpp.404. [ DOI ] [ Google Scholar ]
- Boyraz G., Legros D.N. Coronavirus disease (COVID-19) and traumatic stress: probable risk factors and correlates of posttraumatic stress disorder. J. Loss Trauma. 2020;25:503–522. doi: 10.1080/15325024.2020.1763556. [ DOI ] [ Google Scholar ]
- Bridges D., Pitiot A., MacAskill M.R., Peirce J.W. The timing mega-study: comparing a range of experiment generators, both lab-based and online. PeerJ. 2020;8:e9414. doi: 10.7717/peerj.9414. [ DOI ] [ PMC free article ] [ PubMed ] [ Google Scholar ]
- Bridgland V.M.E., Moeck E.K., Green D.M., Swain T.L., Nayda D.M., Matson L.A., Hutchison N.P., Takarangi M.K.T. Why the COVID-19 pandemic is a traumatic stressor. PLoS One. 2021;16 doi: 10.1371/journal.pone.0240146. [ DOI ] [ PMC free article ] [ PubMed ] [ Google Scholar ]
- Brooks S.K., Webster R.K., Smith L.E., Woodland L., Wessely S., Greenberg N., Rubin G.J. The psychological impact of quarantine and how to reduce it: rapid review of the evidence. Lancet. 2020;395:912–920. doi: 10.1016/S0140-6736(20)30460-8. [ DOI ] [ PMC free article ] [ PubMed ] [ Google Scholar ]
- Brown S.M., Doom J.R., Lechuga-Peña S., Watamura S.E., Koppels T. Stress and parenting during the global COVID-19 pandemic. Child Abuse Negl. 2020;110 doi: 10.1016/j.chiabu.2020.104699. [ DOI ] [ PMC free article ] [ PubMed ] [ Google Scholar ]
- Bueno-Notivol J., Gracia-García P., Olaya B., Lasheras I., López-Antón R., Santabárbara J. Prevalence of depression during the COVID-19 outbreak: a meta-analysis of community-based studies. Int. J. Clin. Health Psychol. 2021;21 doi: 10.1016/j.ijchp.2020.07.007. [ DOI ] [ PMC free article ] [ PubMed ] [ Google Scholar ]
- Buske-Kirschbaum A., Jobst S., Wustmans A., Kirschbaum C., Rauh W., Hellhammer D.H. Attenuated free cortisol response to psychosocial stress in children with atopic dermatitis. Psychosom. Med. 1997:419–426. doi: 10.1097/00006842-199707000-00012. [ DOI ] [ PubMed ] [ Google Scholar ]
- Buske-Kirschbaum A., von Auer K., Krieger S., Weis S., Rauh W., Hellhammer D.H. Blunted cortisol responses to psychosocial stress in asthmatic children: a general feature of atopic disease? Psychosom. Med. 2003;65:806–810. doi: 10.1097/01.psy.0000095916.25975.4f. [ DOI ] [ PubMed ] [ Google Scholar ]
- Buske-Kirschbaum A., Ebrecht M., Hellhammer D.H. Blunted HPA axis responsiveness to stress in atopic patients is associated with the acuity and severeness of allergic inflammation. Brain Behav. Immun. 2010;24:1347–1353. doi: 10.1016/j.bbi.2010.06.013. [ DOI ] [ PubMed ] [ Google Scholar ]
- Calbi M., Langiulli N., Ferroni F., Montalti M., Kolesnikov A., Gallese V., Umiltà M.A. The consequences of COVID-19 on social interactions: an online study on face covering. Sci. Rep. 2021;11:2601. doi: 10.1038/s41598-021-81780-w. [ DOI ] [ PMC free article ] [ PubMed ] [ Google Scholar ]
- Calina D., Hartung T., Mardare I., Mitroi M., Poulas K., Tsatsakis A., Rogoveanu I., Docea A.O. COVID-19 pandemic and alcohol consumption: impacts and interconnections. Toxicol. Rep. 2021;8:529–535. doi: 10.1016/j.toxrep.2021.03.005. [ DOI ] [ PMC free article ] [ PubMed ] [ Google Scholar ]
- Can Y.S., Arnrich B., Ersoy C. Stress detection in daily life scenarios using smart phones and wearable sensors: a survey. J. Biomed. Inform. 2019;92 doi: 10.1016/j.jbi.2019.103139. [ DOI ] [ PubMed ] [ Google Scholar ]
- Chan S.M.S., Chiu F.K.H., Lam C.W.L., Leung P.Y.V., Conwell Y. Elderly suicide and the 2003 SARS epidemic in Hong Kong. Int. J. Geriatr. Psychiatry. 2006;21:113–118. doi: 10.1002/gps.1432. [ DOI ] [ PubMed ] [ Google Scholar ]
- Chew N.W.S., Lee G.K.H., Tan B.Y.Q., Jing M., Goh Y., Ngiam N.J.H., Yeo L.L.L., Ahmad A., Ahmed Khan F., Napolean Shanmugam G., Sharma A.K., Komalkumar R.N., Meenakshi P.V., Shah K., Patel B., Chan B.P.L., Sunny S., Chandra B., Ong J.J.Y., Paliwal P.R., Wong L.Y.H., Sagayanathan R., Chen J.T., Ying Ng A.Y., Teoh H.L., Tsivgoulis G., Ho C.S., Ho R.C., Sharma V.K. A multinational, multicentre study on the psychological outcomes and associated physical symptoms amongst healthcare workers during COVID-19 outbreak. Brain Behav. Immun. 2020;88:559–565. doi: 10.1016/j.bbi.2020.04.049. [ DOI ] [ PMC free article ] [ PubMed ] [ Google Scholar ]
- Churproong S., Jongkol P., Pasayadej K., Chadsarin S., Wattanapan P., Watakit P., Kittiwattanasak A., Sinjariyanon W. The strategy to encourage the bed ridden paraplegic patient to keep fit at home with the development of exercise machine. JSS. 2016;4 doi: 10.17265/2332-7839/2016.06.005. [ DOI ] [ Google Scholar ]
- Ciman M., Wac K. Individuals’ stress assessment using human-smartphone interaction analysis. IEEE Trans. Affect. Comput. 2016;9:51–65. doi: 10.1109/TAFFC.2016.2592504. [ DOI ] [ Google Scholar ]
- Clements A.D., Richard C.R. The relationship between salivary cortisol concentrations in frozen versus mailed samples. Psychoneuroendocrinology. 1998;23:613–616. doi: 10.1016/S0306-4530(98)00031-6. [ DOI ] [ PubMed ] [ Google Scholar ]
- Coelho C.M., Waters A.M., Hine T.J., Wallis G. The use of virtual reality in acrophobia research and treatment. J. Anxiety Disord. 2009;23:563–574. doi: 10.1016/j.janxdis.2009.01.014. [ DOI ] [ PubMed ] [ Google Scholar ]
- Cohen S., Janicki-Deverts D. Who’s stressed? Distributions of psychological stress in the United States in probability samples from 1983, 2006, and 2009. J. Appl. Soc. Psychol. 2012;42:1320–1334. doi: 10.1111/j.1559-1816.2012.00900.x. [ DOI ] [ Google Scholar ]
- Cohen S., Janicki-Deverts D., Miller G.E. Psychological stress and disease. J. Am. Med. Assoc. 2007;298:1685–1687. doi: 10.1001/jama.298.14.1685. [ DOI ] [ PubMed ] [ Google Scholar ]
- Cohen S., Gianaros P.J., Manuck S.B. A stage model of stress and disease. Perspect. Psychol. Sci. 2016;11:456–463. doi: 10.1177/1745691616646305. [ DOI ] [ PMC free article ] [ PubMed ] [ Google Scholar ]
- Colodro-Conde L., Couvy-Duchesne B., Zhu G., Coventry W.L., Byrne E.M., Gordon S., Wright M.J., Montgomery G.W., Madden P.A.F., Ripke S., Eaves L.J., Heath A.C., Wray N.R., Medland S.E., Martin N.G. A direct test of the diathesis-stress model for depression. Mol. Psychiatry. 2018;23:1590–1596. doi: 10.1038/mp.2017.130. [ DOI ] [ PMC free article ] [ PubMed ] [ Google Scholar ]
- Cox B.J., McWilliams L.A., Clara I.P., Stein M.B. The structure of feared situations in a nationally representative sample. J. Anxiety Disord. 2003;17:89–101. doi: 10.1016/S0887-6185(02)00179-2. [ DOI ] [ PubMed ] [ Google Scholar ]
- Crunfli F., Carregari V.C., Veras F.P., Vendramini P.H., Valença A.G.F., Marcelo Antunes A.S.L., Brandão-Teles C., Da Zuccoli G.S., Reis-de-Oliveira G., Silva-Costa L.C., Saia-Cereda V.M., Codo A.C., Parise P.L., Toledo-Teixeira D.A., de Souza G.F., Muraro S.P., Santos de Castro Í.M., Melo B.M.S., Almeida G.M., Firmino E.M.S., Paiva I.M., Silva B.M.S., Guimarães R.M., Ludwig R.G., Ruiz G.P., Knittel T.L., Gastão Davanzo G., Gerhardt J.A., Rodrigues P.B., Forato J., Amorim M.R., Silva N.B., Martini M.C., Benatti M.N., Batah S., Siyuan L., João R.B., Silva L.S., Nogueira M.H., Aventurato Í.K., de Brito M.R., Alvim M.K.M., Da Silva Júnior J.R., Damião L.L., de Paula Castilho Stefano M.E., Pereira de Sousa I.M., Da Rocha E.D., Gonçalves S.M., Da Silva L.H.L., Bettini V., de Campos B.M., Ludwig G., Mendes Viana R.M., Martins R., Vieira A.S., Alves-Filho J.C., Arruda E., Sebollela A.S., Cendes F., Louzada-Junior P., Donizeti Oliveira R., Cunha F.Q., Damásio A., Aurélio Ramirez Vinolo M., Munhoz C.D., Rehen S.K., Nakaya H.I., Mauad T., Duarte-Neto A.N., Da Silva L.F.F., Dolhnikoff M., Saldiva P., Fabro A.T., Farias A.S., Moraes-Vieira P.M.M., Módena J.L.P., Yasuda C.L., Mori M.A., Cunha T.M., Martins-de-Souza D. medRxiv; 2020. SARS-CoV-2 Infects Brain Astrocytes of COVID-19 Patients and Impairs Neuronal Viability. [ DOI ] [ Google Scholar ]
- Daly M., Robinson E. Psychological distress and adaptation to the COVID-19 crisis in the United States. J. Psychiatr. Res. 2021;136:603–609. doi: 10.1016/j.jpsychires.2020.10.035. [ DOI ] [ PMC free article ] [ PubMed ] [ Google Scholar ]
- Das J., Do Q.-T., Friedman J., McKenzie D., Scott K. Mental health and poverty in developing countries: revisiting the relationship. Soc. Sci. Med. 2007;65:467–480. doi: 10.1016/j.socscimed.2007.02.037. [ DOI ] [ PubMed ] [ Google Scholar ]
- de Kloet E.R., Joëls M., Holsboer F. Stress and the brain: from adaptation to disease. Nat. Rev. Neurosci. 2005;6:463–475. doi: 10.1038/nrn1683. [ DOI ] [ PubMed ] [ Google Scholar ]
- Dedovic K., Renwick R., Mahani N.K., Engert V., Lupien S.J., Pruessner J.C. The montreal imaging stress task: using functional imaging to investigate the effects of perceiving and processing psychosocial stress in the human brain. J. Psychiatry Neurosci. 2005;30:319–325. [ PMC free article ] [ PubMed ] [ Google Scholar ]
- DeJoseph M., Finegood E., Raver C.C., Blair C.B. PsyArXiv; 2019. Measuring Stress Reactivity in the Home: Preliminary Findings From a Version of the Trier Social Stress Test (TSST-H) Appropriate for Field-based Research [Preprint] [ DOI ] [ Google Scholar ]
- Demerouti E., Bakker A.B., Nachreiner F., Schaufeli W.B. A model of burnout and life satisfaction among nurses. J. Adv. Nurs. 2000;32:454–464. doi: 10.1046/j.1365-2648.2000.01496.x. [ DOI ] [ PubMed ] [ Google Scholar ]
- DeVries M.W., Wilkerson B. Stress, work and mental health: a global perspective. Acta Neuropsychiatr. 2003;15:44–53. doi: 10.1046/j.0013-0427.2003.00027.x. [ DOI ] [ PubMed ] [ Google Scholar ]
- Dickerson S.S., Kemeny M.E. Acute stressors and cortisol responses: a theoretical integration and synthesis of laboratory research. Psychol. Bull. 2004;130:355–391. doi: 10.1037/0033-2909.130.3.355. [ DOI ] [ PubMed ] [ Google Scholar ]
- Dickerson S.S., Gruenewald T.L., Kemeny M.E. When the social self is threatened: shame, physiology, and health. J. Pers. 2004;72:1191–1216. doi: 10.1111/j.1467-6494.2004.00295.x. [ DOI ] [ PubMed ] [ Google Scholar ]
- Diemer J., Mühlberger A., Pauli P., Zwanzger P. Virtual reality exposure in anxiety disorders: impact on psychophysiological reactivity. World J. Biol. Psychiatry. 2014;15:427–442. doi: 10.3109/15622975.2014.892632. [ DOI ] [ PubMed ] [ Google Scholar ]
- Dufau S., Duñabeitia J.A., Moret-Tatay C., McGonigal A., Peeters D., Alario F.-X., Balota D.A., Brysbaert M., Carreiras M., Ferrand L., Ktori M., Perea M., Rastle K., Sasburg O., Yap M.J., Ziegler J.C., Grainger J. Smart phone, smart science: how the use of smartphones can revolutionize research in cognitive science. PLoS One. 2011;6 doi: 10.1371/journal.pone.0024974. [ DOI ] [ PMC free article ] [ PubMed ] [ Google Scholar ]
- Düsing R., Tops M., Radtke E.L., Kuhl J., Quirin M. Relative frontal brain asymmetry and cortisol release after social stress: the role of action orientation. Biol. Psychol. 2016;115:86–93. doi: 10.1016/j.biopsycho.2016.01.012. [ DOI ] [ PubMed ] [ Google Scholar ]
- Dustmann C., Fasani F. The effect of local area crime on mental health. Econ. J. 2016;126:978–1017. doi: 10.1111/ecoj.12205. [ DOI ] [ Google Scholar ]
- Eagle D.E., Rash J.A., Tice L., Proeschold-Bell R.J. Evaluation of a remote, internet-delivered version of the Trier Social Stress Test. Int. J. Psychophysiol. 2021 doi: 10.1016/j.ijpsycho.2021.03.009. [ DOI ] [ PubMed ] [ Google Scholar ]
- Ehlert U., Gaab J., Heinrichs M. Psychoneuroendocrinological contributions to the etiology of depression, posttraumatic stress disorder, and stress-related bodily disorders: the role of the hypothalamus–pituitary–adrenal axis. Biol. Psychol. 2001;57:141–152. doi: 10.1016/S0301-0511(01)00092-8. [ DOI ] [ PubMed ] [ Google Scholar ]
- Eisenbarth H., Chang L.J., Wager T.D. Multivariate brain prediction of heart rate and skin conductance responses to social threat. J. Neurosci. 2016;36:11987–11998. doi: 10.1523/JNEUROSCI.3672-15.2016. [ DOI ] [ PMC free article ] [ PubMed ] [ Google Scholar ]
- Embriaco N., Papazian L., Kentish-Barnes N., Pochard F., Azoulay E. Burnout syndrome among critical care healthcare workers. Curr. Opin. Crit. Care. 2007;13:482–488. doi: 10.1097/MCC.0b013e3282efd28a. [ DOI ] [ PubMed ] [ Google Scholar ]
- Emmelkamp P.M.G. Technological innovations in clinical assessment and psychotherapy. Psychother. Psychosom. 2005;74:336–343. doi: 10.1159/000087780. [ DOI ] [ PubMed ] [ Google Scholar ]
- Epel E.S., Crosswell A.D., Mayer S.E., Prather A.A., Slavich G.M., Puterman E., Mendes W.B. More than a feeling: a unified view of stress measurement for population science. Front. Neuroendocrinol. 2018;49:146–169. doi: 10.1016/j.yfrne.2018.03.001. [ DOI ] [ PMC free article ] [ PubMed ] [ Google Scholar ]
- Eres F., Atanasoska T. Occupational stress of teachers: a comparative study between Turkey and Macedonia. Int. J. Humanit. Soc. Sci. 2011;17:59–65. [ Google Scholar ]
- Ettman C.K., Abdalla S.M., Cohen G.H., Sampson L., Vivier P.M., Galea S. Prevalence of depression symptoms in US adults before and during the COVID-19 pandemic. JAMA Netw. Open. 2020;3 doi: 10.1001/jamanetworkopen.2020.19686. [ DOI ] [ PMC free article ] [ PubMed ] [ Google Scholar ]
- Fallon M.A., Careaga J.S., Sbarra D.A., OʼConnor M.-F. Utility of a virtual trier social stress test: initial findings and benchmarking comparisons. Psychosom. Med. 2016;78:835–840. doi: 10.1097/PSY.0000000000000338. [ DOI ] [ PubMed ] [ Google Scholar ]
- Feeney J.R., McCarthy J.M., Goffin R. Applicant anxiety: examining the sex-linked anxiety coping theory in job interview contexts. Int. J. Sel. Assess. 2015;23:295–305. doi: 10.1111/ijsa.12115. [ DOI ] [ Google Scholar ]
- Fehlner P., Bilek E., Harneit A., Böhringer A., Moessnang C., Meyer-Lindenberg A., Tost H. Neural responses to social evaluative threat in the absence of negative investigator feedback and provoked performance failures. Hum. Brain Mapp. 2020;41:2092–2103. doi: 10.1002/hbm.24932. [ DOI ] [ PMC free article ] [ PubMed ] [ Google Scholar ]
- Fich L.B., Jönsson P., Kirkegaard P.H., Wallergård M., Garde A.H., Hansen Å. Can architectural design alter the physiological reaction to psychosocial stress? A virtual TSST experiment. Physiol. Behav. 2014;135:91–97. doi: 10.1016/j.physbeh.2014.05.034. [ DOI ] [ PubMed ] [ Google Scholar ]
- Fischer A., Schröder J., Vettorazzi E., Wolf O.T., Pöttgen J., Lau S., Heesen C., Moritz S., Gold S.M. An online programme to reduce depression in patients with multiple sclerosis: a randomised controlled trial. Lancet Psychiatry. 2015;2:217–223. doi: 10.1016/S2215-0366(14)00049-2. [ DOI ] [ PubMed ] [ Google Scholar ]
- Foody C., James J.E., Leader G. Parenting stress, salivary biomarkers, and ambulatory blood pressure in mothers of children with Autism Spectrum disorders. Res. Autism Spectr. Disord. 2014;8:99–110. doi: 10.1016/j.rasd.2013.10.015. [ DOI ] [ PubMed ] [ Google Scholar ]
- Friedman G.D., Cutter G.R., Donahue R.P., Hughes G.H., Hulley S.B., Jacobs D.R., Liu K., Savage P.J. Cardia: study design, recruitment, and some characteristics of the examined subjects. J. Clin. Epidemiol. 1988;41:1105–1116. doi: 10.1016/0895-4356(88)90080-7. [ DOI ] [ PubMed ] [ Google Scholar ]
- Fries E., Hesse J., Hellhammer J., Hellhammer D.H. A new view on hypocortisolism. Psychoneuroendocrinology. 2005;30:1010–1016. doi: 10.1016/j.psyneuen.2005.04.006. [ DOI ] [ PubMed ] [ Google Scholar ]
- Garcia-Ceja E., Osmani V., Mayora O. Automatic stress detection in working environments from smartphones’ accelerometer data: a first step. IEEE J. Biomed. Health Inform. 2016;20:1053–1060. doi: 10.1109/jbhi.2015.2446195. [ DOI ] [ PubMed ] [ Google Scholar ]
- Garde A.H., Hansen A.M. Long-term stability of salivary cortisol. Scand. J. Clin. Lab. Invest. 2005;65:433–436. doi: 10.1080/00365510510025773. [ DOI ] [ PubMed ] [ Google Scholar ]
- Garfin D.R., Silver R.C., Ugalde F.J., Linn H., Inostroza M. Exposure to rapid succession disasters: a study of residents at the epicenter of the Chilean Bío Bío earthquake. J. Abnorm. Psychol. 2014;123:545–556. doi: 10.1037/a0037374. [ DOI ] [ PubMed ] [ Google Scholar ]
- Glaser R., Kiecolt-Glaser J.K. Stress-induced immune dysfunction: implications for health. Nat. Rev. Immunol. 2005;5:243–251. doi: 10.1038/nri1571. [ DOI ] [ PubMed ] [ Google Scholar ]
- Gold S.M., Mohr D.C., Huitinga I., Flachenecker P., Sternberg E.M., Heesen C. The role of stress-response systems for the pathogenesis and progression of MS. Trends Immunol. 2005;26:644–652. doi: 10.1016/j.it.2005.09.010. [ DOI ] [ PubMed ] [ Google Scholar ]
- Goldstein D.S. Stress-induced activation of the sympathetic nervous system. Baillière’s Clin. Endocrinol. Metab. 1987;1:253–278. doi: 10.1016/S0950-351X(87)80063-0. [ DOI ] [ PubMed ] [ Google Scholar ]
- Goodman W.K., Janson J., Wolf J.M. Meta-analytical assessment of the effects of protocol variations on cortisol responses to the Trier Social Stress Test. Psychoneuroendocrinology. 2017;80:26–35. doi: 10.1016/j.psyneuen.2017.02.030. [ DOI ] [ PubMed ] [ Google Scholar ]
- Gouin J.-P. Chronic stress, immune dysregulation, and health. Am. J. Lifestyle Med. 2011;5:476–485. doi: 10.1177/1559827610395467. [ DOI ] [ Google Scholar ]
- Groarke J.M., Berry E., Graham-Wisener L., McKenna-Plumley P.E., McGlinchey E., Armour C. Loneliness in the UK during the COVID-19 pandemic: cross-sectional results from the COVID-19 psychological wellbeing study. PLoS One. 2020;15 doi: 10.1371/journal.pone.0239698. [ DOI ] [ PMC free article ] [ PubMed ] [ Google Scholar ]
- Grootswagers T. A primer on running human behavioural experiments online. Behav. Res. Methods. 2020 doi: 10.3758/s13428-020-01395-3. [ DOI ] [ PubMed ] [ Google Scholar ]
- Guarnera M., Hichy Z., Cascio M.I., Carrubba S. Facial expressions and ability to recognize emotions from eyes or mouth in children. Eur. J. Psychol. 2015;11:183–196. doi: 10.5964/ejop.v11i2.890. [ DOI ] [ PMC free article ] [ PubMed ] [ Google Scholar ]
- Gunnar M.R., Quevedo K. The neurobiology of stress and development. Annu. Rev. Psychol. 2007;58:145–173. doi: 10.1146/annurev.psych.58.110405.085605. [ DOI ] [ PubMed ] [ Google Scholar ]
- Gunnar M.R., Reid B.M., Donzella B., Miller Z.R., Gardow S., Tsakonas N.C., Thomas K.M., DeJoseph M., Bendezú J.J. Validation of an online version of the Trier Social Stress Test in a study of adolescents. Psychoneuroendocrinology. 2020;125 doi: 10.1016/j.psyneuen.2020.105111. [ DOI ] [ PMC free article ] [ PubMed ] [ Google Scholar ]
- Hagger M.S., Keech J.J., Hamilton K. Managing stress during the coronavirus disease 2019 pandemic and beyond: reappraisal and mindset approaches. Stress Health. 2020;36:396–401. doi: 10.1002/smi.2969. [ DOI ] [ PMC free article ] [ PubMed ] [ Google Scholar ]
- Hallam R.S., Hafner R.J. Fears of phobic patients: factor analyses of self-report data. Behav. Res. Ther. 1978;16:1–6. doi: 10.1016/0005-7967(78)90084-0. [ DOI ] [ PubMed ] [ Google Scholar ]
- Hall-Kenyon K.M., Bullough R.V., MacKay K.L., Marshall E.E. Preschool teacher well-being: a review of the literature. Early Childhood Edu. J. 2014;42:153–162. doi: 10.1007/s10643-013-0595-4. [ DOI ] [ Google Scholar ]
- Harari G., Lane N.D., Wang R., Crosier B.S., Campbell A.T., Gosling S.D. Using smartphones to collect behavioral data in psychological science: opportunities, practical considerations, and challenges. Perspect. Psychol. Sci. 2016;11:838–854. doi: 10.1177/1745691616650285. [ DOI ] [ PMC free article ] [ PubMed ] [ Google Scholar ]
- Harvie H., Jain B., Nelson B.W., Knight E.L., Roos L.E., Giuliano R.J. PsyArXiv; 2021. Induction of Acute Stress Through an Internet-delivered Trier Social Stress Test as Assessed by Photoplethysmography on a Smartphone. [ DOI ] [ PubMed ] [ Google Scholar ]
- Hassard J., Teoh K.R.H., Visockaite G., Dewe P., Cox T. The cost of work-related stress to society: a systematic review. J. Occup. Health Psychol. 2018;23:1–17. doi: 10.1037/ocp0000069. [ DOI ] [ PubMed ] [ Google Scholar ]
- Hawn S.E., Paul L., Thomas S., Miller S., Amstadter A.B. Stress reactivity to an electronic version of the Trier Social Stress Test: a pilot study. Front. Psychol. 2015;6:724. doi: 10.3389/fpsyg.2015.00724. [ DOI ] [ PMC free article ] [ PubMed ] [ Google Scholar ]
- Helminen E.C., Morton M.L., Wang Q., Felver J.C. A meta-analysis of cortisol reactivity to the Trier Social Stress Test in virtual environments. Psychoneuroendocrinology. 2019;110 doi: 10.1016/j.psyneuen.2019.104437. [ DOI ] [ PubMed ] [ Google Scholar ]
- Hernando D., Roca S., Sancho J., Alesanco Á., Bailón R. Validation of the apple watch for heart rate variability measurements during relax and mental stress in healthy subjects. Sensors (Basel) 2018;18 doi: 10.3390/s18082619. [ DOI ] [ PMC free article ] [ PubMed ] [ Google Scholar ]
- Herzberg M.P., Hunt R.H., Thomas K.M., Gunnar M.R. Differential brain activity as a function of social evaluative stress in early adolescence: brain function and salivary cortisol. Dev. Psychopathol. 2020;32:1926–1936. doi: 10.1017/S095457942000125X. [ DOI ] [ PMC free article ] [ PubMed ] [ Google Scholar ]
- Het S., Rohleder N., Schoofs D., Kirschbaum C., Wolf O.T. Neuroendocrine and psychometric evaluation of a placebo version of the ‘Trier Social Stress Test’. Psychoneuroendocrinology. 2009;34:1075–1086. doi: 10.1016/j.psyneuen.2009.02.008. [ DOI ] [ PubMed ] [ Google Scholar ]
- Hines E.A., Brown G.E. A standard stimulus for measuringvasomotor reactions: its application in the study of hyperten-sion. Proc. Staff Meet. Mayo Clin. 1932;7:332. [ Google Scholar ]
- Holman E.A., Thompson R.R., Garfin D.R., Silver R.C. The unfolding COVID-19 pandemic: a probability-based, nationally representative study of mental health in the United States. Sci. Adv. 2020;6 doi: 10.1126/sciadv.abd5390. [ DOI ] [ PMC free article ] [ PubMed ] [ Google Scholar ]
- Huckins J.F., daSilva A.W., Wang W., Hedlund E., Rogers C., Nepal S.K., Wu J., Obuchi M., Murphy E.I., Meyer M.L., Wagner D.D., Holtzheimer P.E., Campbell A.T. Mental health and behavior of college students during the early phases of the COVID-19 pandemic: longitudinal smartphone and ecological momentary assessment study. J. Med. Internet Res. 2020;22 doi: 10.2196/20185. [ DOI ] [ PMC free article ] [ PubMed ] [ Google Scholar ]
- Huizink A.C., Robles de Medina P.G., Mulder E.J.H., Visser G.H.A., Buitelaar J.K. Stress during pregnancy is associated with developmental outcome in infancy. J. Child Psychol. Psychiatry. 2003;44:810–818. doi: 10.1111/1469-7610.00166. [ DOI ] [ PubMed ] [ Google Scholar ]
- Husain F., Anderson M., Lopes Cardozo B., Becknell K., Blanton C., Araki D., Vithana E.K. Prevalence of war-related mental health conditions and association with displacement status in postwar Jaffna District, Sri Lanka. JAMA. 2011;306:522–531. doi: 10.1001/jama.2011.1052. [ DOI ] [ PubMed ] [ Google Scholar ]
- Ingram J., Maciejewski G., Hand C.J. Changes in diet, sleep, and physical activity are associated with differences in negative mood during COVID-19 lockdown. Front. Psychol. 2020;11 doi: 10.3389/fpsyg.2020.588604. [ DOI ] [ PMC free article ] [ PubMed ] [ Google Scholar ]
- Ironson G., Wynings C., Schneiderman N., Baum A., Rodriguez M., Greenwood D., Benight C., Antoni M., LaPerriere A., Huang H.S., Klimas N., Fletcher M.A. Posttraumatic stress symptoms, intrusive thoughts, loss, and immune function after Hurricane Andrew. Psychosom. Med. 1997;59:128–141. doi: 10.1097/00006842-199703000-00003. [ DOI ] [ PubMed ] [ Google Scholar ]
- Iversen R.T.B. The mental health of seafarers. Int. Marit. Health. 2012;63:78–89. [ PubMed ] [ Google Scholar ]
- Jentsch V.L., Wolf O.T. The impact of emotion regulation on cardiovascular, neuroendocrine and psychological stress responses. Biol. Psychol. 2020;154 doi: 10.1016/j.biopsycho.2020.107893. [ DOI ] [ PubMed ] [ Google Scholar ]
- Jeon H.-S., Dunkle R.E. Stress and depression among the oldest-old: a longitudinal analysis. Res. Aging. 2009;31:661–687. doi: 10.1177/0164027509343541. [ DOI ] [ PMC free article ] [ PubMed ] [ Google Scholar ]
- Jeong H., Yim H.W., Song Y.-J., Ki M., Min J.-A., Cho J., Chae J.-H. Mental health status of people isolated due to Middle East Respiratory Syndrome. Epidemiol. Health. 2016;38 doi: 10.4178/epih.e2016048. [ DOI ] [ PMC free article ] [ PubMed ] [ Google Scholar ]
- Joëls M., Baram T.Z. The neuro-symphony of stress. Nat. Rev. Neurosci. 2009;10:459–466. doi: 10.1038/nrn2632. [ DOI ] [ PMC free article ] [ PubMed ] [ Google Scholar ]
- Johnston D.W., Tuomisto M.T., Patching G.R. The relationship between cardiac reactivity in the laboratory and in real life. Health Psychol. 2008;27:34–42. doi: 10.1037/0278-6133.27.1.34. [ DOI ] [ PubMed ] [ Google Scholar ]
- Jönsson P., Wallergård M., Osterberg K., Hansen A.M., Johansson G., Karlson B. Cardiovascular and cortisol reactivity and habituation to a virtual reality version of the Trier Social Stress Test: a pilot study. Psychoneuroendocrinology. 2010;35:1397–1403. doi: 10.1016/j.psyneuen.2010.04.003. [ DOI ] [ PubMed ] [ Google Scholar ]
- Kelly A.L., Berthelsen D.C. Preschool teachers’ experiences of stress. Teach. Teach. Educ. 1995;11:345–357. doi: 10.1016/0742-051X(94)00038-8. [ DOI ] [ Google Scholar ]
- Kelly O., Matheson K., Martinez A., Merali Z., Anisman H. Psychosocial stress evoked by a virtual audience: relation to neuroendocrine activity. Cyberpsychol. Behav. 2007;10:655–662. doi: 10.1089/cpb.2007.9973. [ DOI ] [ PubMed ] [ Google Scholar ]
- Kemeny M.E., Gruenewald T.L., Dickerson S.S. Shame as the emotional response to threat to the social self: implications for behavior, physiology, and health. Psychol. Inq. 2004;15:153–160. [ Google Scholar ]
- Khan K.S., Mamun M.A., Griffiths M.D., Ullah I. The mental health impact of the COVID-19 pandemic across different cohorts. Int. J. Ment. Health Addict. 2020:1–7. doi: 10.1007/s11469-020-00367-0. [ DOI ] [ PMC free article ] [ PubMed ] [ Google Scholar ]
- Kickbusch I., Leung G.M., Bhutta Z.A., Matsoso M.P., Ihekweazu C., Abbasi K. Covid-19: how a virus is turning the world upside down. BMJ. 2020;369:m1336. doi: 10.1136/bmj.m1336. [ DOI ] [ PubMed ] [ Google Scholar ]
- Kim H., Lee S., Lee S., Hong S., Kang H., Kim N. Depression prediction by using ecological momentary assessment, actiwatch data, and machine learning: observational study on older adults living alone. JMIR Mhealth Uhealth. 2019;7 doi: 10.2196/14149. [ DOI ] [ PMC free article ] [ PubMed ] [ Google Scholar ]
- Kirschbaum C. Why we need an online version of the Trier Social Stress Test. Psychoneuroendocrinology. 2021;125 doi: 10.1016/j.psyneuen.2021.105129. [ DOI ] [ PMC free article ] [ PubMed ] [ Google Scholar ]
- Kirschbaum C., Pirke K.M., Hellhammer D.H. The ‘Trier Social Stress Test’—a tool for investigating psychobiological stress responses in a laboratory setting. Neuropsychobiology. 1993;28:76–81. doi: 10.1159/000119004. [ DOI ] [ PubMed ] [ Google Scholar ]
- Kirschbaum C., Pruessner J.C., Stone A.A., Federenko I., Gaab J., Lintz D., et al. Persistent high cortisol responses to repeated psychological stress in a subpopulation of healthy men. Psychosom. Med. 1995;57:468–474. doi: 10.1097/00006842-199509000-00009. [ DOI ] [ PubMed ] [ Google Scholar ]
- Kluge A., Termer A. Human-centered design (HCD) of a fault-finding application for mobile devices and its impact on the reduction of time in fault diagnosis in the manufacturing industry. Appl. Ergon. 2017;59:170–181. doi: 10.1016/j.apergo.2016.08.030. [ DOI ] [ PubMed ] [ Google Scholar ]
- Kluge A., Nazir S., Manca D. Advanced applications in process control and training needs of field and control room operators. IIE Trans. Occup. Ergon. Hm. Factors. 2014;2:121–136. doi: 10.1080/21577323.2014.920437. [ DOI ] [ Google Scholar ]
- Kluge A., Silbert M., Wiemers U.S., Frank B., Wolf O.T. Retention of a standard operating procedure under the influence of social stress and refresher training in a simulated process control task. Ergonomics. 2019;62:361–375. doi: 10.1080/00140139.2018.1542036. [ DOI ] [ PubMed ] [ Google Scholar ]
- Kothgassner O.D., Felnhofer A., Hlavacs H., Beutl L., Palme R., Kryspin-Exner I., Glenk L.M. Salivary cortisol and cardiovascular reactivity to a public speaking task in a virtual and real-life environment. Comput. Human Behav. 2016;62:124–135. doi: 10.1016/j.chb.2016.03.081. [ DOI ] [ Google Scholar ]
- Kowal M., Coll-Martín T., Ikizer G., Rasmussen J., Eichel K., Studzińska A., Koszałkowska K., Karwowski M., Najmussaqib A., Pankowski D., Lieberoth A., Ahmed O. Who is the most stressed during the COVID-19 pandemic? Data from 26 countries and areas. Appl. Psychol. Health Well. Being. 2020;12:946–966. doi: 10.1111/aphw.12234. [ DOI ] [ PMC free article ] [ PubMed ] [ Google Scholar ]
- Krijn M., Emmelkamp P.M.G., Biemond R., de Wilde Ligny C., Schuemie M.J., van der Mast C.A. Treatment of acrophobia in virtual reality: the role of immersion and presence. Behav. Res. Ther. 2004;42:229–239. doi: 10.1016/S0005-7967(03)00139-6. [ DOI ] [ PubMed ] [ Google Scholar ]
- Kudielka B.M., Wüst S. Human models in acute and chronic stress: assessing determinants of individual hypothalamus–pituitary–adrenal axis activity and reactivity. Stress. 2010;13:1–14. doi: 10.3109/10253890902874913. [ DOI ] [ PubMed ] [ Google Scholar ]
- Kudielka B.M., Hellhammer D.H., Wüst S. Why do we respond so differently? Reviewing determinants of human salivary cortisol responses to challenge. Psychoneuroendocrinology. 2009;34:2–18. doi: 10.1016/j.psyneuen.2008.10.004. [ DOI ] [ PubMed ] [ Google Scholar ]
- Kudielka B.M., Gierens A., Hellhammer D.H., Wüst S., Schlotz W. Salivary cortisol in ambulatory assessment—some dos, some don’ts, and some open questions. Psychosom. Med. 2012;74:418–431. doi: 10.1097/PSY.0b013e31825434c7. [ DOI ] [ PubMed ] [ Google Scholar ]
- Kumari S., Mahla R.S. Stay-at-home isolation modulates sleep pattern associating with depression and anxiety mood disorders. J. Clin. Sleep Med. 2021;17:343–344. doi: 10.5664/jcsm.8908. [ DOI ] [ PMC free article ] [ PubMed ] [ Google Scholar ]
- Kwon J.H., Powell J., Chalmers A. How level of realism influences anxiety in virtual reality environments for a job interview. Int. J. Hum. Stud. 2013;71:978–987. doi: 10.1016/j.ijhcs.2013.07.003. [ DOI ] [ Google Scholar ]
- Labuschagne I., Grace C., Rendell P., Terrett G., Heinrichs M. An introductory guide to conducting the trier social stress test. Neurosci. Biobehav. Rev. 2019;107:686–695. doi: 10.1016/j.neubiorev.2019.09.032. [ DOI ] [ PubMed ] [ Google Scholar ]
- Lam J.C.W., Shields G.S., Trainor B.C., Slavich G.M., Yonelinas A.P. Greater lifetime stress exposure predicts blunted cortisol but heightened DHEA responses to acute stress. Stress Health. 2019;35:15–26. doi: 10.1002/smi.2835. [ DOI ] [ PMC free article ] [ PubMed ] [ Google Scholar ]
- Lam K.H., van Oirschot P., den Teuling B., Hulst H.E., de Jong B.A., Uitdehaag B., de Groot V., Killestein J. Reliability, construct and concurrent validity of a smartphone-based cognition test in multiple sclerosis. Mult. Scler. 2021 doi: 10.1177/13524585211018103. 13524585211018103. [ DOI ] [ PMC free article ] [ PubMed ] [ Google Scholar ]
- Laugharne J., Janca A., Widiger T. Posttraumatic stress disorder and terrorism: 5 years after 9/11. Curr. Opin. Psychiatry. 2007;20:36–41. doi: 10.1097/YCO.0b013e328010dc2c. [ DOI ] [ PubMed ] [ Google Scholar ]
- Lazarus R.S. From psychological stress to the emotions: a history of changing outlooks. Annu. Rev. Psychol. 1993;44:1–21. doi: 10.1146/annurev.ps.44.020193.000245. [ DOI ] [ PubMed ] [ Google Scholar ]
- Le J.T., Watson P., Begg D., Albertella L., Le Pelley M.E. Physiological and subjective validation of a novel stress procedure: the Simple Singing Stress Procedure. Behav. Res. Methods. 2020 doi: 10.3758/s13428-020-01505-1. [ DOI ] [ PubMed ] [ Google Scholar ]
- Lederbogen F., Kirsch P., Haddad L., Streit F., Tost H., Schuch P., Wüst S., Pruessner J.C., Rietschel M., Deuschle M., Meyer-Lindenberg A. City living and urban upbringing affect neural social stress processing in humans. Nature. 2011;474:498–501. doi: 10.1038/nature10190. [ DOI ] [ PubMed ] [ Google Scholar ]
- Lejuez C.W., Kahler C.W., Brown R.A. A modified computer version of the Paced Auditory Serial Addition Task (PASAT) as a laboratory-based stressor. Behav. Ther. 2003 [ Google Scholar ]
- Liston C., McEwen B.S., Casey B.J. Psychosocial stress reversibly disrupts prefrontal processing and attentional control: the retreat from racial justice in American thought and policy. Proc. Natl. Acad. Sci. U. S. A. 2009;106:912–917. doi: 10.1073/pnas.0807041106. [ DOI ] [ PMC free article ] [ PubMed ] [ Google Scholar ]
- Liu Q., Zhang W. Sex differences in stress reactivity to the trier social stress test in virtual reality. Psychol. Res. Behav. Manag. 2020;13:859–869. doi: 10.2147/PRBM.S268039. [ DOI ] [ PMC free article ] [ PubMed ] [ Google Scholar ]
- Lohse K.R., Hilderman C.G.E., Cheung K.L., Tatla S., van der Loos H.F.M. Virtual reality therapy for adults post-stroke: a systematic review and meta-analysis exploring virtual environments and commercial games in therapy. PLoS One. 2014;9 doi: 10.1371/journal.pone.0093318. [ DOI ] [ PMC free article ] [ PubMed ] [ Google Scholar ]
- Loken E.K., Hettema J.M., Aggen S.H., Kendler K.S. The structure of genetic and environmental risk factors for fears and phobias. Psychol. Med. 2014;44:2375–2384. doi: 10.1017/S0033291713003012. [ DOI ] [ PMC free article ] [ PubMed ] [ Google Scholar ]
- Lowell A., Suarez-Jimenez B., Helpman L., Zhu X., Durosky A., Hilburn A., Schneier F., Gross R., Neria Y. 9/11-related PTSD among highly exposed populations: a systematic review 15 years after the attack. Psychol. Med. 2018;48:537–553. doi: 10.1017/S0033291717002033. [ DOI ] [ PMC free article ] [ PubMed ] [ Google Scholar ]
- Lu Y., Li X., Geng D., Mei N., Wu P.-Y., Huang C.-C., Jia T., Zhao Y., Wang D., Xiao A., Yin B. Cerebral micro-structural changes in COVID-19 patients - an MRI-based 3-month follow-up study. EClinicalMedicine. 2020;25 doi: 10.1016/j.eclinm.2020.100484. [ DOI ] [ PMC free article ] [ PubMed ] [ Google Scholar ]
- Lucas B.d.L., Barbosa T.d.S., Castelo P.M., Gavião M.B.D. Salivary alpha-amylase and hormones levels of young adults with different body composition. J. Texture Stud. 2019;50:45–52. doi: 10.1111/jtxs.12384. [ DOI ] [ PubMed ] [ Google Scholar ]
- Lund C., de Silva M., Plagerson S., Cooper S., Chisholm D., Das J., Knapp M., Patel V. Poverty and mental disorders: breaking the cycle in low-income and middle-income countries. Lancet. 2011;378:1502–1514. doi: 10.1016/S0140-6736(11)60754-X. [ DOI ] [ PubMed ] [ Google Scholar ]
- Mahmud M.S., Talukder M.U., Rahman S.M. Does ‘Fear of COVID-19’ trigger future career anxiety? An empirical investigation considering depression from COVID-19 as a mediator. Int. J. Soc. Psychiatry. 2021;67:35–45. doi: 10.1177/0020764020935488. [ DOI ] [ PMC free article ] [ PubMed ] [ Google Scholar ]
- Margerison-Zilko C., Goldman-Mellor S., Falconi A., Downing J. Health impacts of the great recession: a critical review. Curr. Epidemiol. Rep. 2016;3:81–91. doi: 10.1007/s40471-016-0068-6. [ DOI ] [ PMC free article ] [ PubMed ] [ Google Scholar ]
- Mason J.W. A review of psychoendocrine research on the pituitary-adrenal cortical system. Psychosom. Med. 1968;30:576–607. doi: 10.1097/00006842-196809000-00020. [ DOI ] [ PubMed ] [ Google Scholar ]
- Matthews K.A., Gump B.B., Owens J.F. Chronic stress influences cardiovascular and neuroendocrine responses during acute stress and recovery, especially in men. Health Psychol. 2001;20:403–410. doi: 10.1037/0278-6133.20.6.403. [ DOI ] [ PubMed ] [ Google Scholar ]
- McEwen B.S. Protective and damaging effects of stress mediators. N. Engl. J. Med. 1998;338:171–179. doi: 10.1056/nejm199801153380307. [ DOI ] [ PubMed ] [ Google Scholar ]
- McVicar A. Workplace stress in nursing: a literature review. J. Adv. Nurs. 2003;44:633–642. doi: 10.1046/j.0309-2402.2003.02853.x. [ DOI ] [ PubMed ] [ Google Scholar ]
- Meir Drexler S., Merz C.J., Jentsch V.L., Wolf O.T. How stress and glucocorticoids timing-dependently affect extinction and relapse. Neurosci. Biobehav. Rev. 2019;98:145–153. doi: 10.1016/j.neubiorev.2018.12.029. [ DOI ] [ PubMed ] [ Google Scholar ]
- Merz C.J., Hagedorn B., Wolf O.T. An oral presentation causes stress and memory impairments. Psychoneuroendocrinology. 2019;104:1–6. doi: 10.1016/j.psyneuen.2019.02.010. [ DOI ] [ PubMed ] [ Google Scholar ]
- Michaud K., Matheson K., Kelly O., Anisman H. Impact of stressors in a natural context on release of cortisol in healthy adult humans: a meta-analysis. Stress. 2008;11:177–197. doi: 10.1080/10253890701727874. [ DOI ] [ PubMed ] [ Google Scholar ]
- Miller G. The smartphone psychology manifesto. Perspect. Psychol. Sci. 2012;7:221–237. doi: 10.1177/1745691612441215. [ DOI ] [ PubMed ] [ Google Scholar ]
- Moccia M., Lanzillo R., Brescia Morra V., Bonavita S., Tedeschi G., Leocani L., Lavorgna L. Assessing disability and relapses in multiple sclerosis on tele-neurology. Neurol. Sci. 2020;41:1369–1371. doi: 10.1007/s10072-020-04470-x. [ DOI ] [ PMC free article ] [ PubMed ] [ Google Scholar ]
- Montero-López E., Santos-Ruiz A., García-Ríos M.C., Rodríguez-Blázquez R., Pérez-García M., Peralta-Ramírez M.I. A virtual reality approach to the Trier Social Stress Test: contrasting two distinct protocols. Behav. Res. Methods. 2016;48:223–232. doi: 10.3758/s13428-015-0565-4. [ DOI ] [ PubMed ] [ Google Scholar ]
- Mühlberger A., Herrmann M.J., Wiedemann G., Ellgring H., Pauli P. Repeated exposure of flight phobics to flights in virtual reality. Behav. Res. Ther. 2001;39:1033–1050. doi: 10.1016/S0005-7967(00)00076-0. [ DOI ] [ PubMed ] [ Google Scholar ]
- Musliner K.L., Seifuddin F., Judy J.A., Pirooznia M., Goes F.S., Zandi P.P. Polygenic risk, stressful life events and depressive symptoms in older adults: a polygenic score analysis. Psychol. Med. 2015;45:1709–1720. doi: 10.1017/S0033291714002839. [ DOI ] [ PMC free article ] [ PubMed ] [ Google Scholar ]
- Myin-Germeys I., Oorschot M., Collip D., Lataster J., Delespaul P., van Os J. Experience sampling research in psychopathology: opening the black box of daily life. Psychol. Med. 2009;39:1533–1547. doi: 10.1017/S0033291708004947. [ DOI ] [ PubMed ] [ Google Scholar ]
- Narvaez Linares N.F., Charron V., Ouimet A.J., Labelle P.R., Plamondon H.A. A systematic review of the Trier Social Stress Test methodology: issues in promoting study comparison and replicable research. Neurobiol. Stress. 2020;13 doi: 10.1016/j.ynstr.2020.100235. [ DOI ] [ PMC free article ] [ PubMed ] [ Google Scholar ]
- Nater U.M., Rohleder N. Salivary alpha-amylase as a non-invasive biomarker for the sympathetic nervous system: current state of research. Psychoneuroendocrinology. 2009;34:486–496. doi: 10.1016/j.psyneuen.2009.01.014. [ DOI ] [ PubMed ] [ Google Scholar ]
- Neria Y., DiGrande L., Adams B.G. Posttraumatic stress disorder following the September 11, 2001, terrorist attacks: a review of the literature among highly exposed populations. Am. Psychol. 2011;66:429–446. doi: 10.1037/a0024791. [ DOI ] [ PMC free article ] [ PubMed ] [ Google Scholar ]
- Njenga F., Nguthi A., Kangethe R. War and mental disorders in Africa. World Psychiatry. 2006;5:38–39. [ PMC free article ] [ PubMed ] [ Google Scholar ]
- Noack H., Nolte L., Nieratschker V., Habel U., Derntl B. Imaging stress: an overview of stress induction methods in the MR scanner. J. Neural Transm. (Vienna) 2019;126:1187–1202. doi: 10.1007/s00702-018-01965-y. [ DOI ] [ PubMed ] [ Google Scholar ]
- O’Donnell K., Kammerer M., O’Reilly R., Taylor A., Glover V. Salivary alpha-amylase stability, diurnal profile and lack of response to the cold hand test in young women. Stress. 2009;12:549–554. doi: 10.3109/10253890902822664. [ DOI ] [ PubMed ] [ Google Scholar ]
- Ocklenburg S., Ströckens F., Bless J.J., Hugdahl K., Westerhausen R., Manns M. Investigating heritability of laterality and cognitive control in speech perception. Brain Cogn. 2016;109:34–39. doi: 10.1016/j.bandc.2016.09.003. [ DOI ] [ PubMed ] [ Google Scholar ]
- Oldenburg M., Jensen H.-J., Latza U., Baur X. Seafaring stressors aboard merchant and passenger ships. Int. J. Public Health. 2009;54:96–105. doi: 10.1007/s00038-009-7067-z. [ DOI ] [ PubMed ] [ Google Scholar ]
- Oldenburg M., Jensen H.-J., Wegner R. Burnout syndrome in seafarers in the merchant marine service. Int. Arch. Occup. Environ. Health. 2013;86:407–416. doi: 10.1007/s00420-012-0771-7. [ DOI ] [ PubMed ] [ Google Scholar ]
- Ornstein K.A., Leff B., Covinsky K.E., Ritchie C.S., Federman A.D., Roberts L., Kelley A.S., Siu A.L., Szanton S.L. Epidemiology of the homebound population in the United States. JAMA Intern. Med. 2015;175:1180–1186. doi: 10.1001/jamainternmed.2015.1849. [ DOI ] [ PMC free article ] [ PubMed ] [ Google Scholar ]
- Osmanovic-Thunström A., Mossello E., Åkerstedt T., Fratiglioni L., Wang H.-X. Do levels of perceived stress increase with increasing age after age 65? A population-based study. Age Ageing. 2015;44:828–834. doi: 10.1093/ageing/afv078. [ DOI ] [ PubMed ] [ Google Scholar ]
- Ottenweller J.E., Natelson B.H., Pitman D.L., Drastal S.D. Adrenocortical and behavioral responses to repeated stressors: toward an animal model of chronic stress and stress-related mental illness. Biol. Psychiatry. 1989;26:829–841. doi: 10.1016/0006-3223(89)90123-6. [ DOI ] [ PubMed ] [ Google Scholar ]
- Owens M.E., Beidel D.C. Can virtual reality effectively elicit distress associated with social anxiety disorder? J. Psychopathol. Behav. Assess. 2015;37:296–305. doi: 10.1007/s10862-014-9454-x. [ DOI ] [ Google Scholar ]
- Parker A.J., Woodhead Z.V.J., Thompson P.A., Bishop D.V.M. Assessing the reliability of an online behavioural laterality battery: a pre-registered study. Laterality. 2020:1–39. doi: 10.1080/1357650X.2020.1859526. [ DOI ] [ PubMed ] [ Google Scholar ]
- Parsons T.D., Rizzo A.A. Affective outcomes of virtual reality exposure therapy for anxiety and specific phobias: a meta-analysis. J. Behav. Ther. Exp. Psychiatry. 2008;39:250–261. doi: 10.1016/j.jbtep.2007.07.007. [ DOI ] [ PubMed ] [ Google Scholar ]
- Pavlova M.A., Sokolov A.A. Reading covered faces. Cereb. Cortex. 2021 doi: 10.1093/cercor/bhab311. bhab311. [ DOI ] [ PubMed ] [ Google Scholar ]
- Pfefferbaum B., North C.S. Mental health and the Covid-19 pandemic. N. Engl. J. Med. 2020;383:510–512. doi: 10.1056/NEJMp2008017. [ DOI ] [ PubMed ] [ Google Scholar ]
- Pithers R.T., Soden R. Scottish and Australian teacher stress and strain: a comparative study. Br. J. Educ. Psychol. 1998;68(Pt 2):269–279. doi: 10.1111/j.2044-8279.1998.tb01289.x. [ DOI ] [ PubMed ] [ Google Scholar ]
- Priebe S., Bogic M., Ajdukovic D., Franciskovic T., Galeazzi G.M., Kucukalic A., et al. Mental disorders following war in the Balkans – a community based study on long-term outcomes in five countries. Arch. Gen. Psychiatry. 2010;67:518–528. doi: 10.1001/archgenpsychiatry.2010.37. [ DOI ] [ PubMed ] [ Google Scholar ]
- Pruessner J.C., Gaab J., Hellhammer D.H., Lintz D., Schommer N., Kirschbaum C. Increasing correlations between personality traits and cortisol stress responses obtained by data aggregation. Psychoneuroendocrinology. 1997;22:615–625. doi: 10.1016/S0306-4530(97)00072-3. [ DOI ] [ PubMed ] [ Google Scholar ]
- Qiu W.Q., Dean M., Liu T., George L., Gann M., Cohen J., Bruce M.L. Physical and mental health of homebound older adults: an overlooked population. J. Am. Geriatr. Soc. 2010;58:2423–2428. doi: 10.1111/j.1532-5415.2010.03161.x. [ DOI ] [ PMC free article ] [ PubMed ] [ Google Scholar ]
- Qiu J., Shen B., Zhao M., Wang Z., Xie B., Xu Y. A nationwide survey of psychological distress among Chinese people in the COVID-19 epidemic: implications and policy recommendations. Gen Psychiatr. 2020;33 doi: 10.1136/gpsych-2020-100213. [ DOI ] [ PMC free article ] [ PubMed ] [ Google Scholar ]
- Ramirez D.A., Brodie F.L., Rose-Nussbaumer J., Ramanathan S. Anxiety in patients undergoing cataract surgery: a pre- and postoperative comparison. Clin. Ophthalmol. 2017;11:1979–1986. doi: 10.2147/OPTH.S146135. [ DOI ] [ PMC free article ] [ PubMed ] [ Google Scholar ]
- Ravesloot C., Ward B., Hargrove T., Wong J., Livingston N., Torma L., Ipsen C. Why stay home? Temporal association of pain, fatigue and depression with being at home. Disabil. Health J. 2016;9:218–225. doi: 10.1016/j.dhjo.2015.10.010. [ DOI ] [ PubMed ] [ Google Scholar ]
- Reinhardt T., Schmahl C., Wüst S., Bohus M. Salivary cortisol, heart rate, electrodermal activity and subjective stress responses to the Mannheim Multicomponent Stress Test (MMST) Psychiatry Res. 2012;198:106–111. doi: 10.1016/j.psychres.2011.12.009. [ DOI ] [ PubMed ] [ Google Scholar ]
- Rockawin D. Using innovative technology to overcome job interview anxiety. Aust. J. Career Dev. 2012;21:46–52. doi: 10.1177/103841621202100206. [ DOI ] [ Google Scholar ]
- Rohleder N., Beulen S.E., Chen E., Wolf J.M., Kirschbaum C. Stress on the dance floor: the cortisol stress response to social-evaluative threat in competitive ballroom dancers. Pers. Soc. Psychol. Bull. 2007;33:69–84. doi: 10.1177/0146167206293986. [ DOI ] [ PubMed ] [ Google Scholar ]
- Rossin-Slater M., Schnell M., Schwandt H., Trejo S., Uniat L. Local exposure to school shootings and youth antidepressant use. Proc. Natl. Acad. Sci. U. S. A. 2020;117:23484–23489. doi: 10.1073/pnas.2000804117. [ DOI ] [ PMC free article ] [ PubMed ] [ Google Scholar ]
- Salomon T., Cohen A., Barazany D., Ben-Zvi G., Botvinik-Nezer R., Gera R., Oren S., Roll D., Rozic G., Saliy A., Tik N., Tsarfati G., Tavor I., Schonberg T., Assaf Y. Brain volumetric changes in the general population following the COVID-19 outbreak and lockdown. bioRxiv. 2020 doi: 10.1101/2020.09.08.285007. [ DOI ] [ PubMed ] [ Google Scholar ]
- Sandín B., Chorot P. Stress and anxiety: diagnosis validity of anxiety disorders according to life events stress, ways of coping and physical symptoms. Psiquis: Revista de Psiquiatría, Psicología y Psicosomática. 1993;15:48–54. [ Google Scholar ]
- Sandner M., Lois G., Streit F., Zeier P., Kirsch P., Wüst S., Wessa M. Investigating individual stress reactivity: high hair cortisol predicts lower acute stress responses. Psychoneuroendocrinology. 2020;118 doi: 10.1016/j.psyneuen.2020.104660. [ DOI ] [ PubMed ] [ Google Scholar ]
- Santl J., Shiban Y., Plab A., Wüst S., Kudielka B.M., Mühlberger A. Gender differences in stress responses during a virtual reality trier social stress test. IJVR. 2019;19 doi: 10.20870/IJVR.2019.19.2.2912. [ DOI ] [ Google Scholar ]
- Schlotz W., editor. Ambulatory Psychoneuroendocrinology: Assessing Salivary Cortisol and Other Hormones in Daily Life. Guilford Press; New York, NY: 2011. [ Google Scholar ]
- Schmitz J., Kumsta R., Moser D., Güntürkün O., Ocklenburg S. KIAA0319 promoter DNA methylation predicts dichotic listening performance in forced-attention conditions. Behav. Brain Res. 2018;337:1–7. doi: 10.1016/j.bbr.2017.09.035. [ DOI ] [ PubMed ] [ Google Scholar ]
- Schwabe L., Haddad L., Schachinger H. HPA axis activation by a socially evaluated cold-pressor test. Psychoneuroendocrinology. 2008;33:890–895. doi: 10.1016/j.psyneuen.2008.03.001. [ DOI ] [ PubMed ] [ Google Scholar ]
- Seddon J.A., Rodriguez V.J., Provencher Y., Raftery-Helmer J., Hersh J., Labelle P.R., Thomassin K. Meta-analysis of the effectiveness of the Trier Social Stress Test in eliciting physiological stress responses in children and adolescents. Psychoneuroendocrinology. 2020 doi: 10.1016/j.psyneuen.2020.104582. [ DOI ] [ PubMed ] [ Google Scholar ]
- Shiban Y., Diemer J., Brandl S., Zack R., Mühlberger A., Wüst S. Trier Social Stress Test in vivo and in virtual reality: dissociation of response domains. Int. J. Psychophysiol. 2016;110:47–55. doi: 10.1016/j.ijpsycho.2016.10.008. [ DOI ] [ PubMed ] [ Google Scholar ]
- Shields G.S. Stress and cognition: a user’s guide to designing and interpreting studies. Psychoneuroendocrinology. 2020;112 doi: 10.1016/j.psyneuen.2019.104475. [ DOI ] [ PubMed ] [ Google Scholar ]
- Shields G.S., Sazma M.A., Yonelinas A.P. The effects of acute stress on core executive functions: a meta-analysis and comparison with cortisol. Neurosci. Biobehav. Rev. 2016;68:651–668. doi: 10.1016/j.neubiorev.2016.06.038. [ DOI ] [ PMC free article ] [ PubMed ] [ Google Scholar ]
- Shiffman S., Stone A.A., Hufford M.R. Ecological momentary assessment. Annu. Rev. Clin. Psychol. 2008;4:1–32. doi: 10.1146/annurev.clinpsy.3.022806.091415. [ DOI ] [ PubMed ] [ Google Scholar ]
- Shin L.M., Liberzon I. The neurocircuitry of fear, stress, and anxiety disorders. Neuropsychopharmacology. 2010;35:169–191. doi: 10.1038/npp.2009.83. [ DOI ] [ PMC free article ] [ PubMed ] [ Google Scholar ]
- Sicorello M., Dieckmann L., Moser D., Lux V., Luhmann M., Neubauer A.B., Schlotz W., Kumsta R. Highs and lows: genetic susceptibility to daily events. PLoS One. 2020;15 doi: 10.1371/journal.pone.0237001. [ DOI ] [ PMC free article ] [ PubMed ] [ Google Scholar ]
- Sieberichs S., Kluge A. Effects of short-term measures to mitigate fatigue risks in aviation. Aviat. Psychol. Appl. Hum. Factors. 2018;8:86–92. doi: 10.1027/2192-0923/a000143. [ DOI ] [ Google Scholar ]
- Slater M. Place illusion and plausibility can lead to realistic behaviour in immersive virtual environments. Philos. Trans. R. Soc. Lond. B Biol. Sci. 2009;364:3549–3557. doi: 10.1098/rstb.2009.0138. [ DOI ] [ PMC free article ] [ PubMed ] [ Google Scholar ]
- Slater M., Pertaub D.-P., Barker C., Clark D.M. An experimental study on fear of public speaking using a virtual environment. Cyberpsychol. Behav. 2006;9:627–633. doi: 10.1089/cpb.2006.9.627. [ DOI ] [ PubMed ] [ Google Scholar ]
- Smeets T., Cornelisse S., Quaedflieg C.W.E.M., Meyer T., Jelicic M., Merckelbach H. Introducing the Maastricht Acute Stress Test (MAST): a quick and non-invasive approach to elicit robust autonomic and glucocorticoid stress responses. Psychoneuroendocrinology. 2012;37:1998–2008. doi: 10.1016/j.psyneuen.2012.04.012. [ DOI ] [ PubMed ] [ Google Scholar ]
- Smith A. The Symbol Digit Modalities Test: a neuropsychologic test for economic screening of learning and other cerebral disorders. Learn. Disord. 1968;3:83–91. [ Google Scholar ]
- Smith A. Western Psychological Services; Los Angeles: 1982. Symbol Digits Modalities Test. [ Google Scholar ]
- Smith K.E., Leitzke B.T., Pollak S.D. Youths’ processing of emotion information: responses to chronic and video-based laboratory stress. Psychoneuroendocrinology. 2020;122 doi: 10.1016/j.psyneuen.2020.104873. [ DOI ] [ PMC free article ] [ PubMed ] [ Google Scholar ]
- Somma F., Bartolomeo P., Vallone F., Argiuolo A., Cerrato A., Miglino O., et al. Further to the left: stress-induced increase of spatial pseudoneglect during the COVID-19 lockdown. Front. Psychol. 2021;12 doi: 10.3389/fpsyg.2021.573846. [ DOI ] [ PMC free article ] [ PubMed ] [ Google Scholar ]
- Spoorthy M.S., Pratapa S.K., Mahant S. Mental health problems faced by healthcare workers due to the COVID-19 pandemic-a review. Asian J. Psychiatr. 2020;51 doi: 10.1016/j.ajp.2020.102119. [ DOI ] [ PMC free article ] [ PubMed ] [ Google Scholar ]
- Stafford M., Chandola T., Marmot M. Association between fear of crime and mental health and physical functioning. Am. J. Public Health. 2007;97:2076–2081. doi: 10.2105/AJPH.2006.097154. [ DOI ] [ PMC free article ] [ PubMed ] [ Google Scholar ]
- Standard W., Zielasko D., von Dawans B., Domes G., Weyers B. In: GI VR / AR Workshop. Gesellschaft für Informatik e.V. Weyers B., Lürig C., Zielasko D. (Hrsg.), editors. 2020. Open TSST VR: trier social stress test in virtual reality. [ DOI ] [ Google Scholar ]
- Starcke K., Brand M. Effects of stress on decisions under uncertainty: a meta-analysis. Psychol. Bull. 2016;142:909–933. doi: 10.1037/bul0000060. [ DOI ] [ PubMed ] [ Google Scholar ]
- Steptoe A., Gibson E.L., Hamer M., Wardle J. Neuroendocrine and cardiovascular correlates of positive affect measured by ecological momentary assessment and by questionnaire. Psychoneuroendocrinology. 2007;32:56–64. doi: 10.1016/j.psyneuen.2006.10.001. [ DOI ] [ PubMed ] [ Google Scholar ]
- Streit F., Haddad L., Paul T., Frank J., Schäfer A., Nikitopoulos J., Akdeniz C., Lederbogen F., Treutlein J., Witt S.H., Meyer-Lindenberg A., Rietschel M., Kirsch P., Wüst S. A functional variant in the neuropeptide S receptor 1 gene moderates the influence of urban upbringing on stress processing in the amygdala. Stress. 2014;17:352–361. doi: 10.3109/10253890.2014.921903. [ DOI ] [ PubMed ] [ Google Scholar ]
- Summerfield D. War and mental health: a brief overview. BMJ. 2000;321:232–235. doi: 10.1136/bmj.321.7255.232. [ DOI ] [ PMC free article ] [ PubMed ] [ Google Scholar ]
- Tang W., Hu T., Hu B., Jin C., Wang G., Xie C., Chen S., Xu J. Prevalence and correlates of PTSD and depressive symptoms one month after the outbreak of the COVID-19 epidemic in a sample of home-quarantined Chinese university students. J. Affect. Disord. 2020;274:1–7. doi: 10.1016/j.jad.2020.05.009. [ DOI ] [ PMC free article ] [ PubMed ] [ Google Scholar ]
- Thompson K.N., Phillips L.J., Komesaroff P., Yuen H.P., Wood S.J., Pantelis C., Velakoulis D., Yung A.R., McGorry P.D. Stress and HPA-axis functioning in young people at ultra high risk for psychosis. J. Psychiatr. Res. 2007;41:561–569. doi: 10.1016/j.jpsychires.2006.05.010. [ DOI ] [ PubMed ] [ Google Scholar ]
- Tolep M.R., Dougherty L.R. The conundrum of the laboratory: challenges of assessing preschool-age children’s salivary cortisol reactivity. J. Psychopathol. Behav. Assess. 2014;36:350–357. doi: 10.1007/s10862-014-9410-9. [ DOI ] [ Google Scholar ]
- Torous J., Jän Myrick K., Rauseo-Ricupero N., Firth J. Digital mental health and COVID-19: using technology today to accelerate the curve on access and quality tomorrow. JMIR Ment. Health. 2020;7 doi: 10.2196/18848. [ DOI ] [ PMC free article ] [ PubMed ] [ Google Scholar ]
- Tortella-Feliu M., Botella C., Llabrés J., Bretón-López J.M., Del Amo A.R., Baños R.M., Gelabert J.M. Virtual reality versus computer-aided exposure treatments for fear of flying. Behav. Modif. 2011;35:3–30. doi: 10.1177/0145445510390801. [ DOI ] [ PubMed ] [ Google Scholar ]
- Trautmann S., Rehm J., Wittchen H.-U. The economic costs of mental disorders: do our societies react appropriately to the burden of mental disorders? EMBO Rep. 2016;17:1245–1249. doi: 10.15252/embr.201642951. [ DOI ] [ PMC free article ] [ PubMed ] [ Google Scholar ]
- Turner J.R., Girdler S.S., Sherwood A., Light K.C. Cardiovascular responses to behavioral stressors: laboratory-field generalization and inter-task consistency. J. Psychosom. Res. 1990;34:581–589. doi: 10.1016/0022-3999(90)90033-Z. [ DOI ] [ PubMed ] [ Google Scholar ]
- van Doornen L.J.P., van Blokland R.W. The relationship between cardiovascular and catecholamine reactions to laboratory and real-life stress. Psychophysiology. 1992;29:173–181. doi: 10.1111/j.1469-8986.1992.tb01682.x. [ DOI ] [ PubMed ] [ Google Scholar ]
- van Os J., Linscott R.J., Myin-Germeys I., Delespaul P., Krabbendam L. A systematic review and meta-analysis of the psychosis continuum: evidence for a psychosis proneness-persistence-impairment model of psychotic disorder. Psychol. Med. 2009;39:179–195. doi: 10.1017/S0033291708003814. [ DOI ] [ PubMed ] [ Google Scholar ]
- von Dawans B., Kirschbaum C., Heinrichs M. The Trier Social Stress Test for Groups (TSST-G): a new research tool for controlled simultaneous social stress exposure in a group format. Psychoneuroendocrinology. 2011;36:514–522. doi: 10.1016/j.psyneuen.2010.08.004. [ DOI ] [ PubMed ] [ Google Scholar ]
- von Dawans B., Strojny J., Domes G. The effects of acute stress and stress hormones on social cognition and behavior: current state of research and future directions. Neurosci. Biobehav. Rev. 2021;121:75–88. doi: 10.1016/j.neubiorev.2020.11.026. [ DOI ] [ PubMed ] [ Google Scholar ]
- Vors O., Marqueste T., Mascret N. The Trier social stress test and the Trier social stress test for groups: qualitative investigations. PLoS One. 2018;13 doi: 10.1371/journal.pone.0195722. [ DOI ] [ PMC free article ] [ PubMed ] [ Google Scholar ]
- Wang R., Chen F., Chen Z., Li T., Harari G., Tignor S., Zhou X., Ben-Zeev D., Campbell A.T. Studentlife: assessing mental health, academic performance and behavioral trends of college students using smartphones. Proceedings of the 2014 ACM International Joint Conference on Pervasive and Ubiquitous Computing. UbiComp ‘14: The 2014 ACM Conference on Ubiquitous Computing, Seattle Washington. September 13–17, 2014; ACM, New York, NY, USA; 2014. pp. 3–14. [ DOI ] [ Google Scholar ]
- Wang M.-C., Vinall-Collier K., Csikar J., Douglas G. A qualitative study of patients’ views of techniques to reduce dental anxiety. J. Dent. 2017;66:45–51. doi: 10.1016/j.jdent.2017.08.012. [ DOI ] [ PubMed ] [ Google Scholar ]
- Westenberg C.L., Miers A.C., Sumter S.R., Kallen V.L., van Pelt J., Blöte A.W. A prepared speech in front of a pre-recorded audience: subjective, physiological, and neuroendocrine responses to the Leiden Public Speaking Task. Biol. Psychol. 2009;82:116–124. doi: 10.1016/j.biopsycho.2009.06.005. [ DOI ] [ PubMed ] [ Google Scholar ]
- Wisetborisut A., Angkurawaranon C., Jiraporncharoen W., Uaphanthasath R., Wiwatanadate P. Shift work and burnout among health care workers. Occup. Med. (Lond) 2014;64:279–286. doi: 10.1093/occmed/kqu009. [ DOI ] [ PubMed ] [ Google Scholar ]
- Wisniewski H., Henson P., Torous J. Using a smartphone app to identify clinically relevant behavior trends via symptom report, cognition scores, and exercise levels: a case series. Front. Psychiatry. 2019;10:652. doi: 10.3389/fpsyt.2019.00652. [ DOI ] [ PMC free article ] [ PubMed ] [ Google Scholar ]
- Wolf O.T. Stress and memory in humans: twelve years of progress? Brain Res. 2009;1293:142–154. doi: 10.1016/j.brainres.2009.04.013. [ DOI ] [ PubMed ] [ Google Scholar ]
- Yehuda R. Post-traumatic stress disorder. N. Engl. J. Med. 2002;346:108–114. doi: 10.1056/NEJMra012941. [ DOI ] [ PubMed ] [ Google Scholar ]
- Zänkert S., Bellingrath S., Wüst S., Kudielka B.M. HPA axis responses to psychological challenge linking stress and disease: what do we know on sources of intra- and interindividual variability? Psychoneuroendocrinology. 2019;105:86–97. doi: 10.1016/j.psyneuen.2018.10.027. [ DOI ] [ PubMed ] [ Google Scholar ]
- Zimmer P., Buttlar B., Halbeisen G., Walther E., Domes G. Virtually stressed? A refined virtual reality adaptation of the Trier Social Stress Test (TSST) induces robust endocrine responses. Psychoneuroendocrinology. 2019;101:186–192. doi: 10.1016/j.psyneuen.2018.11.010. [ DOI ] [ PubMed ] [ Google Scholar ]
- View on publisher site
- PDF (2.5 MB)
- Collections
Similar articles
Cited by other articles, links to ncbi databases.
- Download .nbib .nbib
- Format: AMA APA MLA NLM
Add to Collections
REVIEW article
A comprehensive overview on stress neurobiology: basic concepts and clinical implications.
- 1 Physiology Department, Ribeirão Preto School of Medicine, University of São Paulo, São Paulo, Brazil
- 2 Department of Neuroscience and Behavioral Sciences, Ribeirão Preto Medical School, University of São Paulo, São Paulo, Brazil
Stress is recognized as an important issue in basic and clinical neuroscience research, based upon the founding historical studies by Walter Canon and Hans Selye in the past century, when the concept of stress emerged in a biological and adaptive perspective. A lot of research after that period has expanded the knowledge in the stress field. Since then, it was discovered that the response to stressful stimuli is elaborated and triggered by the, now known, stress system , which integrates a wide diversity of brain structures that, collectively, are able to detect events and interpret them as real or potential threats. However, different types of stressors engage different brain networks, requiring a fine-tuned functional neuroanatomical processing. This integration of information from the stressor itself may result in a rapid activation of the Sympathetic-Adreno-Medullar (SAM) axis and the Hypothalamus-Pituitary-Adrenal (HPA) axis, the two major components involved in the stress response. The complexity of the stress response is not restricted to neuroanatomy or to SAM and HPA axes mediators, but also diverge according to timing and duration of stressor exposure, as well as its short- and/or long-term consequences. The identification of neuronal circuits of stress, as well as their interaction with mediator molecules over time is critical, not only for understanding the physiological stress responses, but also to understand their implications on mental health.
Introduction
Stress is recognized as an important issue in basic and clinical neuroscience research ( de Kloet et al., 2005a ; McEwen et al., 2015 ). Although this physiological phenomenon is fundamental to survival, it is also strongly related to several brain disorders including, depression, anxiety, post-traumatic stress disorder ( Ruiz et al., 2007 ; Heim et al., 2008 ; Martin et al., 2009 ; Walsh, 2011 ; Saveanu and Nemeroff, 2012 ; Nemeroff, 2016 ) accordingly to the International Classification of Diseases, 10th edition (ICD-10). Research focusing on the complexity of stress is still challenging, but several studies in animals and humans have made substantial contributions to its progress in recent years, as reviewed by Hariri and Holmes (2015) . However, it is crucial to provide more meaningful advances in the stress field by means of a translational approach, integrating basic knowledge and clinical practice.
In this review article, we briefly describe the history of stress research and highlight stress basic concepts, explore the complex neuroanatomy, in fact networks, of the stress system and its keen axes and mediators, as well as the time domains of the stress response. Lastly, we raise clinical implications from these concepts and discuss future research challenges on the stress field.
History of Stress Research
In the beginning of the last century, the North American physiologist Walter Bradfort Cannon, after a series of investigations, made a compilation on the visceral adaptive responses to different stimuli. This compilation, which has been mainly conducted in physiology laboratories at Harvard University, described bodily changes occurring in conjunction with nociceptive events, hunger and cold, exercise and strong emotions. Cannon noted that functions that establish and support the body energy reserves at rest, in face of a stressful situation, were immediately intensified or completely interrupted in order to mobilize great energy. This mobilization allows an improvement of potential escape and attack and/or defense responses. From these observations, Cannon proposed what he called the “ fighting-or-flight ” response ( Cannon, 1915 ).
A few years later, Cannon coined the term homeostasis based on the idea of milieu intérieur (internal medium) of Claude Bernard; Cannon wrote: “the blood and other fluids surrounding cells constitute the internal environment with which occur direct exchanges of each cell, and this, must always be kept with parameters suitable for cell function, regardless of changes that may be occurring in the external environment.” Cannon determined homeostasis as the ability of maintaining the physiological systems in direction of a dynamic equilibrium. The concept of homeostasis together with the fight-or-flight response has contributed for the foundation of stress research.
Hans Selye, yet as a medical student, found that patients suffering from different diseases often exhibited similar symptoms that could constitute a single syndrome. These evidences confronted him again when he was seeking for new hormones, from ovarian cow extracts at the Department of Biochemistry at McGill University in Montreal. In his study, the effects that were primarily attributed to an ovarian hormone were also observed after administration of various other extracts from several organs and toxic substances. The latter, regardless of the preparation method, induced the same changes: increased adrenal cortex size, gastrointestinal ulcers, as well as thymus and lymph nodes involution, therefore constituting the so-called “General Adaptation Syndrome” ( Selye, 1936 ).
The General Adaptation Syndrome is characterized by a set of non-specific responses, which according to Selye, develops in three stages: (1) alarm phase, characterized by acute manifestations; (2) resistance phase, when the acute manifestations disappear; and (3) exhaustion phase, when first stage reaction may be present again or when it may occur the collapse of the organism ( Selye, 1936 ).
In this context, it was designed as the basis of the stress theory and the concept of stress was built from the physical laws of Hooke. Selye was the first to define stress from a biological point of view as “ a nonspecific response of the body to any demand made upon it ” ( Selye, 1950 , 1976 ).
Scientists have discovered that the response to stressful stimuli is elaborated and triggered by the, now known, stress system , which integrates a wide diversity of brain structures that, collectively, are able to detect events and interpret them as either a real or a potential threat: stressor ( Dedovic et al., 2009 ). Many research after this period has been done and promoted great knowledge to the stress field ( McEwen et al., 2015 ).
Thus, the perception of real or potential threats leads to the release of mediating molecules. The interaction between these molecules with their corresponding receptors, in the periphery and in the brain, results in the stress response , which through physiological and behavioral mechanisms restores the body homeostasis and promotes adaptation ( de Kloet et al., 2005a ; Joëls and Baram, 2009 ; Figure 1 ).
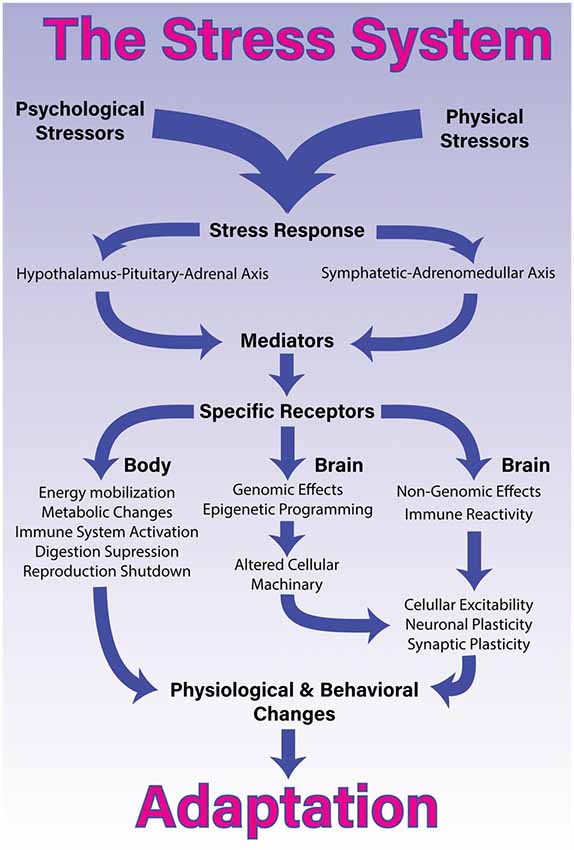
Figure 1 . The stress system. Processing and coping with stressful situations requires the engagement of complex mechanisms that integrate brain and body. The response to stressful stimuli is articulated by a wide diversity of brain structures that collectively are able to detect or interpret events as either real or potential threats (stressors) . The perception of these events as stressors involves different networks depending whether it is a physical or psychological stressor. The identification of a stressor leads to activation of two major constituents of the stress system and the release of its final mediating molecules. The sympathetic-adreno-medullar (SAM) axis, secretes noradrenaline and norepinephrine and the hypothalamus-pituitary-adrenal (HPA) axis, secretes glucocorticoids. Once these axes are activated in response to a given stressor, they will generate a coordinated response that starts within seconds and might last for days, providing quick responses enabling both, an appropriated strategy, almost immediately, and homeostasis restoration. To accomplish this, the stress response systemically promotes energy mobilization, metabolic changes, activation of the immune system and suppression of the digestive and reproductive systems. More specifically in the brain, the stress response induces short- and long-term effects through non-genomic, genomic and epigenetic mechanisms. These central effects, combined with proinflammatory signaling, lead to alterations in cellular excitability as well as synaptic and neuronal plasticity. Collectively, these body-brain effects mediate alterations in physiology and behavior that enable adaptation and survival.
In the last few decades, the initial concepts of stress have been revised. Sterling and Eyer (1988) as well as others ( McEwen and Stellar, 1993 ; Schulkin et al., 1994 ) proposed the new concept of allostasis , allostatic load and allostatic overload . These concepts would take into account the findings of the 60s, 70s and 80s from stress research, such as physiological variations in normal states and the presence of anticipatory responses to stressors ( Dallman, 2003 ; Schulkin, 2003 ). The latter authors highlighted that the new concepts of allostasis, allostatic load and allostatic overload are more biologically precise than stress, to understand a complex system of adaptation or maladaptation. In the definition of allostasis, the set-point to maintain a physiological equilibrium is constantly changed, so what is ideal for baseline situations is not necessarily ideal in stressful situations ( Koolhaas et al., 2010 , 2011 ). Moreover, the set-point to maintain physiological balance in allostasis does not work in a linear fashion, but it can be regulated in several ways ( McEwen and Karatsoreos, 2015 ). Finally, the terms “allostatic load” and “allostatic overload” consider the cumulative effects of stressors on the body in normal physiological and pathological situations, respectively ( McEwen and Karatsoreos, 2015 ). Despite the proposal of the allostasis concept, some authors argue that the concept of homeostasis was interpreted in a restricted manner, and the use of the term allostasis would be just a semantic change, since the allostatic response system remains the same biologic system studied in homeostatic response ( Dallman, 2003 ; Day, 2005 ). In fact, the proposed concepts of allostasis still offer little help to the understanding of stress, and rather than clarifying, brought several misunderstandings to the stress neurobiology ( Davies, 2016 ). At last, the necessity of the allostasis concepts is questioned with indications that it is not necessary or desirable at this point on stress research ( Dallman, 2003 ). In general, the concepts of stress, stressor and stress response are still widely used and accepted by the scientific community, and accordingly we adopt them in the current review.
Neuroanatomy
The stress response involves an efficient, evolutionarily-conserved and complex system, with modulation in several levels of the central nervous system (CNS), governing learning, memory and strategic decision ( Sapolsky and Pulsinelli, 1985 ; Sapolsky et al., 1985 ; Pavlides et al., 1993 ; Sapolsky, 2000a ; McEwen, 2007 ; Joëls and Baram, 2009 ; Bains et al., 2015 ).
The first step in the stress response is the perception of a stressor. When a situation is perceived as a threat, the brain recruits several neuronal circuits to maintain physiological integrity even in the most adverse conditions ( Ulrich-Lai and Herman, 2009 ). However, detection of different types of stressors requires engagement of different networks. Psychological and physical stressors engage different neuronal networks and cellular activity, leaving distinct footprints within the brain. Stimuli that produce actual disturbances of physiological status, that overwhelm the organism, e.g., hemorrhage or infection are considered as physical stressors. In the other hand, psychological stressors are generally defined as stimuli that threaten the current state and are perceived in an anticipatory condition e.g., aversive environmental stimuli, predator-related cues and failure to satisfy internal drives ( Dayas et al., 2001 ).
Therefore, physical and psychological stressors are processed by different circuitries in the brain, which may overlap at some instances. Regardless of the stressor processing, the stress system will be activated in a coordinated fashion. Here, we present up-to-date information on brain processing for physical and psychological stressors and how they interact (Figure 2 ).
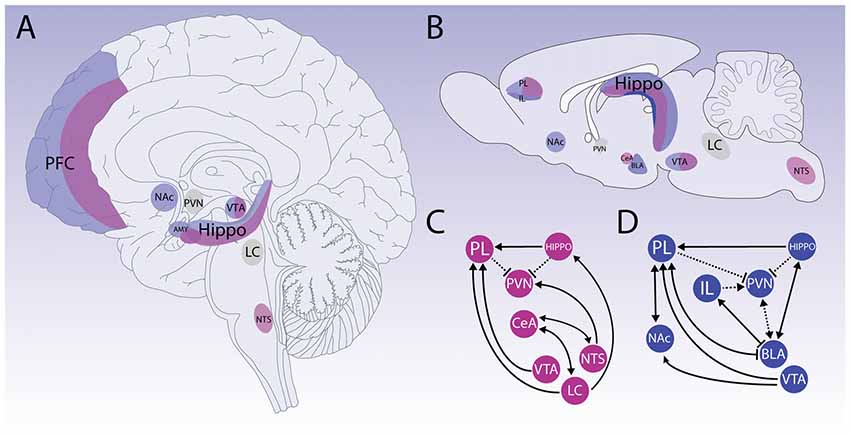
Figure 2 . Neuroanatomy of stress. Schematic representation of primarily neuroanatomical substrates responsible for physical (pink) and psychological (blue) stressors processing. Upper panels show that neural processing for different types of stressors detection and appraisal of the situation engage several structures, which may overlap at some instances on human and rodent brain ( A,B , respectively). Bottom panels represent how physical and psychogenic stressors require engagement of different networks ( C,D , respectively). Physical stressors mainly activate structures related to vital functions control located on brainstem and hypothalamus. Structures such as the nucleus of the solitary tract (NTS) and locus coeruleus (LC) have an important role in the physical stress pathways. However, prosencephalic regions also participate in physical stress processing, such as prelimbic area (PL) in pre-frontal cortex (PFC). Also, it is important to mention that the central nucleus of the amygdala (CeA) participates in autonomic response integration. For instance, psychological stressors are perceived in an anticipatory condition, which may heavily rely on limbic structures and can be modulated by the reward system. The PFC is critical to develop appropriate responses to environment changes, and it is densely innervated by dopaminergic projections from the Ventral Tegmental Area (VTA) and Nucleus Accumbens (NAc). PFC disruption is associated with anhedonia and aberrant reward-seeking behavior. Although PFC involvement is complex and integrates different stress responses in general, PL and Infralimbic (IL) regions coordinate a top-down control. The amygdaloid complex also participates on psychological stress circuitry and with PFC disruption its involvement becomes more prevalent, and the circuitry switches to a bottom-up control. Another important structure that stands out due to its importance on cognitive and memory function, and that is activated in response to both physical and psychological stressors is the Hippocampus (HIPPO). The CA1 region has important connections with the above-mentioned limbic structures and HIPPO is an important structure of the HPA axis negative feedback. The paraventricular nucleus of hypothalamus (PVN) and LC (shown in gray) represent the main relay of the stress response triggering respectively the HPA and the SAM axis. The cross-talk activity between those nuclei allows a cognitive processing of the stress response and enables complex behavioral responses.
Physical Stressors
Physical stressors are mainly processed by brainstem and hypothalamic regions ( Dayas et al., 2001 ; de Kloet et al., 2005a ; Fenoglio et al., 2006 ; Joëls and Baram, 2009 ; Ulrich-Lai and Herman, 2009 ), they usually require immediate systemic reaction, which might be considered reflexive ( Ulrich-Lai and Herman, 2009 ). Thus, the first phase of the stress response (sympathetic adrenomedullar system—SAM), provides a rapid physiological adaptation, resulting in short-lasting responses, such as alertness, vigilance and appraisal of the situation, enabling a strategic decision to face the challenge in the initial phase of a stressful event ( de Kloet et al., 2005a ; Joëls and Baram, 2009 ). Whereas, the secondary phase involves the hormonal mechanism (Hypothalamic Pituitary adrenal axis—HPA) considered sluggish compared to the synaptic mechanisms that activate the SAM, but resulting in an amplified and protracted secretory response (long-lasting responses). Both SAM and HPA axes will be further detailed in the below sections.
Thus, when a real stressor is recognized or perceived through signals (such as pain, inflammation and others) by the brainstem, the neurocircuitry that includes preganglionic autonomic neurons and hypophysiotrophic neurons in the paraventricular nucleus of the hypothalamus (PVN) are activated, generating a rapid autonomic nervous system (ANS) and the HPA axis responses (as illustrated in Figure 1 ; Ulrich-Lai and Herman, 2009 ).
In addition to several nuclei of the brainstem, medullary and spinal cord systems (that participate in the activation of ANS through its sympathetic and parasympathetic arms), physical stressors induce the activation of other brain structures that regulates autonomic stress response including PVN, nucleus of the solitary tract (NTS) and dorsomedial hypothalamus (DMH; Geerling et al., 2010 ). Such structures also have projections to both sympathetic and parasympathetic ANS arms.
The PVN contains distinct populations of neurons that project to autonomic targets in the brainstem and spinal cord (such as the middle cell column, the parabrachial nucleus, the DMX and NTS) and to median eminence sympathetic outflow therefore can be mediated by the PVN, but not exclusively. Complementary to the PVN role, another important hypothalamic nucleus is the DMH, which houses anatomically segregated neuronal populations responsible for regulating autonomic responses and also important for activating or inhibiting HPA axis activity ( Ulrich-Lai and Herman, 2009 ).
The Locus coeruleus (LC; Cunningham and Sawchenko, 1988 ) and rostral ventrolateral medulla (RVLM; Dempsey et al., 2017 ) also directly innervate the intermediolateral cell column, while the dorsal motor nucleus of the vagus nerve (DMX) and the nucleus ambiguus (NA) mediate descending outputs for postganglionic parasympathetic nervous system ( Saper and Stornetta, 2015 ).
It is well known that in the brainstem the NTS has an important role in the stress pathways. It is located in a quite strategic position and constitutes a relay for sensory, visceral and somatic information ( Sapolsky et al., 2000 ; Herman et al., 2003 ; Ulrich-Lai and Herman, 2009 ; Bains et al., 2015 ). Moreover, the NTS is directly involved in the control of the cardiovascular and respiratory functions, containing different neuronal groups that receive distinct ascending pathways, as part of the baroreflex and chemoreceptors regulation ( Machado, 2001 ; Zoccal et al., 2014 ) systems. The NTS also modulates the HPA axis activity mainly through noradrenergic and adrenergic projections to the PVN, but some studies indicate involvement of other catecholaminergic pathways in this modulation ( Herman et al., 2003 ; Gaillet et al., 1991 ; Ritter et al., 2003 ).
Other important structures for triggering the stress response are the circumventricular organs (i.e., the median preoptic nucleus, the subfornical organ (SFO) and the organum vasculosum of the lamina terminalis), that respond to perturbations in fluid and send projections to other integrative brain regions. For instance, the activation of the medial parvocellular PVN by the SFO is essential for the central blood pressure regulation by angiotensin II and regulation of drinking behavior ( Simpson and Routtenberg, 1975 ; Hindmarch and Ferguson, 2016 ).
And finally, it has also been described that limbic forebrain regions may contribute to physical stressors processing as well, influencing the functions in the autonomic responses to stress and the activation of the HPA-axis ( Ulrich-Lai and Herman, 2009 ). These limbic stress circuits involve the amygdala, hippocampus and prefrontal cortex (PFC) that receive associational information from subcortical and cortical areas and their output converge to subcortical relay sites, making downstream processing of limbic information (top-down regulation; Ulrich-Lai and Herman, 2009 ). Those circuitries will be explored in the circuits overlapping section and we have highlighted how stressors might interact with the reward (approaching × aversion) system.
Psychological Stressors
While stressors that are predominantly physically demanding are more likely to evoke autonomic stress responses, psychological uncontrollable and social-evaluative threatening stressors elicit both physical and cognitive stress responses ( Skoluda et al., 2015 ). Together with the prosencephalic nuclei, components of limbic circuits such as the PFC, amygdala, hippocampus (HIPPO), PVN, ventral tegmental area (VTA) and nucleus accumbens (NAc) have a fundamental role in the regulation of stress response ( Ulrich-Lai and Herman, 2009 ; Russo and Nestler, 2013 ).
The PFC is critical to develop appropriate responses to environment changes, enabling behavioral plasticity ( Ridderinkhof et al., 2004 ). However, the involvement of PFC in stress response is complex, since different anatomic subdivisions play different roles. Bilateral lesions of prelimbic (PL) cortex portion increases plasma level of adenocorticotrophic hormone (ACTH), corticosterone and PVN c-Fos expression ( Dioro et al., 1993 ; Figueiredo et al., 2003 ). However, lesions of infralimbic (IL) cortex reduce corticosterone secretion ( Sullivan and Gratton, 1999 ). Moreover, studies with animal behaviors showed that lesions in dorsal sites of PFC result in anxiogenic effects, while lesions in ventral sites of PFC have anxiolytic effects ( Sullivan and Gratton, 2002 ). These data indicate that PL and IL have opposite effects in psychological stressors response, inhibiting PVN activity with anxiolytic effects or stimulating PVN with anxiogenic behaviors, respectively ( Radley et al., 2006 ; Jones et al., 2011 ).
Despite the functional connection between PFC and PVN, anatomical studies indicate that PFC only has indirect projections to PVN ( Herman et al., 2003 ). The PL innervates several GABAergic neurons in the BST, which induces inhibition of PVN, whereas it is suggested that IL pathway projects to non-GABAergic in the BST to stimulate the PVN ( Radley, 2012 ). However, it remains to be elucidated the exact pathways between PVN and PFC ( Bains et al., 2015 ).
The PFC also has major projections to the amygdala, an important structure associated with emotional processing ( Gabbott et al., 2005 ; LeDoux, 2007 ). Initially, the entire amygdala complex appears to promote synthesis and secretion of corticosteroids ( Kawakami et al., 1968 ; Saito et al., 1989 ). However, subsequent studies showed that amygdala subnuclei have distinct roles in stress response ( Roozendaal et al., 2009 ). The amygdala complex can be divided in the basolateral nucleus (BLA), the central nucleus of the amygdala (CeA) and the medial nucleus (MeA). Among these, the BLA has a major role in processing psychological stressors ( Janak and Tye, 2015 ), being mainly activated by anticipatory stressors ( Cullinan et al., 1995 ). However, BLA does not affect corticosterone release itself ( Seggie, 1987 ). The role of BLA in the processing of psychological stressors seems to be more critical to the consolidation of aversive memories ( Roozendaal et al., 2009 ).
Intra-BLA infusions of GABAergic receptor agonists impair aversive memory consolidation ( Brioni et al., 1989 ). Besides, after emotional arousal, BLA neurons show lasting increases in the spontaneous firing rates, which could facilitate the consolidation of emotional memories through synaptic plasticity changes ( Pelletier et al., 2005 ). Alterations in emotional and associative learning (i.e., aggression and learned helplessness) increased dendritic arborization in BLA neurons ( Vyas et al., 2004 ; Bennur et al., 2007 ; Wood et al., 2008 ). In specific, some studies indicate that BLA-PFC pathway plays a major role in memory consolidation and stress response ( Laviolette and Grace, 2006 ; Felix-Ortiz et al., 2016 ; Burgos-Robles et al., 2017 ). The BLA have extensive bi-directional monosynaptic glutamatergic projections with PFC (PL and IL; Gabbott et al., 2005 ; Dilgen et al., 2013 ; McGarry and Carter, 2016 ), which is crucial for emotional learning, fear-related responses, anxiety-like behaviors and social interactions ( Laviolette and Grace, 2006 ; Felix-Ortiz et al., 2016 ; Burgos-Robles et al., 2017 ). Finally, the activity of stress response in the BLA is probably not only dependent on interactions with PFC, but also mediated by projections between PVN and amygdala ( Prewitt and Herman, 1998 ).
The BLA has abundant output to the CeA and MeA, which have multisynaptic connections to the PVN ( Herman et al., 2003 ). The CeA and MeA innervate brainstem structures that directly projects to PVN, such as the bed nucleus of the stria terminalis (BNST; Prewitt and Herman, 1998 ). However, recent evidence with optogenetics approaches indicates that PVN projections to the CeA are activated during retrieval of long-term fear memories. In contrast, retrieval of short-term memories is mostly dependent on PFC inputs to the BLA, indicating a time-dependent shift in fear memories circuits ( Do-Monte et al., 2015 ). In turn, CeA has direct connections to periaqueductal gray (PAG), a fundamental area that organizes the threat response ( LeDoux, 2012 ). Specifically, dorsal PAG is related to unconditioned threats exposure and ventral PAG is activated in conditioned threats, each one with distinct behavioral outputs. While lesions in dorsal PAG increased freezing behavior, damage to the ventral PAG decreased freezing ( De Oca et al., 1998 ; LeDoux, 2012 ).
Another important limbic structure for stress processing is the hippocampal formation ( McEwen et al., 1968 ; Smotherman et al., 1981 ), which consists of the dentate gyrus (DG) and Ammon’s horn/ Cornus Ammonics (CA) subfields that compose the well-known tri-synaptic circuit, first from DG granule cells to CA3 pyramidal cells and then from CA3 to CA1 pyramidal cells ( Amaral and Witter, 1989 ; Andersen et al., 2000 ; Naber et al., 2001 ). While the DG receives most of extra-hippocampal afferents from the entorhinal cortex via the perforant path ( Witter, 2007 ), the CA1 and subiculum pyramidal neurons are the main HIPPO output to several areas, such as excitatory projections to PFC (PL and IL) and indirect inhibitory projections to PVN ( Barbas and Blatt, 1995 ; Herman and Mueller, 2006 ).
The hippocampal inhibitory control over the HPA axis acts in a negative feedback manner inhibiting it ( Jacobson and Sapolsky, 1991 ; Herman et al., 1996 ). The mainly indirect hypothalamic input of HIPPO originates in the CA1 and subiculum that densely innervate BNST and hypothalamic structures, except PVN ( Canteras and Swanson, 1992 ; Cullinan et al., 1993 ).
Projections from HIPPO to both the PFC and BLA present some of the most important role in memory but especially for the regulation of psychological stress response ( Godsil et al., 2013 ). HIPPO has bidirectional connections with BLA, but at the same time allows PFC to modulate the stress system with a top-down control ( Mcdonald, 1998 ; Janak and Tye, 2015 ; Radley et al., 2015 ). However, the CA1 and subiculum monosynaptic glutamatergic efferents to PL and IL can become functionally disrupted after intense psychological stress ( Zheng and Zhang, 2015 ). In a strong emotional experience, HIPPO and BLA rapidly activate memory-related mechanisms of synaptic plasticity while PFC functioning is suppressed, promoting long-lasting flashbulb memories ( Diamond et al., 2007 ).
Therefore, in the presence of psychological stressors, if the activity of the amygdala and HIPPO becomes more prevalent, the stress system can switch to a bottom-up control ( Arnsten, 2009 ). On top of that, prolonged psychological stress decreases glutamatergic projection to interneurons in BLA, leading to loss of BLA inhibition by the PFC and finally, to the hyperexcitability of BLA, which is responsible for behavioral abnormalities related to stress ( Wei et al., 2017 ).
At this point, among several hypothalamic nuclei that are directly involved in regulating HPA axis and autonomic responses to stressors, the PVN stands out as the principal integrator of stress signals ( Herman et al., 2003 ; Ulrich-Lai and Herman, 2009 ). In general, the medial parvocellular neurons of the PVN receive projections of first or second order of somatic afferent nociceptive, visceral, humoral or sensory pathways, beyond those of the limbic and reward system such as PFC, amygdala, HIPPO, VTA and NAc ( Russo and Nestler, 2013 ; Bains et al., 2015 ). As already mentioned before, most limbic–PVN connections are indirect and are made through GABAergic cell groups in the BNST and peri-PVN regions of the hypothalamus ( Crestani et al., 2013 ). Studies involving activity mapping (mostly c-Fos expression) have demonstrated an important role for BNST in the regulation of the HPA axis ( Zhu et al., 2001 ), while the posterior BNST nuclei are involved in inhibition of the HPA axis, the anteroventral nuclei are involved in its excitation ( Choi et al., 2007 ). In the reward system, pharmacological inhibition of NAc increased c-Fos expression in PVN ( Noh et al., 2012 ). Lastly, the parvocellular neurons of the PVN are strategically connected with several brainstem and forebrain nuclei such as NTS, DMH, LC, VLM, DMX and NA, important to the processing and integration of both modalities of stressors, physical and psychological, which results in a rapid activation of the HPA axis ( Ulrich-Lai and Herman, 2009 ; Senst and Bains, 2014 ).
Overlapping Processing
Many of the above circuitry and brain areas responsible for physical and psychological stressors may sound exclusively related to brainstem and forebrain, respectively. However, some structures are also engaged with different modalities of stressor (Figure 2 ).
The best-characterized reward circuit in the brain is made up of dopaminergic neurons in the VTA that project to the NAc, a subdivision of the ventral striatum ( Bath et al., 2017 ). The primary brain reward centers are inter-connected in complex ways in the brain reward circuitry, but mostly VTA and NAc regulates limbic areas mentioned above ( Heshmati and Russo, 2015 ).
The dopaminergic tonus of VTA is fundamental to the recognition of rewards or punishment in the environment ( Russo and Nestler, 2013 ). Exciting all VTA neurons enhances/mimics social defeat stress-induced effects on social avoidance and anhedonia while silencing all VTA neurons (regardless of the projection region or the cell type) promotes the opposite effect ( Krishnan et al., 2007 ). On the other hand, the optogenetic stimulation of VTA dopaminergic neurons drives to a conditioned place preference ( Tsai et al., 2009 ), whereas inhibition of VTA promotes conditioned placed aversion ( Tan et al., 2012 ).
However, VTA is composed by different neuronal subpopulations, which participate in distinct circuits that encode different motivational signature ( Lammel et al., 2012 , 2014 ). Dopaminergic neurons of VTA project to NAc, HIPPO, BLA and PFC, however, the NAc also receives glutamatergic innervations from ventral HIPPO, BLA and PFC ( Russo and Nestler, 2013 ; Lammel et al., 2014 ) and all these nuclei are under the regulatory influence of dopamine. Indeed, dopaminergic neurons that innervate the mPFC show reduced firing after social defeat chronic stress ( Chaudhury et al., 2012 ). Dopaminergic neurons in VTA exhibit two patterns of spontaneous firing activity: a slow-frequency, single-spike firing and a burst firing, effectively regulating the activity of neurons in dopaminergic target areas and encoding reward-related signals ( Grace et al., 2007 ). This mechanism of encoding and engaging specific networks makes sense since the behavioral response to a rewarding (approach) vs. an aversive experience (aversion) is different and therefore involves different, perhaps overlapping, neural circuits ( Lammel et al., 2011 ).
Accordingly, associating this fined-tuned VTA regulation to the stress processing, it has been proposed that a specific ventral VTA pathway is related to painful physical stress. Several findings showed that dopaminergic cells that specifically project to the mPFC regulate the process of noxious stimuli ( Abercrombie et al., 1989 ; Mantz et al., 1989 ; Bassareo et al., 2002 ; Brischoux et al., 2009 ; Matsumoto and Hikosaka, 2009 ; Lammel et al., 2011 ). But it is important to mention that although dopamine has a central role in processing physical stressors, in this scenario, the NAc detains the central role in regulating the reward system ( Grace et al., 2007 ) which is deeply related to the processing of psychological stressors and promotion of behavioral flexibility ( Heshmati and Russo, 2015 ).
Essentially, HIPPO projections to NAc promote susceptibility to psychological stress, BLA-NAc pathway increases reward seeking behavior and PFC-NAc promotes resilience ( Stuber et al., 2011 ; Vialou et al., 2014 ; Bagot et al., 2015 ; Heshmati and Russo, 2015 ). Increased firing of VTA dopaminergic neurons that innervate the NAc occurs only in susceptible mice after chronic social defeat stress, while VTA-PFC pathway reduced firing after the same protocol ( Chaudhury et al., 2012 ). Therefore, we can see that while mesocortical pathways encode physical processing, the NAc may represent an important hub in processing psychological stress.
Not only the PFC activity corresponds to an integrative hot point of limbic and reward circuitry, but also its contribution to the inhibition of the HPA axis by PFC top-down control is crucial. If the inhibitory HPA axis feedback does not function properly, as in the case of chronic stress and neuropsychiatric disorders, the loss of negative feedback in the PVN regulated by the PFC and HIPPO, associated to the hyperexcitability of BLA and silencing of NAc creates a positive feedback in the PVN that can both overload the stress system ( Duvarci and Paré, 2007 ; Willner et al., 2013 ) and deplete the reward system ( Heshmati and Russo, 2015 ).
Also, regarding the PFC, interesting data have revealed that stimulation of PL enhances hypoxia-mediated corticosterone responses and PVN by using c-Fos activation mapping ( Jones et al., 2011 ). Some brainstem nuclei that regulate autonomic response, such as DMX and NAc, receive and send information from and to other regions of the CNS, respectively, including IL and PL portions of PFC, the CeA and BNST ( Ulrich-Lai and Herman, 2009 ).
The CeA, previously mentioned as a central hub in fear response is also considered as a key node for stress integration ( Ulrich-Lai and Herman, 2009 ). Moreover, NTS is also densely innervated by afferent projections from the CeA ( Smith and Vale, 2006 ). The NTS can be activated during conditioning paradigms, suggesting that this pathway also plays a role in the integration of anticipatory stress ( Pezzone et al., 1993 ).
It is important to highlight that different modalities of stressors that activate the PVN also activate the LC-Norepinephrine (LC-NE) system, such as restraint, shock (notably unpredictable), audiogenic stress, autonomic and immunological challenges and also social stress ( Wood and Valentino, 2016 ). The LC is a compact pontine nucleus adjacent to the fourth ventricle and houses the majority of the NE-expressing neurons in the brain and innervates the entire neuraxis ( Swanson and Hartman, 1975 ).
Activation of the LC-NE occurs in parallel and coordinated with PVN activation. This cross-talk between those nuclei allows a cognitive processing of the stress response engaging limbic and prosencephalic regions, such as the HIPPO and cortex, which govern neuronal excitability, cognition, memory and complex behaviors ( Joëls and de Kloet, 1989 ; Wood and Valentino, 2016 ). Recent techniques, such as chemogenetics, optogenetics, combined with traditional retrograde tracing, which enable selective manipulation of LC-NE system in rodents, determined the pivotal role of the LC-NE, for example, for stress-induced anxiety-like behavior ( McCall et al., 2015 ). Interestingly, Corticotrophin Release Hormone (CRH), which initially was discovered and defined as the hormone that initiates the cascade that ultimately leads to glucocorticoids release, seems to stand out as the molecule that coordinates the cross-talk between the two systems ( Valentino and Van Bockstaele, 2008 ).
The LC-NE network is a major target of CRH ( Chappell et al., 1986 ; Wood and Valentino, 2016 ). During stress, CRH is released into the LC, increasing neuronal firing rate and consequently NE release in forebrain targets ( Valentino et al., 1991 ; Jedema and Grace, 2004 ). Different structures that coordinate autonomic and limbic processing such as CeA ( Van Bockstaele et al., 1998 ), BNST ( Van Bockstaele et al., 1999 ), PVN ( Reyes et al., 2005 ), paragigantocellularis nucleus and Barrington’s nucleus ( Valentino et al., 1992 , 1996 ) send CRH modulatory projections to LC. Barrington’s nucleus is one of the few nuclei that actually sends projections directly into the core of the LC and also has important projections to preganglionic column of the lumbosacral spinal cord ( Valentino et al., 1996 ).
Interestingly, CRH release within the LC is regulated by basal levels of corticosteroids as well ( Valentino and Van Bockstaele, 2008 ).This communication between HPA and LC-NE axis determines the structural basis for emotional arousal, facilitates cognition and promote flexible behavioral responses to stress ( Cole and Koob, 1988 ; Valentino and Van Bockstaele, 2008 ), since CRH release in the LC during stress facilitates shifting of attention between diverse stimuli ( Snyder et al., 2012 ). Therefore, this crosstalk enables organisms to tailor different strategies to coping with constant changing environmental challenges.
SAM Axis and Catecholamines
The stress response to physical and/or psychological stressors, organized by the previously described brain circuitry, involves a rapid physiological adaptation mediated mainly by catecholamines ( de Kloet et al., 2005a ; Joëls and Baram, 2009 ; Tank and Lee Wong, 2015 ). In fact, epinephrine (E) and NE are secreted from adrenal medulla ( Cannon, 1914 ; de Kloet et al., 2005a ; Joëls and Baram, 2009 ; Kvetnansky et al., 2009 ; Tank and Lee Wong, 2015 ) and NE from sympathetic nerves ( Euler, 1946 ; Kvetnansky et al., 2009 ; Tank and Lee Wong, 2015 ).
The circuitry responsible for these autonomic modulations includes direct projections from PVN, LC and RVLM ( Iversen et al., 2000 ; Ulrich-Lai and Herman, 2009 ) to pre-ganglionic sympathetic neurons present in dorsal intermediolateral cellular column (IML) of the spinal cord (thoracolumbar region), being these nuclei modulated by the NTS ( Ulrich-Lai and Herman, 2009 ). Each pre-ganglionic fiber connects with many post-ganglionic neurons located in one or several pre-spinal ganglia or sympathetic paravertebral nuclei ( Boron and Boulpaep, 2009 ). Although, there are many efferent pathways of the ANS, only two neurons are necessary to transmit impulses between the CNS to the effector tissue ( Mccorry, 2007 ).
Other pre-ganglionic neurons, which end at the spinal cord, do not make synapses with a post-ganglionic neuron. For instance, they make synapses directly with chromaffin cells in the adrenal medulla ( Mccorry, 2007 ; Boron and Boulpaep, 2009 ). This glandular tissue synthesizes and secretes E and NE, being E 80% of the catecholamine output by the adrenal medulla in humans ( Goldstein et al., 2003 ; Dünser and Hasibeder, 2009 ; Tank and Lee Wong, 2015 ). Thus, these two components increase the capacity of the sympathetic division in influencing body visceral responses ( Boron and Boulpaep, 2009 ). The sympathetic system activation leads to activation of signaling pathways that evoke changes in blood vessels, glands, visceral organs and smooth muscles ( Tank and Lee Wong, 2015 ).
The parasympathetic component of the ANS regulates the action and duration of the autonomic responses, generating the so-called “vagal tone” of the cardiac and respiratory systems ( Iversen et al., 2000 ; McEwen, 2007 ; Davidson and McEwen, 2012 ). Pre-ganglionic parasympathetic neurons originate from craniosacral vertebral segments (brainstem and sacral spinal cord) synapse with post-ganglionic neurons in the terminal nodes located more peripherally, and usually on the wall of the target organs. Post-parasympathetic ganglionic neurons stimulate the muscarinic and nicotinic receptors present on the membrane of the target cells by releasing acetylcholine ( Boron and Boulpaep, 2009 ).
The effect caused by any of these substances, acetylcholine, norepinephrine and epinephrine, depends on the biochemical properties of the cells and on the receptor distribution in a determined tissue ( Mccorry, 2007 ). In general, SAM activation is considered to mediate short-term effects, with rapid responses, while the HPA axis activation leads to short and long-term effects ( Joëls and Baram, 2009 ; Tank and Lee Wong, 2015 ). As previously mentioned, interactions of these major stress systems (SAM and HPA) occur at several levels, functioning cooperatively and/or sequentially, acting in opposite ways in most visceral organ targets.
Although, these sympathetic and parasympathetic systems act independently from each other ( Antunes-Rodrigues et al., 2005 ; Ulrich-Lai and Herman, 2009 ; Tank and Lee Wong, 2015 ) dominance of the sympathetic system occur under conditions such as exercise and “fight-or-flight” reactions, the parasympathetic system predominates during resting conditions ( Mccorry, 2007 ).
E and NE interact with adrenergic receptors present in cell membranes of smooth muscles and in numerous organs, as well as in neurons widespread in the CNS ( Mccorry, 2007 ; Tank and Lee Wong, 2015 ). These receptors are the G-protein coupled receptors (GPCRs), which are homologous to muscarinic receptors, i.e., are proteins embedded in the cell membrane with seven transmembrane domains ( Mccorry, 2007 ; Dünser and Hasibeder, 2009 ). There are two major types of adrenergic receptors; α- adrenergic and β-adrenergic receptors, with their subtypes ( Langer, 1980 ; Guimarães and Moura, 2001 ; Dünser and Hasibeder, 2009 ).
The rise in circulating E and NE causes general physiological changes that prepare the body for “fight-or-flight” reaction ( Cannon, 1915 , 1929 ; Bowman, 1930 ). Their effects include: maintaining alertness, metabolic actions (increased glucose via glycogenolysis and gluconeogenesis, lipolysis, increased oxygen consumption and thermogenesis) and cardiovascular actions ( Aires, 2012 ).
The central noradrenergic system, specifically the LC is involved in multiple neurochemical circuits, having connections with neuroanatomical structures involved in the stress response, such as the HIPPO, amygdala and temporal neocortex. Studies indicate an important role of the LC in response to acute stress ( Myers et al., 2017 ), acting as an “alarm system,” in attention, excitation and defensive responses ( Cassens et al., 1981 ; Valentino and Van Bockstaele, 2008 ). However, chronic activation of the LC may have a potential role in the development of pathological behaviors related to stress ( Southwick et al., 1999 ; Ziegler et al., 1999 ; Valentino et al., 2012 ; George et al., 2013 ; Reyes et al., 2015 ), which will be discussed in one of the following sections.
Thus, the release of NE has central actions, coordinates and modulates autonomic, endocrine and neuroendocrine responses, through extensive brain and spinal cord connections, while direct projections from LC to medial parvocellular division of the PVN ( Cunningham and Sawchenko, 1988 ) allows the modulation of the HPA axis ( Armario et al., 2012 ). On the other hand, the activity of LC itself may be influenced by CRH through afferent projections from amygdala and brainstem nuclei ( McCall et al., 2015 ; Sun et al., 2015 ), facilitating the complex behavioral and cognitive reactions to stress ( Valentino and Van Bockstaele, 2008 ).
HPA Axis and Glucocorticoids
When an organism faces a threatening stimulus, whether it is psychological or physical, as explained in the previous sections, many brain areas are activated in a coordinated fashion to recruit a complex structure known as hypothalamus, which is composed by many sub nuclei. As already discussed, among those nuclei is the PVN, which is responsible for eliciting the activation of the HPA axis, one of the main components of the stress response. The PVN synthesizes three different neurochemical compounds that behave either as neurotransmitters or hormones, depending on where they are acting. These compounds are oxytocin, vasopressin and CRH ( Vale et al., 1981 ; Sawchenko et al., 1992 , 1996 ).
The pituitary is situated caudally and above the optical chiasm, and consists of an anterior portion (adenohypophysis), that synthesizes and secretes hormones and of a posterior portion (neuro-hypophysis), that stores oxytocin and vasopressin synthesized by the parvocellular neurons in the PVN ( McCann and Brobeck, 1954 ; Joëls and Baram, 2009 ).
When CRH reaches the anterior pituitary, it stimulates the corticotrophs to synthesize and release the ACTH ( Vale et al., 1981 ). ACTH is secreted through the hypophyseal portal system, and acts on the cortex of the adrenal gland, more specifically on the middle layer named fascicullata ( Vale et al., 1978 ; Herman et al., 2003 ), which is responsible for glucocorticoid synthesis and secretion ( Vale et al., 1978 ). The main glucocorticoid in humans is cortisol, and its equivalent in rodents is corticosterone ( de Kloet, 2013 ).
Although the HPA axis has an ultradian rhythm ( Young et al., 2004 ; de Kloet and Sarabdjitsingh, 2008 ; Lightman and Conway-Campbell, 2010 ), levels of corticosteroid hormones follow a circadian rhythm, as well as a glucocorticoid peak secretion in humans, occurring during the early morning and in rodents in the beginning of the evening ( Reppert and Weaver, 2002 ). There are many evidences indicating that the suprachiasmatic nucleus (SCN) of the hypothalamus is the generator of the circadian rhythm ( Welsh et al., 1995 ; Jagota et al., 2000 ; Reppert and Weaver, 2002 ; Engeland and Arnhold, 2005 ). Glucocorticoids act on the anterior pituitary, PVN and other brain structures, such as the HIPPO ( McEwen et al., 1968 ), controlling the activity of the HPA axis. This phenomenon is called short and long negative feedback loops, respectively ( de Kloet et al., 1991 ; Joëls and Baram, 2009 ; Bains et al., 2015 ).
Glucocorticoids are steroids and easily trespass cell membranes and since they are released in the bloodstream they can virtually reach any cell in the body. Although peripherally the glucocorticoids act massively in several target-organs, here we focus on their effects on the brain. Cortisol, or corticosterone in rodents, exerts their effects in the brain by binding to two types of receptors, the glucocorticoid receptor (GR) and the mineralocorticoid receptor (MR; Reul and de Kloet, 1985 ; de Kloet et al., 2005a , b ). These receptors mediate the effects of glucocorticoids in the brain through genomic and non-genomic mechanisms ( Verkuyl et al., 2005 ; de Kloet and Sarabdjitsingh, 2008 ; Groeneweg et al., 2012 ; Joëls et al., 2012 ) and therefore GR and MR characteristics, such as distribution, affinity and mechanism of action are determinant to regulate homeostasis under basal condition or to promote adaptation through the stress response.
The MR shows 10 times higher affinity for corticosterone than GR ( Reul and de Kloet, 1985 ). Such difference is translated to receptor occupancy throughout the day, during the through phase of the circadian cycle, when glucocorticoid levels are low, MRs are occupied whereas the GRs are mostly free. During the peak phase of the circadian cycle, or after high glucocorticoid release due to the stress response, MRs are completely occupied while GRs are partially occupied ( Kitchener et al., 2004 ; Young et al., 2004 ).
Although glucocorticoids are able to reach all neurons in the brain, they exert effects on those neurons expressing GRs and MRs. GRs are abundant and widely spread throughout the brain. On the other hand, MRs are expressed in restricted areas of the brain ( Reul and de Kloet, 1986 ). It is also important to mention that there are some key structures that express both receptors, such as the PVN, HIPPO, amygdala, lateral septum, LC and NTS, among others. Moreover, GR and MR also co-localize with adrenoreceptors allowing the interplay between the SAM and HPA axes ( Härfstrand et al., 1986 ; Joëls and de Kloet, 1989 ; Pu et al., 2007 ; Krugers et al., 2012 ; Zhou et al., 2012 ).
Upon binding to GR or MR, located in the cytosol, receptor-ligand complex forms monomers or dimers (homo or heterodimers) that translocate to the nucleus and bind to promoter regions of about 1%–2% of the genome (known as Glucocorticoid Responsive Elements), or interact with transcriptional factors, usually hampering their efficacy ( Morsink et al., 2006 ; Datson et al., 2008 ; Grbesa and Hakim, 2017 ; Weikum et al., 2017 ). These delayed and long-lasting effects of GR and MR receptor activation ( Joëls et al., 2012 ) mediate transcription of proteins involved in immune, cognitive, metabolic and many other physiological processes, in order to promote changes in physiology and behavior. Moreover, it has also been shown that not only genomic processes are triggered by GR and MR, actually there are studies clearly showing rapid effects of membrane-located MR and GR activation ( Borski, 2000 ; Johnson et al., 2005 ; Karst et al., 2005 ; Olijslagers et al., 2008 ; Wang and Wang, 2009 ; Evanson et al., 2010 ; Karst et al., 2010 ; Roozendaal et al., 2010 ; Groeneweg et al., 2012 ; Nahar et al., 2016 ).
Important to mention, it has been demonstrated that GR levels, and consequently HPA axis function, can be modulated by the environment, as well as life experiences (acute and chronic stressors), through stable changes in the DNA chromatin, which does not alter DNA sequence, a mechanism known as epigenetics ( Hunter et al., 2009 ; Griffiths and Hunter, 2014 ; Buschdorf and Meaney, 2015 ). Alterations such as methylation and acetylation of histones occur genomewide enhancing or hampering chromatin activity, however DNA methylation can also occur in a gene-specific fashion altering its expression ( Tsankova et al., 2006 ; Nestler, 2014 ). In fact, Weaver et al. (2004) showed that environment factors, such as maternal care, play a fundamental role in the programming of the HPA axis function, morespecifically on the nuclear receptor subfamily 3 group C member 1 ( NR3C1) gene (GR), which persists through adulthood. These authors demonstrated that pups, which received low care (licking and grooming the pups) from their dams, had higher hippocampal NC3R1 methylation associated with lower GR expression, when compared to those that received high levels of licking and grooming.
Epigenetic modulation of GR expression has also been described in humans as highlighted by Palma-Gudiel et al. (2015) and Smart et al. (2015) and although some studies point out the fact that stress (early-life adversities) lead to NR3C1 hypermethylation, it is still controversial the association of these epigenetic changes with psychopathologies such as depression in humans ( McGowan et al., 2009 ; Alt et al., 2010 ; Na et al., 2014 ; Palma-Gudiel et al., 2015 ; Smart et al., 2015 ). In the other hand, pre-clinical data have associated stress-induced epigenetic alterations with vulnerability or resilience to psychiatry-like conditions ( Covington et al., 2009 , 2011 ; Wilkinson et al., 2009 ; Nestler, 2014 ).
Stress and the Immune System
As mentioned previously, it is through the GR receptor that the HPA axis modulates the immune system, which involves protein-protein and/or DNA interactions (for further review see Liu et al., 2014 ). Although, the discovery of the interplay between the inflammatory and endocrine systems is dated as a long-time story, it still remains as a hot topic in the field of stress research. At the same period Hans Selye discovered his canonical findings on stress, the prestigious Mayo Clinique developed the substance E ( Neeck, 2002 ; Hillier, 2007 ; Lupien, 2015 ). This substance crystalized by Dr. Kendall ( Mason et al., 1936 ) was being used for treating Addison’s disease ( Kendall, 1953 ), later on recognized as cortisol, which gave birth to a myriad of “wonder” drugs for a variety of inflammatory disorders such as Rheumatoid Arthritis ( Neeck, 2002 ; Hillier, 2007 ; Lupien, 2015 ).
Most recently, research on the relationship between stress hormones and immune system has unraveled many intricate pathways that also can be explored for their clinical implications. It has been postulated that besides preparing the body to deal with the environment demands, stress activates the immune system, which engages active defense against physical injury and pathogens. Ultimately, cytokines are produced to promote multiple kinds of inflammatory responses ( Takahashi et al., 2018 ). But reports stated that stress-enhanced inflammatory activity is present in the absence of infectious pathogens, especially in depressed patients ( Audet et al., 2014 ).
To address whether stress or its hormones are pro or anti-inflammatory, evidence using rodent models demonstrated that stress itself can be both pro- and anti-inflammatory. Some authors have proposed that the timing of immune challenges and measurements determines the direction of glucocorticoid actions. Those authors proposed that glucocorticoids initially present anti-inflammatory action, but later on sensitizes the immune response on the recovery phase (after stressor; Frank et al., 2013 ).
Stress can directly influence immune signaling in two main ways, by reducing the inhibitory effects of glucocorticoid actions, or by directly stimulating the immune system via HPA axis and SAM ( Liu et al., 2014 ; Wohleb et al., 2015 ). But not only the neural components of stress engage the immune system, the immune system also affects the CNS, modulating the HPA axis ( Berkenbosch et al., 1987 ; Linthorst et al., 1994 ; Angeli et al., 1999 ). Therefore, acutely, stressful experiences enhanced levels of circulatory pro-inflammatory cytokines ( Steptoe et al., 2007 ). Some of these inflammatory cytokines are either locally produced by activated microglia ( Wohleb et al., 2015 ) or get access to the brain through circumventricular sites ( Vitkovic et al., 2000 ) or are transported ( Banks, 2006 ).
Interestingly, the sites that have increased pro-inflammatory immune reactivity appear to be related to acute stressor modalities. Social stressors increased expression of pro-inflammatory interleukins and activated microglia in sites such as PFC, amygdala and HIPPO ( Audet et al., 2010 , 2011 ; Tynan et al., 2010 ; Wohleb et al., 2011 ; Hinwood et al., 2012 , 2013 ) whereas physical stressors promoted an increase in the hypothalamus ( O’Connor et al., 2003 ; Deak et al., 2005 ; Hueston and Deak, 2014 ).
Time Domain of Stress
The complexity of the stress response is not restricted to neuroanatomy and mediator molecules, but also diverge according to timing and duration of stressor exposure, as well as its short- and/or long-term consequences ( de Kloet, 2013 ). Stress mediators operate in a feedback loop after HPA axis activation, and regulate, in a positive or negative way, different brain structures to restore homeostasis ( de Kloet et al., 2005b ). When the timing of stress response is inappropriate, aberrant HPA axis activity could lead to pathological states ( Heim et al., 2000 , 2008 ; de Kloet et al., 2005a ; Juruena, 2013 ; Nemeroff, 2016 ). We can divide basal regulation of glucocorticoids release in ultradian and circadian cycles, and also categorize the effects of stress response in rapid or delayed regarding their initiation, and in short or long-term, regarding their duration, in both scenarios they range from milliseconds to days ( Joëls et al., 2012 ). Moreover, stress also differs when it occurs in early-life or adulthood of an individual, which can increase or decrease the possibility of developing brain disorders ( Lupien et al., 2009 ; Juruena, 2013 ; Timmermans et al., 2013 ).
In ultradian and circadian cycles, peaks of corticosterone release contribute to regulation of basal metabolic demand and the responsiveness of stress ( Lightman and Conway-Campbell, 2010 ). The origin of pulsatile corticosterone release in an ultradian and circadian fashion is not fully elucidated, but it has been a general assumption that the hypothalamus modulates this phenomenon, specifically, the hypothalamic SCN has efferent projections to neuroendocrine cells in the PVN that trigger the HPA axis activation ( Engeland and Arnhold, 2005 ).
Acute stress mediators start acting within seconds after the stressor detection and provide quick responses to an appropriated strategy, involving modulation of limbic-cortical circuits ( Bains et al., 2015 ). The PVN in acute stress is fundamental, both to drive “fight-or-flight” responses through stress mediators release, or to inhibit acute response ( Dallman, 2005 ). After corticosterone is released, frequency of miniature excitatory postsynaptic currents (mEPSCs) in PVN are suppressed, which decreases glutamatergic excitability and increases GABA inhibition ( Di et al., 2005 ). These effects of corticosterone in the PVN occur mainly via non-genomic GR activity and endocannabinoid signaling ( Di et al., 2003 ; Verkuyl et al., 2005 ). However, in other structures, excitatory activity is increased after acute stress ( Joëls et al., 2012 ), for instance corticosterone release enhances mEPSC frequency via MR in CA1 pyramidal cells ( Karst et al., 2005 ; Olijslagers et al., 2008 ). Similarly, mEPSC frequency in BLA is also increased via MR after acute corticosterone release ( Karst et al., 2010 ). Interestingly, firing frequency of BLA neurons remains at high levels even after corticosterone washout, mainly modulated by GR and cannabinoid receptor 1 ( Karst et al., 2010 ). In cortical structures, such as the PFC, acute stress situation increases GR-dependent glutamate release ( Musazzi et al., 2010 ).
Hours after stressor exposure, delayed effects start to occur in a different way on limbic-cortical structures ( Joëls et al., 2012 ). Neurons of CA1 have enhanced amplitude but not frequency of mEPSCs via GR ( Karst et al., 2005 ; Martin et al., 2009 ). The GR activity also modulates synaptic plasticity in CA1, promoting Long-term Depression (LTD) and impairs Long-term Potentiation (LTP; Pavlides et al., 1993 ; Xiong et al., 2004 ; Kim et al., 2006 ). In opposite, corticosterone presents delayed effects via MR by increasing the induction of LTP in CA1 ( Pavlides et al., 1996 ). In BLA, delayed effects of corticosterone increase excitability of neurons, maintaining excitability after acute stress ( Duvarci and Paré, 2007 ). Similarly, corticosterone enhances glutamatergic transmission and reduces inhibitory post-synaptic currents (mIPSCs) in the PFC ( Hill et al., 2011 ). These delayed effects of stress in limbic-cortical structures restore homeostasis, as well as retain important information to better cope with similar situations in the future ( Joëls et al., 2013 ).
When there is an over-exposure to stressors, lasting from hours to days, it is possible to observe structural changes in limbic-cortical areas and even in the reward system ( Joëls et al., 2013 ; Russo and Nestler, 2013 ). Dendritic complexity is progressively reduced in HIPPO and PFC, after chronic exposure to stressors ( McEwen and Magarinos, 1997 ; Holmes and Wellman, 2009 ). By contrast, neurons in the BLA and NAc increase dendritic density, increase excitatory tone and decrease inhibitory tone in this context ( Vyas et al., 2002 ; Christoffel et al., 2011 ; Muhammad et al., 2012 ). At the cellular level, chronic stress impairs induction of LTP in CA1 of the HIPPO and reduces both AMPA and NMDA-mediated synaptic transmission ( Joëls et al., 2012 ; Yuen et al., 2012 ). The behavioral consequences of these structural changes were associated with anxious behavior, probably by hypertrophy of the amygdala ( Mitra and Sapolsky, 2008 ) and deficits in learning, which could be explained by impaired hippocampal and PFC structures ( Joëls et al., 2012 ; de Kloet, 2013 ) and reduced BDNF in VTA ( Krishnan et al., 2007 ).
It is interesting to note that when chronic stress is experienced early in life, its effects on the brain last longer than when it occurs during adulthood ( Lupien et al., 2009 ). When rodent pups are exposed to prolonged maternal separation, the density of CRH binding sites increases in HIPPO, amygdala and PFC ( Anisman et al., 1998 ). Activity of CRH mediates stress-related synaptic plasticity loss in the HIPPO, anxiogenic behavior dependent of amygdala and cognitive impairment associated with PFC ( Schulkin et al., 1998 ; Sánchez et al., 2001 ; Fenoglio et al., 2006 ).
Clinical Implications
At this point, in our evolutionary history, stress could be implied in a maladaptive performance in a large proportion of the population, considering the large number of comorbidities that occur from dysfunction of the stress system ( de Kloet et al., 2005b ). The HPA axis dysregulation and prolonged exposure to glucocorticoids reduce the ability of neurons to resist insults, increasing the risk for injury by other toxic events ( Lupien et al., 2009 ). Moreover, new researches have called attention to adversities in early life, which are greatly associated with higher vulnerability to disorders later in life, causing a long-term impact in the circuitry responsible for cognitive and emotional function ( Gold et al., 1988 ; Heim and Nemeroff, 2002 ; Lippmann et al., 2007 ; Chrousos, 2009 ; Lupien et al., 2009 ; Juruena, 2013 ; Lucassen et al., 2013 ; Krugers et al., 2017 ).
In this sense, both basic and clinical researches have advanced in recent years but much remains to be understood about the subject. In general, animal models have provided a comprehensive view of the stress effects on the brain, abundantly on the limbic structures ( Hariri and Holmes, 2015 ). The amygdala is a highly conserved brain structure that is fundamental to detect potential danger ( Janak and Tye, 2015 ), while HIPPO provides support to encoding environmental information associated with the stressor ( Herman et al., 2005 ) and the PFC provides associations between cues and stressor ( Milad and Quirk, 2012 ). The identification of these highly evolutionary conserved networks that are affected by stress, allowed important discoveries in clinical research ( Hariri and Holmes, 2015 ).
Increasing data highlight that highly debilitating stress comorbidities such as depression, anxiety disorders, PTSD and epilepsy share pathogenic mechanisms with stress dysfunction and between each other. These mechanisms are probably deeply connected and the structural and functional change caused by one disease triggers the other, despite these it is still not clear on the relationship between them ( Gold et al., 1988 ; de Kloet et al., 2005a , b ; Kanner, 2012 ). Considering this, we presented in this review a short glimpse of how stress is related to these CNS pathologies. For further review, we suggest to look into the literature cited here.
A significant percentage of patients with Major Depression (MD) have increased concentrations of cortisol in plasma, urine and cerebrospinal fluid, exaggerated cortisol response after ACTH hormone stimulation and hyperplasia of the pituitary and adrenal glands ( Gold et al., 1988 ; Juruena et al., 2004 ). In addition, one of the most consistent findings in neuropsychiatry is the reduction of hippocampal volume by 10%–15% in MD ( Sapolsky, 2000b ).
Chronic stressors in early life result in permanent epigenetic, endocrine, neural, immune and inflammatory changes, constituting a relevant risk factor for several neuropsychiatric diseases in adult life ( Xiong and Zhang, 2013 ; Zhang et al., 2013 ; Berens et al., 2017 ). Traumatic childhood experiences such as abuse, neglect and parental loss increase the incidence of psychiatric disorders, such as MD that boost to 59%–75% in adult life ( Widom et al., 2007 ) and anxiety disorders that are 1.9–3.6-fold more common in people who experience early life stress ( Fernandes and Osório, 2015 ). In epilepsy, stress can influence in multiple ways, often as seizure-precipitating but also increased the risk of epilepsy development ( van Campen et al., 2014 ). Additionally, the dysregulation of HPA axis in stress can trigger immunological responses that are considered as risk factor for Alzheimer’s disease ( Jeong et al., 2006 ; Caruso et al., 2018 ; Hoijemakers et al., 2016 ).
However, studies indicate that infancy coincides with a period referred as hyporesponsive period to stress (Stress Hyporesponsive Period—SHRP), which is supposed to be a period that is necessary for the proper development of the brain after birth ( Sapolsky and Meaney, 1986 ; de Kloet et al., 2005b ). It corresponds with a period of low peripheral concentration of glucocorticoids, in which a physiological response to mild stress (increased glucocorticoids and adrenaline, increased cardiovascular circulation, immune system modifications) does not occur. There is no consensus when exactly this period starts or how long it lasts, but it is suggested that it could last from around the 2nd to 12th postnatal day in rodents ( Sapolsky and Meaney, 1986 ) and up to 5 years in humans ( Gunnar and Donzella, 2002 ; Curley et al., 2011 ).
Interestingly, psychological ( Sapolsky and Meaney, 1986 ) and multimodal stressors ( Godoy et al., 2018 ) can disrupt the SHRP, turning the HPA-axis responsive to stressors earlier in development ( Cowan et al., 2016 ) and generating disruptions related psychopathologies ( Loi et al., 2014 ; Bath et al., 2017 ).
The effects of stress during early life (ELS) on the brain have been deeply studied (for review see Lupien et al., 2009 ; Lucassen et al., 2013 ; Krugers et al., 2017 ). ELS disrupts the proper development and function of limbic structures, leading to lifelong susceptibility to stress on behavior and cognition as well as on the reward system ( Peña et al., 2017 ). More recently it has been demonstrated that ELS led to an early emergence of timed developmental suppression of fear behavior that correlates to an early maturation ( Bath et al., 2016 ).
Depression-anxiety comorbidity is strongly associated with impairment in health, as well as in cognitive and emotional functions ( Kroenke et al., 2007 ). Similarly, chronic treatment with corticosterone not only generates depressive-like symptoms but also induces amygdala hypertrophy and increases anxiogenic behavioral responses ( Mitra and Sapolsky, 2008 ). In humans, individual differences in amygdala reactivity to threat-related facial expressions predict vulnerability to stress, such as subjects with hyperactivation of the amygdala are more likely to experience depression and anxiety symptoms ( Yang et al., 2008 ; Swartz et al., 2015 ).
Also, hyperactivation of LC-NE is related to neuropsychiatric disorders such as PTSD and MD, the activation of this system out of proper context may lead to hyperarousal, loss of concentration, restlessness and impaired focused attention, which are characteristic symptoms of stress-related psychiatric disorders ( Southwick et al., 1999 ; Wong et al., 2000 ). For instance, PTSD is associated with increased rates of anxiety and depression ( Nemeroff et al., 2006 ). However, some clinical investigations reported low levels of cortisol in PTSD patients, while individuals with anxiety disorders or depression show an increase of cortisol response ( Daskalakis et al., 2013 ; Zoladz and Diamond, 2016 ; Zorn et al., 2017 ).
Actually, the literature reports alterations in the HPA axis in PTSD patients and from the study by Mason et al. (1986) , in which low basal levels of cortisol were detected in PTSD patients, more and more studies corroborated this finding ( Yehuda et al., 1990 , 1993 ; Brand et al., 2006 ; Rohleder and Karl, 2006 ; Wessa et al., 2006 ). However, there are studies also showing no differences ( Baker et al., 1999 ; Duval et al., 2004 ; Yehuda et al., 2004a , b ; Otte et al., 2007 ) as well as increased ( Bremner et al., 2003 ; Inslicht et al., 2006 ; Lindauer et al., 2006 ) levels of basal cortisol in PTSD patients. Because this topic remains controversial in the literature, more insights such as those presented in Zoladz and Diamond (2013) are recommended. Alterations in other compartments of the HPA axis were also identified in PTSD patients such as enhanced GR sensitivity and HPA axis enhanced negative feedback ( Grossman et al., 2003 ; Duval et al., 2004 ; Yehuda et al., 2004b ; Rohleder and Karl, 2006 ), elevated levels of CRH in the cerebrospinal fluid ( Bremner et al., 1997 ; Baker et al., 1999 ) and reduced release of ACTH after CRH ( Smith et al., 1989 ) and CCK4 ( Kellner et al., 2000 ) administration. Animal models of PTSD can directly address these questions, but it is known that different types of aversive exposure in PTSD models (e.g., predator, shock, odor) or even the controllability of stress interact in a complex neuro-gene-environment system ( Daskalakis et al., 2013 ; Zoladz and Diamond, 2013 ; Homberg et al., 2016 ; Maier and Seligman, 2016 ).
Actually, exposure to severe stressors such as urban violence, sexual abuse, combat in war, disasters and many others, is believed to be associated to development of PTSD, leading individuals to present physiological and behavioral alterations including nightmares, hypervigilance, flashbacks of the trauma and sleep disturbances (DSM-V, Zoladz and Diamond, 2013 ; Yehuda et al., 2015 ). Although a positive correlation has been reported between the severity of the trauma and PTSD symptomatology in veteran soldiers ( Snow et al., 1988 ; Sutker et al., 1993 ), as well as in civilians ( Shore et al., 1986 ; Pynoos et al., 1993 ; Mollica et al., 1998 ), this is not always the case ( Başoglu et al., 1994 ; Schnyder et al., 2001 ; Stevenson et al., 2001 ). It has also been reported that the type of stressor seems to play a bigger role than its severity ( Kessler et al., 1995 ; Amir et al., 1996 ).
Interestingly, not everybody develops PTSD after trauma exposure, which suggests that other factors (despite the trauma severity and type), such as socioeconomic profile, psychiatry disorder history, substance abuse, immune system, genetics and epigenetics play a role on the susceptibility for PTSD. Actually, there are evidences showing that the interplay between environment and genetics (and epigenetics) are risk factors for PTSD development ( Mehta and Binder, 2012 ; Wilker and Kolassa, 2012 ; DiGangi et al., 2013 ).
In patients with epilepsy, stress is usually reported as one of the major seizure precipitants ( Frucht et al., 2000 ; Spector et al., 2000 ; Nakken et al., 2005 ; Sperling et al., 2008 ; van Campen et al., 2012 ; Ferlisi and Shorvon, 2014 ). In adults, there is a positive correlation between stress and frequency of epileptic seizures ( Swinkels et al., 1998 ; Moshe et al., 2008 ). Similarly, children with epilepsy living close to conflict/war areas have a higher frequency of seizures in comparison to children living in areas without conflict ( Bosnjak et al., 2002 ). Epileptogenesis is a multi-stage process that can begin early in life and may be negatively influenced by stress ( Joëls, 2009 ), and it is now suggested that early life stress can create permanent vulnerability to the development of epilepsy ( Huang, 2014 ).
Therefore, many evidences indicate that stress is relevant in the phases of epileptogenesis, both in adults and young people. Corticosterone plays a contributory role in the epileptogenic process in animal models of epilepsy ( Karst et al., 1999 ; Kumar et al., 2007 ; Hemanth Kumar et al., 2012 ; Salzberg et al., 2007 ; Koe et al., 2009 ; Castro et al., 2012 ; Jones, 2013 ; van Campen et al., 2014 ), and there are several actions of corticosterone in the brain that could mediate such effects. This could occur indirectly through the genomic effects of glucocorticoids, resulting in alterations of networks associated with seizures, or by direct non-genomic effects of these on the excitability of the limbic system ( Joëls, 2009 ).
Other pathologies affect the peripheral nervous system and many different organs. The CRH circuitry connecting LC-NE and PVN is strategically positioned so it may control autonomic responses to visceral stimuli and may underlie the co-morbidity of pelvic visceral and behavioral symptoms observed in many stress-related disorders ( Valentino et al., 1999 ). Although most immediate responses coordinated by SAM axis are important for survival, when levels of circulating catecholamines are maintained elevated for prolonged periods of time, they can lead to different pathologies. Those pathologies may primarily affect the cardiovascular system (cardiac arrhythmias, angina, congestive, heart failure, hypertension and/or cardiac hypertrophy; Antunes-Rodrigues et al., 2005 ; Lundberg, 2005 ; McEwen, 2007 ; Dünser and Hasibeder, 2009 ; Zhang and Anderson, 2014 ; Carter and Goldstein, 2015 ; Tank and Lee Wong, 2015 ; Breen et al., 2016 ).
In the past decades identification of neuronal circuits associated to stress, as well as their interaction with mediators over time, was critical not only for understanding physiological stress responses, but also to understand their clinical implications. Stress-related brain disorders are extremely prevalent, so identification of mechanisms related to stress and consequently the potential development of new pharmacological therapeutic approaches are necessary and urgent.
As an example, pre-clinical studies on psychopathology-related topics are being done with classic GR antagonist RU486 ( Arp et al., 2016 ) as well as using newly developed compounds, which modulate the GR in a tissue-gene specific fashion. These compounds are the so-called selective glucocorticoid receptor modulators (SGRMs) which upon binding to the GR promote a differential conformation of the receptor leading to differential recruitment of corregulators and therefore enhancing or hampering gene expression in a cell-tissue specific manner. Thus, the same compound can induce agonist- and antagonist-like effects, for instance the SGRMs C108297 acts as an antagonist in neurogenesis related processes such as proliferation and survival of hippocampal neurons, and as an agonist in fear memory retention on the avoidance behavior task ( Zalachoras et al., 2013 ). In the other hand the C118335 compound acts as an antagonist in the same task whereas showing agonist effects on plasmatic corticosterone levels ( Atucha et al., 2015 ).
From our own experience the Wistar Audiogenic Rat (WAR) strain is a genetically selected experimental epilepsy model, which displays after 56 generations of inbreeding, not only seizure-associated behaviors and electrophysiological alterations, but also comorbidities which includes high anxiety, hyperactive HPA axis, adrenal medulla hyperplasia, ectopic beats, high blood pressure, tachychardia and central respiratory alterations ( Fazan et al., 2011 , 2015 ; Umeoka et al., 2011 ; Granjeiro et al., 2016 ; Totola et al., 2017 ), for a recent comprehensive review on the WAR strain see Garcia-Cairasco et al. (2017) . Further experiments are therefore needed in order to evaluate the pharmacological profile of new anti-epileptic, anxiolytic or even anti-depressive drugs, using as a model of comorbidities of the WAR strain.
In the clinical scenario a final comment can be done, when referring to the additional complexity associated, for example, to the presence of comorbid neurological and neuropsychiatric conditions, such as, for example, the epilepsies, autism and mood disorders, recognizing that we are talking about network disorders ( Kanner et al., 2017 ). In the particular case of depression, anxiety and epilepsy, Kanner (2017) and Nogueira et al. (2017) highlight how strong are the bidirectional links that exist between epilepsy and depression, anxiety and epilepsy. Therefore stressors, which work as triggers, such as those cited in the current review, are common to all the situations. On the other hand, Rayner (2017) highlights that complex cognitive networks associated to depression interact so strongly with epilepsy related networks, in a way that the difficulties with diagnosis and treatment increase, as soon as we recognize that those networks share common structures and mechanisms. One way to overcome this, in order to make relevant and reliable contributions in this field, is the construction of algorhythms from computational neuroscience modeling, where actual data, either from basic science or from human clinical settings are used to generate predictions with translational value. In that context, recently Spiga et al. (2017) developed a mathematical model of the adrenal steroidogenic regulatory network that accounts for key regulatory processes occurring at different timescales and using the model to predict the time evolution of steroidogenesis in response to physiological ACTH alterations (basal pulses vs. inflammatory stress). In brief, these authors showed in a rat model, that although the steroidogenic regulatory network architecture is sufficient to respond to both small and large ACTH perturbations, coupling the regulatory network with the immune system would explain dissociated dynamics between ACTH and glucocorticoids observed when inflammatory stress is present.
Concluding Remarks
We are able to adapt to the dynamic and challenging environment we live in, as well as to unexpected life events we face every now and then. Examples are endless and, most of the time, we can overcome these events. We are able to do so because the existence of quite complex networks, which integrate body and brain, in order to enhance performance, promote adaptation and ultimately survival, the stress system .
A diversity of brain areas integrates sensorial, physiological and emotional signs. When different brain networks interpret these signs as a threat (real or potential), a series of responses follow, increasing performance to deal with the situation and retain that information to better cope with similar situations in the future, characterizing the stress response . The stress response, by means of mediator molecules, promotes short and long-term alterations in cellular excitability, as well as in neuronal and synaptic plasticity leading to transient and/or permanent changes in physiology and behavior.
The downside of the stress system is that sometimes it is not able to overcome the environmental, physiological or emotional demand. It mostly happens in occasions in which the demand is extremely strong, chronic and/or during development.
The aim of this review article was to bring comprehensive basic concepts about the stress system such as history of stress research, neuroanatomy, major effectors of the stress response, time domains of stress and the clinical implication of malfunction might have over the susceptibility to the development of increasingly-common brapdin disorders.
Author Contributions
LDG, MTR and PD-P wrote the manuscript draft. NG-C and EHLU conceptualize, wrote and corrected the manuscript. LDG and MTR contributed equally to this work.
This work was funded by the National Council for Scientific and Technological Development, Coordination for Improvement of Higher Education Personnel, Coordenação de Aperfeiçoamento de Pessoal de Nível Superior (CAPES) PROEX-Physiology and the São Paulo Research Foundation (Fundação de Amparo à Pesquisa do Estado de São Paulo, FAPESP 2007/50261-4). Currently LDG holds FAPESP fellowship 2014/17959-1 and 2017/11339-0, MTR holds Conselho Nacional de Desenvolvimento Científico e Tecnológico (CNPq) fellowship 142451/2014-2, PD-P holds CAPES fellowship, NG-C holds CNPQ Research fellowship 305883/2014-3 and EHLU holds FAPESP fellowships 2016/21369-0, 2015/18773-1.
Conflict of Interest Statement
The authors declare that the research was conducted in the absence of any commercial or financial relationships that could be construed as a potential conflict of interest.
Acknowledgments
We would like to thank all students enrolled in the Course on Behavioural, Neuro-Psychopharmacological, Endocrine and Molecular Aspects of Stress (RFI-5803) held at the Graduate Program in Physiology (PROEX-CAPES) of the FMRP-USP in July 2015, who contributed with insightful discussions. To the Course Staff Marian Joëls, Ron de Kloet, Mário Juruena, Marcus Brandão, Samia Joca and Cristiane Baes for their inspirational lectures.
Abercrombie, E. D., Keefe, K. A., DiFrischia, D. S., and Zigmond, M. J. (1989). Differential effect of stress on in vivo dopamine release in striatum, nucleus accumbens, and medial frontal cortex. J. Neurochem. 52, 1655–1658. doi: 10.1111/j.1471-4159.1989.tb09224.x
PubMed Abstract | CrossRef Full Text | Google Scholar
Aires, M. M. (2012). Fisiologia. 4th Edn. Rio de Janeiro: Guanabara-Koogan.
Alt, S. R., Turner, J. D., Klok, M. D., Meijer, O. C., Lakke, E. A. J. F., Derijk, R. H., et al. (2010). Differential expression of glucocorticoid receptor transcripts in major depressive disorder is not epigenetically programmed. Psychoneuroendocrinology 35, 544–556. doi: 10.1016/j.psyneuen.2009.09.001
Amaral, D. G., and Witter, M. P. (1989). The three-dimensional organization of the hippocampal formation: a review of anatomical data. Neuroscience 31, 571–591. doi: 10.1016/0306-4522(89)90424-7
Amir, M., Kaplan, Z., and Kotler, M. (1996). Type of trauma, severity of posttraumatic stress disorder core symptoms, and sssociated features. J. Gen. Psychol. 123, 341–351. doi: 10.1080/00221309.1996.9921286
Andersen, P., Soleng, A. F., and Raastad, M. (2000). The hippocampal lamella hypothesis revisited. Brain Res. 886, 165–171. doi: 10.1016/s0006-8993(00)02991-7
Angeli, A., Masera, R. G., Sartori, M. L., Fortunati, N., Racca, S., Dovio, A., et al. (1999). Modulation by cytokines of glucocorticoid action. Ann. N Y Acad. Sci. 876, 210–220. doi: 10.1111/j.1749-6632.1999.tb07641.x
Anisman, H., Zaharia, M. D., Meaney, M. J., and Meralis, Z. (1998). Do early-life events permantently alter behavior and hormonal responses to stressors? Int. J. Dev. Neurosci. 16, 149–164. doi: 10.1016/s0736-5748(98)00025-2
Antunes-Rodrigues, J., Moreira, A. C., Elias, L. L. K., and Castro, M. (2005). Neuroendocrinologia: Básica e Aplicada. 1st Edn. Rio de Janeiro: Guanabra-Koogan.
Armario, A., Daviu, N., Muñoz-Abellán, C., Rabasa, C., Fuentes, S., Belda, X., et al. (2012). What can we know from pituitary-adrenal hormones about the nature and consequences of exposure to emotional stressors? Cell. Mol. Neurobiol. 32, 749–758. doi: 10.1007/s10571-012-9814-6
Arnsten, A. F. T. (2009). Stress signaling pathways that impair prefrontal cortex structure and function. Nat. Rev. Neurosci. 10, 410–422. doi: 10.1038/nrn2648
Arp, J. M., Ter Horst, J. P., Loi, M., den Blaauwen, J., Bangert, E., Fernández, G., et al. (2016). Blocking glucocorticoid receptors at adolescent age prevents enhanced freezing between repeated cue-exposures after conditioned fear in adult mice raised under chronic early life stress. Neurobiol. Learn. Mem. 133, 30–38. doi: 10.1016/j.nlm.2016.05.009
Atucha, E., Zalachoras, I., van den Heuvel, J. K., van Weert, L. T. C. M., Melchers, D., Mol, I. M., et al. (2015). A mixed glucocorticoid/mineralocorticoid selective modulator with dominant antagonism in the male rat brain. Endocrinology 156, 4105–4114. doi: 10.1210/en.2015-1390
Audet, M. C., Jacobson-Pick, S., Wann, B. P., and Anisman, H. (2011). Social defeat promotes specific cytokine variations within the prefrontal cortex upon subsequent aggressive or endotoxin challenges. Brain Behav. Immun. 25, 1197–1205. doi: 10.1016/j.bbi.2011.03.010
Audet, M. C., Mangano, E. N., and Anisman, H. (2010). Behavior and pro-inflammatory cytokine variations among submissive and dominant mice engaged in aggressive encounters: moderation by corticosterone reactivity. Front. Behav. Neurosci. 4:156. doi: 10.3389/fnbeh.2010.00156
Audet, M.-C., McQuaid, R. J., Merali, Z., and Anisman, H. (2014). Cytokine variations and mood disorders: influence of social stressors and social support. Front. Neurosci. 8:416. doi: 10.3389/fnins.2014.00416
Bagot, R. C., Parise, E. M., Peña, C. J., Zhang, H.-X., Maze, I., Chaudhury, D., et al. (2015). Ventral hippocampal afferents to the nucleus accumbens regulate susceptibility to depression. Nat. Commun. 6:7062. doi: 10.1038/ncomms8626
Bains, J. S., Wamsteeker Cusulin, J. I., and Inoue, W. (2015). Stress-related synaptic plasticity in the hypothalamus. Nat. Rev. Neurosci. 16, 377–388. doi: 10.1038/nrn3881
Baker, D. G., West, S. A., Nicholson, W. E., Ekhator, N. N., Kasckow, J. W., Hill, K. K., et al. (1999). Serial CSF corticotropin-releasing hormone levels and adrenocortical activity in combat veterans with posttraumatic stress disorder. Am. J. Psychiatry 156, 585–588.
PubMed Abstract | Google Scholar
Banks, W. A. (2006). The blood-brain barrier in psychoneuroimmunology. Neurol. Clin. 24, 413–419. doi: 10.1016/j.ncl.2006.03.009
Barbas, H., and Blatt, G. J. (1995). Topographically specific hippocampal projections target functionally distinct prefrontal areas in the rhesus monkey. Hippocampus 5, 511–533. doi: 10.1002/hipo.450050604
Başoglu, M., Paker, M., Ozmen, E., Taşdemir, O., and Sahin, D. (1994). Factors related to long-term traumatic stress responses in survivors of torture in Turkey. JAMA 272, 357–363. doi: 10.1001/jama.1994.03520050037027
Bassareo, V., De Luca, M. A., and Di Chiara, G. (2002). Differential expression of motivational stimulus properties by dopamine in nucleus accumbens shell versus core and prefrontal cortex. J. Neurosci. 22, 4709–4719. doi: 10.1523/JNEUROSCI.22-11-04709.2002
Bath, K., Manzano-Nieves, G., and Goodwill, H. (2016). Early life stress accelerates behavioral and neural maturation of the hippocampus in male mice. Horm. Behav. 82, 64–71. doi: 10.1016/j.yhbeh.2016.04.010
Bath, K. G., Russo, S. J., Pleil, K. E., Wohleb, E. S., Duman, R. S., and Radley, J. J. (2017). Circuit and synaptic mechanisms of repeated stress: perspectives from differing contexts, duration, and development. Neurobiol. Stress 7, 137–151. doi: 10.1016/j.ynstr.2017.05.001
Bennur, S., Shankaranarayana Rao, B. S., Pawlak, R., Strickland, S., McEwen, B. S., and Chattarji, S. (2007). Stress-induced spine loss in the medial amygdala is mediated by tissue-plasminogen activator. Neuroscience 144, 8–16. doi: 10.1016/j.neuroscience.2006.08.075
Berens, A. E., Jensen, S. K. G., and Nelson, C. A. III. (2017). Biological embedding of childhood adversity: from physiological mechanisms to clinical implications. BMC Med. 15:135. doi: 10.1186/s12916-017-0895-4
Berkenbosch, F., van Oers, J., del Rey, A., Tilders, F., and Besedovsky, H. (1987). Corticotropin-releasing factor-producing neurons in the rat activated by interleukin-1. Science 238, 524–526. doi: 10.1126/science.2443979
Boron, W. F., and Boulpaep, E. L. (2009). Medical Physiology: A Cellular and Molecular Approach. 2nd Edn. Philadelphia, PA: Saunders Elsevier.
Google Scholar
Borski, R. J. (2000). Nongenomic membrane actions of glucocorticoids in vertebrates. Trends Endocrinol. Metab. 11, 427–436. doi: 10.1016/s1043-2760(00)00325-8
Bosnjak, J., Vukovic-Bobic, M., and Mejaski-bosnjak, V. (2002). Behavior effect of war on the occurrence of epileptic seizures in children. Epilepsy Behav. 3, 502–509. doi: 10.1016/s1525-5050(02)00602-9
Bowman, K. M. (1930). Bodily changes in pain, hunger, fear and rage. (By Walter B. Cannon, 404 pages. New York: D. Appleton and Co., 1929) Am. J. Psychiatry 86, 770–771. doi: 10.1176/ajp.86.4.770
CrossRef Full Text
Brand, S. R., Engel, S. M., Canfield, R. L., and Yehuda, R. (2006). The effect of maternal PTSD following in utero trauma exposure on behavior and temperament in the 9-month-old infant. Ann. N Y Acad. Sci. 1071, 454–458. doi: 10.1196/annals.1364.041
Breen, M. S., Beliakova-Bethell, N., Mujica-Parodi, L. R., Carlson, J. M., Ensign, W. Y., Woelk, C. H., et al. (2016). Acute psychological stress induces short-term variable immune response. Brain Behav. Immun. 53, 172–182. doi: 10.1016/j.bbi.2015.10.008
Bremner, J. D., Licinio, J., Darnell, A., Krystal, J. H., Owens, M. J., Southwick, S. M., et al. (1997). Elevated CSF corticotropin-releasing factor concentrations in posttraumatic stress disorder. Am. J. Psychiatry 154, 624–629. doi: 10.1176/ajp.154.5.624
Bremner, J. D., Vythilingam, M., Vermetten, E., Adil, J., Khan, S., Nazeer, A., et al. (2003). Cortisol response to a cognitive stress challenge in posttraumatic stress disorder (PTSD) related to childhood abuse. Psychoneuroendocrinology 28, 733–750. doi: 10.1016/s0306-4530(02)00067-7
Brioni, J. D., Nagahara, A. H., and McGaugh, J. L. (1989). Involvement of the amygdala GABAergic system in the modulation of memory storage. Brain Res. 487, 105–112. doi: 10.1016/0006-8993(89)90945-1
Brischoux, F., Chakraborty, S., Brierley, D. I., and Ungless, M. A. (2009). Phasic excitation of dopamine neurons in ventral VTA by noxious stimuli. Proc. Natl. Acad. Sci. U S A 106, 4894–4899. doi: 10.1073/pnas.0811507106
Burgos-Robles, A., Kimchi, E. Y., Izadmehr, E. M., Porzenheim, M. J., Ramos-Guasp, W. A., Nieh, E. H., et al. (2017). Amygdala inputs to prefrontal cortex guide behavior amid conflicting cues of reward and punishment. Nat. Neurosci. 20, 824–835. doi: 10.1038/nn.4553
Buschdorf, J. P., and Meaney, M. J. (2015). “Epigenetics/programming in the HPA axis,” in Comprehensive Physiology , Hoboken, NJ: John Wiley and Sons, Inc., 87–110.
Cannon, W. B. (1914). The interrelations of emotions as suggested by recent physiological researches. Am. J. Psychol. 25, 256–282. doi: 10.2307/1413414
CrossRef Full Text | Google Scholar
Cannon, W. B. (1915). Bodily Changes in Pain, Hunger, Fear and Rage. An Account of Recent Researches Into the Function of Emotional Excitement. New York, NY and London: Appleton Co.
Cannon, W. B. (1929). Organization for physiological homeostasis. Physiol. Rev. 9, 399–431. doi: 10.1152/physrev.1929.9.3.399
Canteras, N. S., and Swanson, L. W. (1992). Projections of the ventral subiculum to the amygdala, septum, and hypothalamus: a PHAL anterograde tract-tracing study in the rat. J. Comp. Neurol. 324, 180–194. doi: 10.1002/cne.903240204
Carter, J. R., and Goldstein, D. S. (2015). Sympathoneural and adrenomedullary responses to mental stress. Compr. Physiol. 5, 119–146. doi: 10.1002/cphy.c140030
Caruso, A., Nicoletti, F., Mango, D., Saidi, A., Orlando, R., and Scaccianoce, S. (2018). Stress as risk fator for Alzheimer’s disease. Pharmacol. Res. 132, 130–134. doi: 10.1016/j.phrs.2018.04.017
PubMed Abstract | CrossRef Full Text
Cassens, G., Kuruc, A., Roffman, M., Orsulak, P. J., and Schildkraut, J. J. (1981). Alterations in brain norepinephrine metabolism and behavior induced by environmental stimuli previously paired with inescapable shock. Behav. Brain Res. 2, 387–407. doi: 10.1016/0166-4328(81)90020-6
Castro, O. W., Santos, V. R., Pun, R. Y. K., McKlveen, J. M., Batie, M., Holland, K. D., et al. (2012). Impact of corticosterone treatment on spontaneous seizure frequency and epileptiform activity in mice with chronic epilepsy. PLoS One 7:e46044. doi: 10.1371/journal.pone.0046044
Chappell, P. B., Smith, M. A., Kilts, C. D., Bissette, G., Ritchie, J., Anderson, C., et al. (1986). Alterations in corticotropin-releasing factor-like immunoreactivity in discrete rat brain regions after acute and chronic stress. J. Neurosci. 6, 2908–2914. doi: 10.1523/JNEUROSCI.06-10-02908.1986
Chaudhury, D., Walsh, J. J., Friedman, A. K., Juarez, B., Ku, S. M., Koo, J. W., et al. (2012). Rapid regulation of depression-related behaviours by control of midbrain dopamine neurons. Nature 493, 532–536. doi: 10.1038/nature11713
Choi, D. C., Furay, A. R., Evanson, N. K., Ostrander, M. M., Ulrich-Lai, Y. M., and Herman, J. P. (2007). Bed nucleus of the stria terminalis subregions differentially regulate hypothalamic—pituitary—adrenal axis activity: implications for the integration of limbic inputs. J. Neurosci. 27, 2025–2034. doi: 10.1523/JNEUROSCI.4301-06.2007
Christoffel, D. J., Golden, S. A., Dumitriu, D., Robison, A. J., Janssen, W. G., Ahn, H. F., et al. (2011). IκB kinase regulates social defeat stress-induced synaptic and behavioral plasticity. J. Neurosci. 31, 314–321. doi: 10.1523/JNEUROSCI.4763-10.2011
Chrousos, G. P. (2009). Stress and disorders of the stress system. Nat. Rev. Endocrinol. 5, 374–381. doi: 10.1038/nrendo.2009.106
Cole, B. J., and Koob, G. F. (1988). Propranolol antagonizes the enhanced conditioned fear produced by corticotropin releasing factor. J. Pharmacol. Exp. Ther. 247, 902–910.
Covington, H. E. III., Maze, I., LaPlant, Q. C., Vialou, V. F., Ohnishi, Y. N., Berton, O., et al. (2009). Antidepressant actions of histone deacetylase inhibitors. J. Neurosci. 29, 11451–11460. doi: 10.1523/JNEUROSCI.1758-09.2009
Covington, H. E. III., Maze, I., Sun, H., Bomze, H. M., DeMaio, K. D., Wu, E. Y., et al. (2011). A role for repressive histone methylation in cocaine-induced vulnerability to stress. Neuron 71, 656–670. doi: 10.1016/j.neuron.2011.06.007
Cowan, C. S. M., Callaghan, B. L., Kan, J. M., and Richardson, R. (2016). The lasting impact of early-life adversity on individuals and their descendants: potential mechanisms and hope for intervention. Genes Brain Behav. 15, 155–168. doi: 10.1111/gbb.12263
Crestani, C. C., Alves, F. H., Gomes, F. V., Resstel, L. B., Correa, F. M., and Herman, J. P. (2013). Mechanisms in the bed nucleus of the stria terminalis involved in control of autonomic and neuroendocrine functions: a review. Curr. Neuropharmacol. 11, 141–159. doi: 10.2174/1570159X11311020002
Cullinan, W. E., Herman, J. P., Battaglia, D. F., Akil, H., and Watson, S. J. (1995). Pattern and time course of immediate early gene expression in rat brain following acute stress. Neuroscience 64, 477–505. doi: 10.1016/0306-4522(94)00355-9
Cullinan, W. E., Herman, J. P., and Watson, S. J. (1993). Ventral subicular interactions with the hypothalamic paraventricular nucleus: evidence for a relay in the bed nucleus of the stria terminalis. J. Comp. Neurol. 332, 1–20. doi: 10.1002/cne.903320102
Cunningham, E. T. Jr., and Sawchenko, P. E. (1988). Anatomical specificity of noradrenergic inputs to the paraventricular and supraoptic nuclei of the rat hypothalamus. J. Comp. Neurol. 274, 60–76. doi: 10.1002/cne.902740107
Curley, J. P., Jensen, C. L., Mashoodh, R., and Champagne, F. A. (2011). Social influences on neurobiology and behavior: epigenetic effects during development. Psychoneuroendocrinology 36, 352–371. doi: 10.1016/j.psyneuen.2010.06.005
Dallman, M. F. (2003). Stress by any other name …? Horm. Behav. 43, 18–20. doi: 10.1016/s0018-506x(02)00034-x
Dallman, M. F. (2005). Fast glucocorticoid actions on brain: back to the future. Front. Neuroendocrinol. 26, 103–108. doi: 10.1016/j.yfrne.2005.08.001
Daskalakis, N. P., Yehuda, R., and Diamond, D. M. (2013). Animal models in translational studies of PTSD. Psychoneuroendocrinology 38, 1895–1911. doi: 10.1016/j.psyneuen.2013.06.006
Datson, N. A., Morsink, M. C., Meijer, O. C., and de Kloet, E. R. (2008). Central corticosteroid actions: search for gene targets. Eur. J. Pharmacol. 583, 272–289. doi: 10.1016/j.ejphar.2007.11.070
Davidson, R. J., and McEwen, B. S. (2012). Social influences on neuroplasticity: stress and interventions to promote well-being. Nat. Neurosci. 15, 689–695. doi: 10.1038/nn.3093
Davies, K. J. A. (2016). Adaptive homeostasis. Mol. Aspects Med. 49, 1–7. doi: 10.1016/j.mam.2016.04.007
Day, T. A. (2005). Defining stress as a prelude to mapping its neurocircuitry: no help from allostasis. Prog. Neuropsychopharmacol. Biol. Psychiatry 29, 1195–1200. doi: 10.1016/j.pnpbp.2005.08.005
Dayas, C. V., Buller, K. M., Crane, J. W., Xu, Y., and Day, T. A. (2001). Stressor categorization: acute physical and psychological stressors elicit distinctive recruitment patterns in the amygdala and in medullary noradrenergic cell groups. Eur. J. Neurosci. 14, 1143–1152. doi: 10.1046/j.0953-816x.2001.01733.x
de Kloet, E. R. (2013). Functional profile of the binary brain corticosteroid receptor system: mediating, multitasking, coordinating, integrating. Eur. J. Pharmacol. 719, 53–62. doi: 10.1016/j.ejphar.2013.04.053
de Kloet, E. R., Joëls, M., and Holsboer, F. (2005a). Stress and the brain: from adaptation to disease. Nat. Rev. Neurosci. 6, 463–475. doi: 10.1038/nrn1683
de Kloet, E. R., Sibug, R. M., Helmerhorst, F. M., and Schmidt, M. (2005b). Stress, genes and the mechanism of programming the brain for later life. Neurosci. Biobehav. Rev. 29, 271–281. doi: 10.1016/j.neubiorev.2004.10.008
de Kloet, E. R., and Sarabdjitsingh, R. A. (2008). Everything has rhythm: focus on glucocorticoid pulsatility. Endocrinology 149, 3241–3243. doi: 10.1210/en.2008-0471
de Kloet, E. R., Sutanto, W., Rots, N., van Haarst, A., van den Berg, D., Oitzl, M., et al. (1991). Plasticity and function of brain corticosteroid receptors during aging. Acta Endocrinol. 125, 65–72.
De Oca, B. M., DeCola, J. P., Maren, S., and Fanselow, M. S. (1998). Distinct regions of the periaqueductal gray are involved in the acquisition and expression of defensive responses. J. Neurosci. 18, 3426–3432. doi: 10.1523/JNEUROSCI.18-09-03426.1998
Deak, T., Bordner, K. A., McElderry, N. K., Barnum, C. J., Blandino, P. Jr., Deak, M. M., et al. (2005). Stress-induced increases in hypothalamic IL-1: a systematic analysis of multiple stressor paradigms. Brain Res. Bull. 64, 541–556. doi: 10.1016/j.brainresbull.2004.11.003
Dedovic, K., Duchesne, A., Andrews, J., Engert, V., and Pruessner, J. C. (2009). The brain and the stress axis: the neural correlates of cortisol regulation in response to stress. Neuroimage 47, 864–871. doi: 10.1016/j.neuroimage.2009.05.074
Dempsey, B., Le, S., Turner, A., Bokiniec, P., Ramadas, R., Bjaalie, J. G., et al. (2017). Mapping and analysis of the connectome of sympathetic premotor neurons in the rostral ventrolateral medulla of the rat using a volumetric brain atlas. Front. Neural Circuits 11:9. doi: 10.3389/fncir.2017.00009
Di, S., Malcher-Lopes, R., Halmos, K. C., and Tasker, J. G. (2003). Nongenomic glucocorticoid inhibition via endocannabinoid release in the hypothalamus: a fast feedback mechanism. J. Neurosci. 23, 4850–4857. doi: 10.1523/JNEUROSCI.23-12-04850.2003
Di, S., Malcher-Lopes, R., Marcheselli, V. L., Bazan, N. G., and Tasker, J. G. (2005). Rapid glucocorticoid-mediated endocannabinoid release and opposing regulation of glutamate andalfa-aminobutyric acid inputs to hypothalamic magnocellular neurons. Endocrinology 146, 4292–4301. doi: 10.1210/en.2005-0610
Diamond, D. M., Campbell, A. M., Park, C. R., Halonen, J., and Zoladz, P. R. (2007). The temporal dynamics model of emotional memory processing: a synthesis on the neurobiological basis of stress-induced amnesia, flashbulb and traumatic memories and the yerkes-dodson law. Neural Plast. 2007:60803. doi: 10.1155/2007/60803
DiGangi, J., Guffanti, G., McLaughlin, K. A., and Koenen, K. C. (2013). Considering trauma exposure in the context of genetics studies of posttraumatic stress disorder: a systematic review. Biol. Mood Anxiety Disord. 3:2. doi: 10.1186/2045-5380-3-2
Dilgen, J., Tejeda, H. A., and O’Donnell, P. (2013). Amygdala inputs drive feedforward inhibition in the medial prefrontal cortex. J. Neurophysiol. 110, 221–229. doi: 10.1152/jn.00531.2012
Dioro, D., Viau, V., and Meaney, M. J. (1993). The role of the medial prefrontal cortex (cingulate gyrus) in the regulation of hypothalamic-pituitary-adrenal responses to stress. J. Neurosci. 13, 3839–3847. doi: 10.1523/JNEUROSCI.13-09-03839.1993
Do-Monte, F. H., Quiñones-Laracuente, K., and Quirk, G. J. (2015). A temporal shift in the circuits mediating retrieval of fear memory. Nature 519, 460–463. doi: 10.1038/nature14030
Dünser, M. W., and Hasibeder, W. R. (2009). Sympathetic overstimulation during critical illness: adverse effects of adrenergic stress. J. Intensive Care Med. 24, 293–316. doi: 10.1177/0885066609340519
Duval, F., Crocq, M. A., Guilon, M. S., Mokrani, M. C., Monreal, J., Bailey, P., et al. (2004). Increased adrenocorticotropin suppression after dexamethasone administration in sexually abused adolescents with posttraumatic stress disorder. Ann. N Y Acad. Sci. 1032, 273–275. doi: 10.1196/annals.1314.036
Duvarci, S., and Paré, D. (2007). Glucocorticoids enhance the excitability of principal basolateral amygdala neurons. J. Neurosci. 27, 4482–4491. doi: 10.1523/JNEUROSCI.0680-07.2007
Engeland, W. C., and Arnhold, M. M. (2005). Neural circuitry in the regulation of adrenal corticosterone rhythmicity. Endocrine 28, 325–332. doi: 10.1385/endo:28:3:325
Euler, U. S. V. (1946). A specific sympathomimetic ergone in adrenergic nerve fibres (sympathin) and its relations to adrenaline and nor-adrenaline. Acta Physiol. 12, 73–97. doi: 10.1111/j.1748-1716.1946.tb00368.x
Evanson, N. K., Herman, J. P., Sakai, R. R., and Krause, E. G. (2010). Nongenomic actions of adrenal steroids in the central nervous system. J. Neuroendocrinol. 22, 846–861. doi: 10.1111/j.1365-2826.2010.02000.x
Fazan, R. Jr., de Oliveira, M., Oliveira, J. A., Salgado, H. C., and Garcia-Cairasco, N. (2011). Changes in autonomic control of the cardiovascular system in the Wistar audiogenic rat (WAR) strain. Epilepsy Behav. 22, 666–670. doi: 10.1016/j.yebeh.2011.09.010
Fazan, R. Jr., Silva, C. A., Oliveira, J. A., Salgado, H. C., Montano, N., and Garcia-Cairasco, N. (2015). Evaluation of cardiovascular risk factors in the wistar audiogenic rat (WAR) strain. PLoS One 10:e0129574. doi: 10.1371/journal.pone.0129574
Felix-Ortiz, A. C., Burgos-Robles, A., Bhagat, N. D., Leppla, C. A., and Tye, K. M. (2016). Bidirectional modulation of anxiety-related and social behaviors by amygdala projections to the medial prefrontal cortex. Neuroscience 321, 197–209. doi: 10.1016/j.neuroscience.2015.07.041
Fenoglio, K. A., Brunson, K. L., and Baram, T. Z. (2006). Hippocampal neuroplasticity induced by early-life stress: functional and molecular aspects. Front. Neuroendocrinol. 27, 180–192. doi: 10.1016/j.yfrne.2006.02.001
Ferlisi, M., and Shorvon, S. (2014). Seizure precipitants (triggering factors) in patients with epilepsy. Epilepsy Behav. 33, 101–105. doi: 10.1016/j.yebeh.2014.02.019
Fernandes, V., and Osório, F. L. (2015). Are there associations between early emotional trauma and anxiety disorders? Evidence from a systematic literature review and meta-analysis. Eur. Psychiatry 30, 756–764. doi: 10.1016/j.eurpsy.2015.06.004
Figueiredo, H. F., Bruestle, A., Bodie, B., Dolgas, C. M., and Herman, J. P. (2003). The medial prefrontal cortex differentially regulates stress-induced c-fos expression in the forebrain depending on type of stressor. Eur. J. Neurosci. 18, 2357–2364. doi: 10.1046/j.1460-9568.2003.02932.x
Frank, M. G., Watkins, L. R., and Maier, S. F. (2013). Stress-induced glucocorticoids as a neuroendocrine alarm signal of danger. Brain Behav. Immun. 33, 1–6. doi: 10.1016/j.bbi.2013.02.004
Frucht, M. M., Quigg, M., Schwaner, C., and Fountain, N. B. (2000). Distribution of seizure precipitants among epilepsy syndromes. Epilepsia 41, 1534–1539. doi: 10.1111/j.1528-1167.2000.01534.x
Gabbott, P. L. A., Warner, T. A., Jays, P. R. L., Salway, P., and Busby, S. J. (2005). Prefrontal cortex in the rat: projections to subcortical autonomic, motor, and limbic centers. J. Comp. Neurol. 492, 145–177. doi: 10.1002/cne.20738
Gaillet, S., Lachuer, J., Malaval, F., Assenmacher, I., and Szafarczyk, A. (1991). The involvement of noradrenergic ascending pathways in the stress-induced activation of ACTH and corticosterone secretions is dependent on the nature of stressors. Exp. Brain Res. 87, 173–180. doi: 10.1007/bf00228518
Garcia-Cairasco, N., Umeoka, E. H. L., and Cortes de Oliveira, J. A. (2017). The Wistar Audiogenic Rat (WAR) strain and its contributions to epileptology and related comorbidities: history and perspectives. Epilepsy Behav. 71, 250–273. doi: 10.1016/j.yebeh.2017.04.001
Geerling, J. C., Shin, J. W., Chimenti, P. C., and Loewy, A. D. (2010). Paraventricular hypothalamic nucleus: axonal projections to the brainstem. J. Comp. Neurol. 518, 1460–1499. doi: 10.1002/cne.22283
George, S. A., Knox, D., Curtis, A. L., Aldridge, J. W., Valentino, R. J., and Liberzon, I. (2013). Altered locus coeruleus-norepinephrine function following single prolonged stress. Eur. J. Neurosci. 37, 901–909. doi: 10.1111/ejn.12095
Godoy, L. D., Umeoka, E. H. L., Ribeiro, D. E., Santos, V. R., Antunes-Rodrigues, J., Joca, S. R. L., et al. (2018). Multimodal early-life stress induces biological changes associated to psychopathologies. Horm. Behav. 100, 69–80. doi: 10.1016/j.yhbeh.2018.03.005
Godsil, B. P., Kiss, J. P., and Spedding, M. (2013). The hippocampal-prefrontal pathway: the weak link in psychiatric disorders? Eur. J. Neuropsychopharmacol. 10, 1165–1181. doi: 10.1016/j.euroneuro.2012.10.018
Gold, P. W., Goodwin, F. K., and Chrousos, G. P. (1988). Clinical and biochemical manifestations of depression. N. Engl. J. Med. 319, 413–420. doi: 10.1056/nejm198808183190706
Goldstein, D. S., Eisenhofer, G., and Kopin, I. J. (2003). Sources and significance of plasma levels of catechols and their metabolites in humans. J. Pharmacol. Exp. Ther. 305, 800–811. doi: 10.1124/jpet.103.049270
Grace, A. A., Floresco, S. B., Goto, Y., and Lodge, D. J. (2007). Regulation of firing of dopaminergic neurons and control of goal-directed behaviors. Trends Neurosci. 30, 220–227. doi: 10.1016/j.tins.2007.03.003
Granjeiro, É. M., da Silva, G. S., Giusti, H., Oliveira, J. A., Glass, M. L., and Garcia-Cairasco, N. (2016). Behavioral, ventilatory and thermoregulatory responses to hypercapnia and hypoxia in the wistar audiogenic rat (WAR) strain. PLoS One 11:e0154141. doi: 10.1371/journal.pone.0154141
Grbesa, I., and Hakim, O. (2017). Genomic effects of glucocorticoids. Protoplasma 254, 1175–1185. doi: 10.1007/s00709-016-1063-y
Griffiths, B. B., and Hunter, R. G. (2014). Neuroepigenetics of stress. Neuroscience 275, 420–435. doi: 10.1016/j.neuroscience.2014.06.041
Groeneweg, F. L., Karst, H., de Kloet, E. R., and Joëls, M. (2012). Mineralocorticoid and glucocorticoid receptors at the neuronal membrane, regulators of nongenomic corticosteroid signalling. Mol. Cell. Endocrinol. 350, 299–309. doi: 10.1016/j.mce.2011.06.020
Grossman, R., Yehuda, R., New, A., Schmeidler, J., Silverman, J., Mitropoulou, V., et al. (2003). Dexamethasone suppression test findings in subjects with personality disorders: associations with posttraumatic stress disorder and major depression. Am. J. Psychiatry 160, 1291–1298. doi: 10.1176/appi.ajp.160.7.1291
Guimarães, S., and Moura, D. (2001). Vascular adrenoceptors: an update. Pharmacol. Rev. 53, 319–356.
Gunnar, M. R., and Donzella, B. (2002). Social regulation of the cortisol levels in early human development. Psychoneuroendocrinology 27, 199–220. doi: 10.1016/s0306-4530(01)00045-2
Härfstrand, A., Fuxe, K., Cintra, A., Agnati, L. F., Zini, I., Wikström, A. C., et al. (1986). Glucocorticoid receptor immunoreactivity in monoaminergic neurons of rat brain. Proc. Natl. Acad. Sci. U S A 83, 9779–9783. doi: 10.1073/pnas.83.24.9779
Hariri, A. R., and Holmes, A. (2015). Finding translation in stress research. Nat. Neurosci. 18, 1347–1352. doi: 10.1038/nn.4111
Heim, C., and Nemeroff, C. B. (2002). Neurobiology of early life stress: clinical studies. Semin. Clin. Neuropsychiatry 7, 147–159. doi: 10.1053/scnp.2002.33127
Heim, C., Newport, J., Heit, S., Graham, Y. P., Wilcox, M., Bonsall, R., et al. (2000). Pituitary-Adrenal and autonomic responses to stress in women after sexual and physical abuse in childhood. JAMA 284, 592–597. doi: 10.1001/jama.284.5.592
Heim, C., Newport, D. J., Mletzko, T., Miller, A. H., and Nemeroff, C. B. (2008). The link between childhood trauma and depression: insights from HPA axis studies in humans. Psychoneuroendocrinology 33, 693–710. doi: 10.1016/j.psyneuen.2008.03.008
Hemanth Kumar, B. S., Mishra, S. K., Rana, P., Singh, S., and Khushu, S. (2012). Neurodegenerative evidences during early onset of depression in CMS rats as detected by proton magnetic resonance spectroscopy at 7 T. Behav. Brain Res. 232, 53–59. doi: 10.1016/j.bbr.2012.03.011
Herman, J. P., Figueiredo, H., Mueller, N. K., Ulrich-Lai, Y., Ostrander, M. M., Choi, D. C., et al. (2003). Central mechanisms of stress integration: hierarchical circuitry controlling hypothalamo-pituitary-adrenocortical responsiveness. Front. Neuroendocrinol. 24, 151–180. doi: 10.1016/j.yfrne.2003.07.001
Herman, J. P., and Mueller, N. K. (2006). Role of the ventral subiculum in stress integration. Behav. Brain Res. 174, 215–224. doi: 10.1016/j.bbr.2006.05.035
Herman, J. P., Ostrander, M. M., Mueller, N. K., and Figueiredo, H. (2005). Limbic system mechanisms of stress regulation: hypothalamo-pituitary-adrenocortical axis. Prog. Neuropsychopharmacol. Biol. Psychiatry 29, 1201–1213. doi: 10.1016/j.pnpbp.2005.08.006
Herman, J. P., Prewitt, C. M., and Cullinan, W. E. (1996). Neuronal circuit regulation of the hypothalamo-pituitary-adrenocortical stress axis. Crit. Rev. Neurobiol. 10, 371–394. doi: 10.1615/critrevneurobiol.v10.i3-4.50
Heshmati, M., and Russo, S. J. (2015). Anhedonia and the brain reward circuitry in depression. Curr. Behav. Neurosci. Reports 2, 146–153. doi: 10.1007/s40473-015-0044-3
Hill, M. N., McLaughlin, R. J., Pan, B., Fitzgerald, M. L., Roberts, C. J., Lee, T. T. Y., et al. (2011). Recruitment of prefrontal cortical endocannabinoid signaling by glucocorticoids contributes to termination of the stress response. J. Neurosci. 31, 10506–10515. doi: 10.1523/JNEUROSCI.0496-11.2011
Hillier, S. G. (2007). Diamonds are forever: the cortisone legacy. J. Endocrinol. 195, 1–6. doi: 10.1677/joe-07-0309
Hindmarch, C. C. T., and Ferguson, A. V. (2016). Physiological roles for the subfornical organ: a dynamic transcriptome shaped by autonomic state. J. Physiol. 594, 1581–1589. doi: 10.1113/jp270726
Hinwood, M., Morandini, J., Day, T. A., and Walker, F. R. (2012). Evidence that micróglia mediate the neurobiological effects of chronic psychological stress on the medial pré-frontal córtex. Cereb. Cortex 22, 1442–1454. doi: 10.1093/cercor/bhr229
Hinwood, M., Tynan, R. J., Charnley, J. L., Beynon, S. B., Day, T. A., and Walker, F. R. (2013). Chronic stress induced remodeling of the prefrontal cortex: structural re-organization of microglia and the inhibitory effect of minocycline. Cereb. Cortex 23, 1784–1797. doi: 10.1093/cercor/bhs151
Hoijemakers, L., Heinen, Y., van Dam, A. M., Lucassen, P. J., and Korosi, A. (2016). Microglial priming and Alzheimer’s disease: a possible role for (Early) imune challenges and epigenetics? Front. Hum. Neurosci. 10:398. doi: 10.3389/fnhum.2016.00398
Holmes, A., and Wellman, C. L. (2009). Stress-induced prefrontal reorganization and executive dysfunction in rodents. Neurosci. Biobehav. Rev. 33, 773–783. doi: 10.1016/j.neubiorev.2008.11.005
Homberg, J. R., Kyzar, E. J., Scattoni, M. L., Norton, W. H., Pittman, J., Gaikwad, S., et al. (2016). Genetic and environmental modulation of neurodevelopmental disorders: translational insights from labs to beds. Brain Res. Bull. 125, 79–91. doi: 10.1016/j.brainresbull.2016.04.015
Huang, L. T. (2014). Early-life stress impacts the developing hippocampus and primes seizure occurrence: cellular, molecular, and epigenetic mechanisms. Front. Mol. Neurosci. 7:8. doi: 10.3389/fnmol.2014.00008
Hueston, C. M., and Deak, T. (2014). The inflamed axis: the interaction between stress, hormones, and the expression of inflammatory-related genes within key structures comprising the hypothalamic-pituitary-adrenal axis. Physiol. Behav. 124, 77–91. doi: 10.1016/j.physbeh.2013.10.035
Hunter, R. G., McCarthy, K. J., Milne, T. A., Pfaff, D. W., and McEwen, B. S. (2009). Regulation of hippocampal H3 histone methylation by acute and chronic stress. Proc. Natl. Acad. Sci. U S A 106, 20912–20917. doi: 10.1073/pnas.0911143106
Inslicht, S. S., Marmar, C. R., Neylan, T. C., Metzler, T. J., Hart, S. L., Otte, C., et al. (2006). Increased cortisol in women with intimate partner violence-related posttraumatic stress disorder. Ann. N Y Acad. Sci. 1071, 428–429. doi: 10.1196/annals.1364.035
Iversen, S., Iversen, L., and Saper, C. B. (2000). Principles of Neural Science. 4th Edn. New York, NY: McGraw-Hill.
Jacobson, L., and Sapolsky, R. (1991). The role of the hippocampus in feedback regulation of the hypothalamic-pituitary-adrenocortical axis. Endocr. Rev. 12, 118–134. doi: 10.1210/edrv-12-2-118
Jagota, A., de la Iglesia, H. O., and Schwartz, W. J. (2000). Morning and evening circadian oscillations in the suprachiasmatic nucleus in vitro . Nat. Neurosci. 3, 372–376. doi: 10.1038/73943
Janak, P. H., and Tye, K. M. (2015). From circuits to behaviour in the amygdala. Nature 517, 284–292. doi: 10.1038/nature14188
Jedema, H. P., and Grace, A. A. (2004). Corticotropin-releasing hormone directly activates noradrenergic neurons of the locus ceruleus recorded in vitro . J. Neurosci. 24, 9703–9713. doi: 10.1523/JNEUROSCI.2830-04.2004
Jeong, Y. H., Park, C. H., Yoo, J., Shin, K. Y., Ahn, S. M., Kim, H. S., et al. (2006). Chronic stress accelerates learning and memory impairments and increases amyloid deposition in APPV717I-CT100 transgenic mice, na Alzheimer’s disease model. FASEB J. 20, 729–731. doi: 10.1096/fj.05-4265fje
Joëls, M. (2009). Stress, the hippocampus, and epilepsy. Epilepsia 50, 586–597. doi: 10.1111/j.1528-1167.2008.01902.x
Joëls, M., and Baram, T. Z. (2009). The neuro-symphony of stress. Nat. Rev. Neurosci. 10, 459–466. doi: 10.1038/nrn2632
Joëls, M., and de Kloet, E. R. (1989). Effects of glucocorticoids and norepinephrine on the excitability in the hippocampus. Science 245, 1502–1505. doi: 10.1126/science.2781292
Joëls, M., Pasricha, N., and Karst, H. (2013). The interplay between rapid and slow corticosteroid actions in brain. Eur. J. Pharmacol. 719, 44–52. doi: 10.1016/j.ejphar.2013.07.015
Joëls, M., Sarabdjitsingh, R. A., and Karst, H. (2012). Unraveling the time domains of corticosteroid hormone influences on brain activity: rapid, slow, and chronic modes. Pharmacol. Rev. 64, 901–938. doi: 10.1124/pr.112.005892
Johnson, L. R., Farb, C., Morrison, J. H., McEwen, B. S., and LeDoux, J. E. (2005). Localization of glucocorticoid receptors at postsynaptic membranes in the lateral amygdala. Neuroscience 136, 289–299. doi: 10.1016/j.neuroscience.2005.06.050
Jones, P. B. (2013). Adult mental health disorders and their age at onset. Br. J. Psychiatry Suppl. 202, s5–s10. doi: 10.1192/bjp.bp.112.119164
Jones, K. R., Myers, B., and Herman, J. P. (2011). Stimulation of the prelimbic cortex differentially modulates neuroendocrine responses to psychogenic and systemic stressors. Physiol. Behav. 104, 266–271. doi: 10.1016/j.physbeh.2011.03.021
Juruena, M. F. (2013). Early-life stress and HPA axis trigger recurrent adulthood depression. Epilepsy Behav. 38, 148–159. doi: 10.1016/j.yebeh.2013.10.020
Juruena, M. F., Cleare, J., and Pariante, M. (2004). The hypothalamic pituitary adrenal axis, glucocorticoid receptor function and relevance to depression. Rev. Bras. Psiquiatr. 26, 189–201. doi: 10.1590/S1516-44462004000300009
Kanner, A. M. (2012). Can neurobiological pathogenic mechanisms of depression facilitate the development of seizure disorders? Lancet Neurol. 11, 1093–1102. doi: 10.1016/S1474-4422(12)70201-6
Kanner, A. M. (2017). Can neurochemical changes of mood disorders explain the increase risk of epilepsy or its worse seizure control? Sleep Med. 42, 2071–2076. doi: 10.1007/s11064-017-2331-8
Kanner, A. M., Scharfman, H., Jette, N., Anagnostou, E., Bernard, C., Camfield, C., et al. (2017). Epilepsy as a network disorder (1): what can we learn from other network disorders such as autistic spectrum disorder and mood disorders? Epilepsy Behav. 77, 106–113. doi: 10.1016/j.yebeh.2017.09.014
Karst, H., Berger, S., Erdmann, G., Schütz, G., and Joëls, M. (2010). Metaplasticity of amygdalar responses to the stress hormone corticosterone. Proc. Natl. Acad. Sci. U S A 107, 14449–14454. doi: 10.1073/pnas.0914381107
Karst, H., Berger, S., Turiault, M., Tronche, F., Schültz, G., and Joëls, M. (2005). Mineralocorticoid receptors are indispensable for nongenomic modulation of hippocampal glutamate transmission by corticosterone. Proc. Natl. Acad. Sci. U S A 102, 19204–19207. doi: 10.1073/pnas.0507572102
Karst, H., de Kloet, E. R., and Joëls, M. (1999). Episodic corticosterone treatment accelerates kindling epileptogenesis and triggers long-term changes in hippocampal CA1 cells, in the fully kindled state. Eur. J. Neurosci. 11, 889–898. doi: 10.1046/j.1460-9568.1999.00495.x
Kawakami, M., Seto, K., Terasawa, E., Yoshida, K., Miyamoto, T., Sekiguchi, M., et al. (1968). Influence of electrical stimulation and lesion in limbic structure upon biosynthesis of adrenocorticoid in the rabbit. Neuroendocrinology 3, 337–348. doi: 10.1159/000121722
Kellner, M., Wiedemann, K., Yassouridis, A., Levengood, R., Guo, L. S., Holsboer, F., et al. (2000). Behavioral and endocrine response to cholecystokinin tetrapeptide in patients with posttraumatic stress disorder. Biol. Psychiatry 47, 107–111. doi: 10.1016/s0006-3223(99)00118-3
Kendall, E. C. (1953). Hormones of the adrenal cortex in health and disease. Proc. Am. Philos. Soc. 97, 8–11.
Kessler, R. C., Sonnega, A., Bromet, E., Hughes, M., and Nelson, C. B. (1995). Posttraumatic stress disorder in the national comorbidity survey. Arch. Gen. Psychiatry 52, 1048–1060. doi: 10.1001/archpsyc.1995.03950240066012
Kim, J. J., Song, E. Y., and Kosten, T. A. (2006). Stress effects in the hippocampus: synaptic plasticity and memory. Stress 9, 1–11. doi: 10.1080/10253890600678004
Kitchener, P., Di Blasi, F., Borrelli, E., and Piazza, P. V. (2004). Differences between brain structures in nuclear translocation and DNA binding of the glucocorticoid receptor during stress and the circadian cycle. Eur. J. Neurosci. 19, 1837–1846. doi: 10.1111/j.1460-9568.2004.03267.x
Koe, A. S., Jones, N. C., Salzberg, M. R., Murphy, A. Z., and State, G. (2009). Early life stress as an influence on limbic epilepsy: a hypothesis whose time has come? Front. Behav. Neurosci. 3:24. doi: 10.3389/neuro.08.024.2009
Koolhaas, J. M., Bartolomucci, A., Buwalda, B., de Boer, S. F., Flügge, G., Korte, S. M., et al. (2011). Stress revisited: a critical evaluation of the stress concept. Neurosci. Biobehav. Rev. 35, 1291–1301. doi: 10.1016/j.neubiorev.2011.02.003
Koolhaas, J. M., de Boer, S. F., Coppens, C. M., and Buwalda, B. (2010). Neuroendocrinology of coping styles: towards understanding the biology of individual variation. Front. Neuroendocrinol. 31, 307–321. doi: 10.1016/j.yfrne.2010.04.001
Krishnan, V., Han, M.-H., Graham, D. L., Berton, O., Renthal, W., Russo, S. J., et al. (2007). Molecular adaptations underlying susceptibility and resistance to social defeat in brain reward regions. Cell 131, 391–404. doi: 10.1016/j.cell.2007.09.018
Kroenke, K., Spitzer, R. L., Williams, J. B. W., Monahan, P. O., and Löwe, B. (2007). Anxiety disorders in primary care: prevalence, impairment, comorbidity, and detection. Ann. Intern. Med. 146, 317–325. doi: 10.7326/0003-4819-146-5-200703060-00004
Krugers, H. J., Arp, J. M., Xiong, H., Kanatsou, S., Lesuis, S. L., Korosi, A., et al. (2017). Early life adversity: lasting consequences for emotional learning. Neurobiol. Stress 6, 14–21. doi: 10.1016/j.ynstr.2016.11.005
Krugers, H., Karst, H., and Joels, M. (2012). Interactions between noradrenaline and corticosteroids in the brain: from electrical activity to cognitive performance. Front. Cell. Neurosci. 6:15. doi: 10.3389/fncel.2012.00015
Kumar, G., Couper, A., Brien, T. J. O., Salzberg, M. R., Jones, N. C., Rees, S. M., et al. (2007). The acceleration of amygdala kindling epileptogenesis by chronic low-dose corticosterone involves both mineralocorticoid and glucocorticoid receptors. Psychoneuroendocrinology 32, 834–842. doi: 10.1016/j.psyneuen.2007.05.011
Kvetnansky, R., Sabban, E. L., and Palkovits, M. (2009). Catecholaminergic systems in stress: structural and molecular genetic approaches. Physiol. Rev. 89, 535–606. doi: 10.1152/physrev.00042.2006
Lammel, S., Ion, D. I., Roeper, J., and Malenka, R. C. (2011). Projection-specific modulation of dopamine neuron synapses by aversive and rewarding stimuli. Neuron 70, 855–862. doi: 10.1016/j.neuron.2011.03.025
Lammel, S., Lim, B. K., and Malenka, R. C. (2014). Reward and aversion in a heterogeneous midbrain dopamine system. Neuropharmacology 76, 351–359. doi: 10.1016/j.neuropharm.2013.03.019
Lammel, S., Lim, B. K., Ran, C., Huang, K. W., Betley, M. J., Tye, K. M., et al. (2012). Input-specific control of reward and aversion in the ventral tegmental area. Nature 491, 212–217. doi: 10.1038/nature11527
Langer, S. Z. (1980). Presynaptic regulation of the release of catecholamines. Pharmacol. Rev. 32, 337–362.
Laviolette, S. R., and Grace, A. A. (2006). Cannabinoids potentiate emotional learning plasticity in neurons of the medial prefrontal cortex through basolateral amygdala inputs. J. Neurosci. 26, 6458–6468. doi: 10.1523/JNEUROSCI.0707-06.2006
LeDoux, J. (2007). The amygdala. Curr. Biol. 17, 868–874. doi: 10.1016/j.cub.2007.08.005
LeDoux, J. (2012). Rethinking the emotional brain. Neuron 73, 653–676. doi: 10.1016/j.neuron.2012.02.004
Lightman, S. L., and Conway-Campbell, B. L. (2010). The crucial role of pulsatile activity of the HPA axis for continuous dynamic equilibration. Nat. Rev. Neurosci. 11, 710–718. doi: 10.1038/nrn2914
Lindauer, R. J. L., Olff, M., van Meijel, E. P. M., Carlier, I. V. E., and Gersons, B. P. R. (2006). Cortisol, learning, memory, and attention in relation to smaller hippocampal volume in police officers with posttraumatic stress disorder. Biol. Psychiatry 59, 171–177. doi: 10.1016/j.biopsych.2005.06.033
Linthorst, A. C., Flachskamm, C., Holsboer, F., and Reul, J. M. (1994). Local administration of recombinant human interleukin-1 α in the rat hippocampus increases serotonergic neurotransmission, hypothalamic-pituitary-adrenocortical axis activity and body temperature. Endocrinology 135, 520–532. doi: 10.1210/en.135.2.520
Lippmann, M., Bress, A., Nemeroff, C. B., Plotsky, P. M., and Monteggia, L. M. (2007). Long-term behavioural and molecular alterations associated with maternal separation in rats. Eur. J. Neurosci. 25, 3091–3098. doi: 10.1111/j.1460-9568.2007.05522.x
Liu, J., Buisman-Pijlman, F., and Hutchinson, M. R. (2014). Toll-like receptor 4: innate immune regulator of neuroimmune and neuroendocrine interactions in stress and major depressive disorder. Front. Neurosci. 8:309. doi: 10.3389/fnins.2014.00309
Loi, M., Koricka, S., Lucassen, P. J., and Joëls, M. (2014). Age- and sex-dependent effects of early life stress on hippocampal neurogenesis. Front. Endocrinol. 5:13. doi: 10.3389/fendo.2014.00013
Lucassen, P. J., Naninck, E. F. G., van Goudoever, J. B., Fitzsimons, C., Joels, M., and Korosi, A. (2013). Perinatal programming of adult hippocampal structure and function; emerging roles of stress, nutrition and epigenetics. Trends Neurosci. 36, 621–631. doi: 10.1016/j.tins.2013.08.002
Lundberg, U. (2005). Stress hormones in health and illness: the roles of work and gender. Psychoneuroendocrinology 30, 1017–1021. doi: 10.1016/j.psyneuen.2005.03.014
Lupien, S. (2015). The history of the science of stress: from Hans Selye to the discovery of anti-inflammatory medication. Sante Ment. Que. 40, 275–286. doi: 10.7202/1033056ar
Lupien, S. J., McEwen, B. S., Gunnar, M. R., and Heim, C. (2009). Effects of stress throughout the lifespan on the brain, behaviour and cognition. Nat. Rev. Neurosci. 10, 434–445. doi: 10.1038/nrn2639
Machado, B. H. (2001). Neurotransmission of the cardiovascular reflexes in the nucleus tractus solitarii of awake rats. Ann. N Y Acad. Sci. 940, 179–196. doi: 10.1111/j.1749-6632.2001.tb03676.x
Maier, S. F., and Seligman, M. E. P. (2016). Learned helplessness at fifty: insights from neuroscience. Psychol. Rev. 123, 349–367. doi: 10.1037/rev0000033
Mantz, J., Thierry, A. M., and Glowinski, J. (1989). Effect of noxious tail pinch on the discharge rate of mesocortical and mesolimbic dopamine neurons: selective activation of the mesocortical system. Brain Res. 476, 377–381. doi: 10.1016/0006-8993(89)91263-8
Martin, E. I., Ressler, K. J., Binder, E., and Nemeroff, C. B. (2009). The neurobiology of anxiety disorders: brain imaging, genetics and psychoneuroendocrinology. Psychiatr. Clin. North Am. 32, 549–575. doi: 10.1016/j.psc.2009.05.004
Mason, J. W., Giller, E. L., Kosten, T. R., Ostroff, R. B., and Podd, L. (1986). Urinary free-cortisol levels in posttraumatic stress disorder patient. J. Nerv. Ment. Dis. 174, 145–149. doi: 10.1097/00005053-198603000-00003
Mason, H. L., Myers, C. S., and Kendall, E. C. (1936). The chemistry of crystalline substances isolated from the suprarrenal gland. J. Biol. Chem. 114, 613–631.
Matsumoto, M., and Hikosaka, O. (2009). Two types of dopamine neuron distinctly convey positive and negative motivational signals. Nature 459, 837–841. doi: 10.1038/nature08028
McCall, J. G., Al-Hasani, R., Siuda, E. R., Hong, D. Y., Norris, A. J., Ford, C. P., et al. (2015). CRH engagement of the locus coeruleus noradrenergic system mediates stress-induced anxiety. Neuron 87, 605–620. doi: 10.1016/j.neuron.2015.07.002
McCann, S. M., and Brobeck, J. R. (1954). Evidence for a role of the supraopticohypophyseal system in regulation of adrenocorticotrophin secretion. Proc. Soc. Exp. Biol. Med. 87, 318–324. doi: 10.3181/00379727-87-21368
Mccorry, L. K. (2007). Physiology of the autonomic nervous system. Am. J. Pharm. Educ. 71:78. doi: 10.5688/aj710478
Mcdonald, A. J. (1998). Cortical pathways to the mammalian amygdala. Prog. Neurobiol. 55, 257–332. doi: 10.1016/s0301-0082(98)00003-3
McEwen, B. S. (2007). Physiology and neurobiology of stress and adaptation: central role of the brain. Physiol. Rev. 87, 873–904. doi: 10.1152/physrev.00041.2006
McEwen, B. S., Gray, J. D., and Nasca, C. (2015). 60 years of neuroendocrinology: redefining neuroendocrinology: stress, sex and cognitive and emotional regulation. J. Endocrinol. 226, T67–T83. doi: 10.1530/joe-15-0121
McEwen, B. S., and Karatsoreos, I. N. (2015). Sleep deprivation and circadian disruption: stress, allostasis and allostatic load. Sleep Med. Clin. 10, 1–10. doi: 10.1016/j.jsmc.2014.11.007
McEwen, B. S., and Magarinos, A. M. (1997). Stress effects on morphology and function of the hippocampus. Ann. N Y Acad. Sci. 821, 271–284. doi: 10.1111/j.1749-6632.1997.tb48286.x
McEwen, B. S., and Stellar, E. (1993). Stress and the individual: mechanism leading to disease. Arch. Intern. Med. 153, 2093–2101. doi: 10.1001/archinte.153.18.2093
McEwen, B. S., Weiss, J. M., and Schwartz, L. S. (1968). Selective retention of corticosterone by limbic structures in rat brain. Nature 220, 911–912. doi: 10.1038/220911a0
McGarry, L. M., and Carter, A. G. (2016). Inhibitory gating of basolateral amygdala inputs to the prefrontal cortex. J. Neurosci. 36, 9391–9406. doi: 10.1523/jneurosci.0874-16.2016
McGowan, P. O., Sasaki, A., D’Alessio, A. C., Dymov, S., Labonté, B., Szyf, M., et al. (2009). Epigenetic regulation of the glucocorticoid receptor in human brain associates with childhood abuse. Nat. Neurosci. 12, 342–348. doi: 10.1038/nn.2270
Mehta, D., and Binder, E. B. (2012). Gene × environment vulnerability factors for PTSD: the HPA-axis. Neuropharmacology 62, 654–662. doi: 10.1016/j.neuropharm.2011.03.009
Milad, M. R., and Quirk, G. J. (2012). Fear extinction as a model for translational neuroscience: ten years of progress. Annu. Rev. Psychol. 63, 129–151. doi: 10.1146/annurev.psych.121208.131631
Mitra, R., and Sapolsky, R. M. (2008). Acute corticosterone treatment is sufficient to induce anxiety and amygdaloid dendritic hypertrophy. Proc. Natl. Acad. Sci. U S A 105, 5573–5578. doi: 10.1073/pnas.0705615105
Mollica, R. F., McInnes, K., Pham, T., Smith Fawzi, M. C., Murphy, E., and Lin, L. (1998). The dose-effect relationships between torture and psychiatric symptoms in vietnamese ex-political detainees and a comparison group. J. Nerv. Ment. Dis. 186, 543–553. doi: 10.1097/00005053-199809000-00005
Morsink, M. C., Joëls, M., Sarabdjitsingh, A. R., Meijer, O. C., de Kloet, E. R., and Datson, N. A. (2006). The dynamic pattern of glucocorticoid receptor-mediated transcriptional responses in neuronal PC12 cells. J. Neurochem. 99, 1282–1298. doi: 10.1111/j.1471-4159.2006.04187.x
Moshe, S., Shilo, M., Chodick, G., Yagev, Y., Blatt, I., Korczyn, A. D., et al. (2008). Occurrence of seizures in association with work-related stress in young male army recruits. Epilepsia 49, 1451–1456. doi: 10.1111/j.1528-1167.2008.01591.x
Muhammad, A., Carroll, C., and Kolb, B. (2012). Stress during development alters dendritic morphology in the nucleus accumbens and prefrontal cortex. Neuroscience 216, 103–109. doi: 10.1016/j.neuroscience.2012.04.041
Musazzi, L., Milanese, M., Farisello, P., Zappettini, S., Tardito, D., Barbiero, V. S., et al. (2010). Acute stress increases depolarization-evoked glutamate release in the rat prefrontal/frontal cortex: the dampening action of antidepressants. PLoS One 5:e8566. doi: 10.1371/journal.pone.0008566
Myers, B., Scheimann, J. R., Franco-Villanueva, A., and Herman, J. P. (2017). Ascending mechanisms of stress integration: implications for brainstem regulation of neuroendocrine and behavioral stress responses. Neurosci. Biobehav. Rev. 74, 366–375. doi: 10.1016/j.neubiorev.2016.05.011
Na, K. S., Chang, H. S., Won, E., Han, K. M., Choi, S., Tae, W. S., et al. (2014). Association between glucocorticoid receptor methylation and hippocampal subfields in major depressive disorder. PLoS One 9:e85425. doi: 10.1371/journal.pone.0085425
Naber, P. A., Lopes da Silva, F. H., and Witter, M. P. (2001). Reciprocal connections between the entorhinal cortex and hippocampal fields CA1 and the subiculum are in register with the projections from CA1 to the subiculum. Hippocampus 11, 99–104. doi: 10.1002/hipo.1028
Nahar, J., Rainville, J. R., Dohanich, G. P., and Tasker, J. G. (2016). Further evidence for a membrane receptor that binds glucocorticoids in the rodent hypothalamus. Steroids 114, 33–40. doi: 10.1016/j.steroids.2016.05.013
Nakken, K. O., Solaas, M. H., Kjeldsen, M. J., Friis, M. L., Pellock, J. M., and Corey, L. A. (2005). Which seizure-precipitating factors do patients with epilepsy most frequently report? Epilepsy Behav. 6, 85–89. doi: 10.1016/j.yebeh.2004.11.003
Neeck, G. (2002). Fifty years of experience with cortisone therapy in the study and treatment of rheumatoid arthritis. Ann. N Y Acad. Sci. 966, 28–38. doi: 10.1111/j.1749-6632.2002.tb04199.x
Nemeroff, C. B. (2016). Paradise lost: the neurobiological and clinical consequences of child abuse and neglect. Neuron 89, 892–909. doi: 10.1016/j.neuron.2016.01.019
Nemeroff, C. B., Bremner, J. D., Foa, E. B., Mayberg, H. S., North, C. S., and Stein, M. B. (2006). Posttraumatic stress disorder: a state-of-the-science review. J. Psychiatr. Res. 40, 1–21. doi: 10.1016/j.jpsychires.2005.07.005
Nestler, E. J. (2014). Epigenetic mechanisms of depression. JAMA Psychiatry 71, 454–456. doi: 10.1001/jamapsychiatry.2013.4291
Nogueira, M. H., Yasuda, C. L., Coan, A. C., Kanner, A. M., and Cendes, F. (2017). Concurrent mood and anxiety disorders are associated with pharmacoresistant seizures in patients with MTLE. Epilepsia 58, 1268–1276. doi: 10.1111/epi.13781
Noh, S. J., Kang, D.-W., Yoo, S. B., Lee, J. Y., Kim, J. Y., Kim, B.-T., et al. (2012). Stress-responsive hypothalamic-nucleus accumbens regulation may vary depending on stressors. Indian J. Exp. Biol. 50, 447–454.
O’Connor, K. A., Johnson, J. D., Hansen, M. K., Wieseler Frank, J. L., Maksimova, E., Watkins, L. R., et al. (2003). Peripheral and central proinflammatory cytokine response to a severe acute stressor. Brain Res. 991, 123–132. doi: 10.1016/j.brainres.2003.08.006
Olijslagers, J. E., de Kloet, E. R., Elgersma, Y., Van Woerden, G. M., Joëls, M., and Karst, H. (2008). Rapid changes in hippocampal CA1 pyramidal cell function via pre- as well as postsynaptic membrane mineralocorticoid receptors. Eur. J. Neurosci. 27, 2542–2550. doi: 10.1111/j.1460-9568.2008.06220.x
Otte, C., Lenoci, M., Metzler, T., Yehuda, R., Marmar, C. R., and Neylan, T. C. (2007). Effects of metyrapone on hypothalamic-pituitary-adrenal axis and sleep in women with post-traumatic stress disorder. Biol. Psychiatry 61, 952–956. doi: 10.1016/j.biopsych.2006.08.018
Palma-Gudiel, H., Córdova-Palomera, A., Leza, J. C., and Fañanás, L. (2015). Glucocorticoid receptor gene (NR3C1) methylation processes as mediators of early adversity in stress-related disorders causality: a critical review. Neurosci. Biobehav. Rev. 55, 520–535. doi: 10.1016/j.neubiorev.2015.05.016
Pavlides, C., Ogawa, S., Kimura, A., and McEwen, B. S. (1996). Role of adrenal steroid mineralocorticoid and glucocorticoid receptors in long-term potentiation in the CA1 field of hippocampal slices. Brain Res. 738, 229–235. doi: 10.1016/s0006-8993(96)00776-7
Pavlides, C., Watanabe, Y., and McEwen, B. S. (1993). Effects of glucocorticoids on hippocampal long-term potentiation. Hippocampus 3, 183–192. doi: 10.1002/hipo.450030210
Pelletier, J. G., Likhtik, E., Filali, M., and Paré, D. (2005). Lasting increases in basolateral amygdala activity after emotional arousal: implications for facilitated consolidation of emotional memories. Learn. Mem. 12, 96–102. doi: 10.1101/lm.88605
Peña, C. J., Kronman, H. G., Walker, D. M., Cates, H. M., Bagot, R. C., Purushothaman, I., et al. (2017). Early life stress confers lifelong stress susceptibility in mice via ventral tegmental area OTX2. Science 356, 1185–1188. doi: 10.1126/science.aan4491
Pezzone, M. A., Lee, W. S., Hoffman, G. E., Pezzone, K. M., and Rabin, B. S. (1993). Activation of brainstem catecholaminergic neurons by conditioned and unconditioned aversive stimuli as revealed by c-Fos immunoreactivity. Brain Res. 608, 310–318. doi: 10.1016/0006-8993(93)91472-5
Prewitt, C. M. F., and Herman, J. P. (1998). Anatomical interactions between the central amygdaloid nucleus and the hypothalamic paraventricular nucleus of the rat: a dual tract-tracing analysis. J. Chem. Neuroanat. 15, 173–185. doi: 10.1016/s0891-0618(98)00045-3
Pu, Z., Krugers, H. J., and Joëls, M. (2007). Corticosterone time-dependently modulates α-adrenergic effects on long-term potentiation in the hippocampal dentate gyrus. Learn. Mem. 14, 359–367. doi: 10.1101/lm.527207
Pynoos, R. S., Goenjian, A., Tashjian, M., Karakashian, M., Manjikian, R., Manoukian, G., et al. (1993). Post-traumatic stress reactions in children after the 1988 Armenian earthquake. Br. J. Psychiatry 163, 239–247. doi: 10.1192/bjp.163.2.239
Radley, J. J. (2012). Toward a limbic cortical inhibitory network: implications for hypothalamic-pituitary-adrenal responses following chronic stress. Front. Behav. Neurosci. 6:7. doi: 10.3389/fnbeh.2012.00007
Radley, J. J., Arias, C. M., and Sawchenko, P. E. (2006). Regional differentiation of the medial prefrontal cortex in regulating adaptive responses to acute emotional stress. J. Neurosci. 26, 12967–12976. doi: 10.1523/jneurosci.4297-06.2006
Radley, J. J., Morilak, D., Viau, V., and Campeau, S. (2015). Chronic stress and brain plasticity: mechanisms underlying adaptive and maladaptive changes and implications for stress-related CNS disorders. Neurosci. Biobehav. Rev. 58, 79–91. doi: 10.1016/j.neubiorev.2015.06.018
Rayner, G. (2017). The contribution of cognitive networks to depression in epilepsy. Epilepsy Curr. 17, 78–83. doi: 10.5698/1535-7511.17.2.78
Reppert, S. M., and Weaver, D. R. (2002). Coordination of circadian timing in mammals. Nature 418, 935–941. doi: 10.1038/nature00965
Reul, J. M., and de Kloet, E. R. (1985). Two receptor systems for corticosterone in rat brain: microdistribution and differential occupation. Endocrinology 117, 2505–2511. doi: 10.1210/endo-117-6-2505
Reul, J. M., and de Kloet, E. R. (1986). Anatomical resolution of two types of corticosterone receptor sites in rat brain with in vitro autoradiography and computerized image analysis. J. Steroid Biochem. 24, 269–272. doi: 10.1016/0022-4731(86)90063-4
Reyes, B. A. S., Valentino, R. J., Xu, G., and Van Bockstaele, E. J. (2005). Hypothalamic projections to locus coeruleus neurons in rat brain. Eur. J. Neurosci. 22, 93–106. doi: 10.1111/j.1460-9568.2005.04197.x
Reyes, B. A. S., Zitnik, G., Foster, C., Van Bockstaele, E. J., and Valentino, R. J. (2015). Social stress engages neurochemically-distinct afferents to the rat locus coeruleus depending on coping strategy. eNeuro 2:ENEURO.0042-15.2015. doi: 10.1523/eneuro.0042-15.2015
Ridderinkhof, K. R., Ullsperger, M., Crone, E. A., and Nieuwenhuis, S. (2004). The role of the medial frontal cortex in cognitive control. Science 306, 443–447. doi: 10.1126/science.1100301
Ritter, S., Watts, A. G., Dinh, T. T., Sanchez-Watts, G., and Pedrow, C. (2003). Immunotoxin lesion of hypothalamically projecting norepinephrine and epinephrine neurons differentially affects circadian and stressor-stimulated corticosterone secretion. Endocrinology 144, 1357–1367. doi: 10.1210/en.2002-221076
Rohleder, N., and Karl, A. (2006). Role of endocrine and inflammatory alterations in comorbid somatic diseases of post-traumatic stress disorder. Minerva Endocrinol. 31, 273–288.
Roozendaal, B., Hernandez, A., Cabrera, S. M., Hagewoud, R., Malvaez, M., Stefanko, D. P., et al. (2010). Membrane-associated glucocorticoid activity is necessary for modulation of long-term memory via chromatin modification. J. Neurosci. 30, 5037–5046. doi: 10.1523/JNEUROSCI.5717-09.2010
Roozendaal, B., McEwen, B. S., and Chattarji, S. (2009). Stress, memory and the amygdala. Nat. Rev. Neurosci. 10, 423–433. doi: 10.1038/nrn2651
Ruiz, J. E., Barbosa Neto, J., Schoedl, A. F., and Mello, M. F. (2007). Psiconeuroendocrinologia do transtorno de estresse pós-traumático. Rev. Bras. Psiquiatr. 29, s7–s12. doi: 10.1590/s1516-44462007000500003
Russo, S. J., and Nestler, E. J. (2013). The brain reward circuitry in mood disorders. Nat. Rev. Neurosci. 14, 609–625. doi: 10.1038/nrn3381
Saito, H., Kaba, H., Sato, T., Honmura, A., Kawakami, T., Seto, K., et al. (1989). Influence of electrical stimulation of the limbic structure on adrenocortical steroidogenesis in hypophysctomized rats. Exp. Clin. Endrocinol. 94, 387–390. doi: 10.1055/s-0029-1210926
Salzberg, M., Kumar, G., Supit, L., Jones, N. C., Morris, M. J., Rees, S., et al. (2007). Early postnatal stress confers enduring vulnerability to limbic epileptogenesis. Epilepsia 48, 2079–2085. doi: 10.1111/j.1528-1167.2007.01246.x
Sánchez, M. M., Ladd, C. O., and Plotsky, P. M. (2001). Early adverse experience as a developmental risk factor for later psychopathology: evidence from rodent and primate models. Dev. Psychopathol. 13, 419–449. doi: 10.1017/s0954579401003029
Saper, C. B., and Stornetta, R. L. (2015). “Central Autonomic System,” in The Rat Nervous System , ed. G. Paxinos, 4th Edn. (Amsterdam: Elsevier), 629–673.
Sapolsky, R. M. (2000a). Glucocorticoids and hippocampal atrophy in neuropsychiatric disorders. Arch. Gen. Psychiatry 57, 925–935. doi: 10.1001/archpsyc.57.10.925
Sapolsky, R. M. (2000b). The possibility of neurotoxicity in the hippocampus in major depression: a primer on neuron death. Biol. Psychiatry 48, 755–765. doi: 10.1016/s0006-3223(00)00971-9
Sapolsky, R. M., Krey, L. C., and McEwen, B. S. (1985). Prolonged glucocorticoid exposure reduces hippocampal neuron number: implications for aging. J. Neurosci. 5, 1222–1227. doi: 10.1523/jneurosci.05-05-01222.1985
Sapolsky, R. M., and Meaney, M. J. (1986). Maturation of the adrenocortical stress response: neuroendocrine control mechanisms and the stress hyporesponsive period. Brain Res. 396, 64–76. doi: 10.1016/0165-0173(86)90010-x
Sapolsky, R., and Pulsinelli, W. (1985). Glucocorticoids potentiate ischemic injury to neurons: therapeutic implications. Science 229, 1397–1400. doi: 10.1126/science.4035356
Sapolsky, R. M., Romero, L. M., and Munck, A. U. (2000). How do glucocorticoids influence stress responses? Integrating permissive, suppressive, stimulatory and preparative actions. Endocr. Rev. 21, 55–89. doi: 10.1210/edrv.21.1.0389
Saveanu, R. V., and Nemeroff, C. B. (2012). Etiology of depression: genetic and environmental factors. Psychiatr. Clin. North Am. 35, 51–71. doi: 10.1016/j.psc.2011.12.001
Sawchenko, P. E., Brown, E. R., Chan, R. K., Ericsson, A., Li, H. Y., Roland, B. L., et al. (1996). The paraventricular nucleus of the hypothalamus and the functional neuroanatomy of visceromotor responses to stress. Prog. Brain Res. 107, 201–222. doi: 10.1016/s0079-6123(08)61866-x
Sawchenko, P. E., Imaki, T., and Vale, W. (1992). Co-localization of neuroactive substances in the endocrine hypothalamus. Ciba Found. Symp. 168, 16–30.
Schnyder, U., Moergeli, H., Klaghofer, R., and Buddeberg, C. (2001). Incidence and prediction of posttraumatic stress disorder symptoms in severely injured accident victims. Am. J. Psychiatry 158, 594–599. doi: 10.1176/appi.ajp.158.4.594
Schulkin, J. (2003). Allostasis: a neural behavioral perspective. Horm. Behav. 43, 21–27. doi: 10.1016/s0018-506x(02)00035-1
Schulkin, J., Gold, P. W., and McEwen, B. S. (1998). Induction of corticotropin-releasing hormone gene expression by glucocorticoids: implication for understanding the states of fear and anxiety and allostatic load. Psychoneuroendocrinology 23, 219–243. doi: 10.1016/s0306-4530(97)00099-1
Schulkin, J., McEwen, B. S., and Gold, P. W. (1994). Allostasis, amygdala and anticipatory angst. Neurosci. Biobehav. Rev. 18, 385–396. doi: 10.1016/0149-7634(94)90051-5
Seggie, J. (1987). Differential responsivity of corticosterone and prolactin to stress following lesions of the septum or amygdala: implications for psychoneuroendocrinology. Prog. Neuropsychopharmacol. Biol. Psychiatry 11, 315–324. doi: 10.1016/0278-5846(87)90076-5
Selye, H. (1936). A syndrome produced by diverse nocuous agents. Nature 138:32. doi: 10.1038/138032a0
Selye, H. (1950). Stress and the general adaptation syndrome. Br. Med. J. 1, 1383–1392.
Selye, H. (1976). The stress concept. Can. Med. Assoc. J. 115:718.
Senst, L., and Bains, J. (2014). Neuromodulators, stress and plasticity: a role for endocannabinoid signalling. J. Exp. Biol. 217, 102–108. doi: 10.1242/jeb.089730
Shore, J. H., Tatum, E. L., and Vollmer, W. M. (1986). Psychiatric reactions to disaster: the Mount St. Helens experience. Am. J. Psychiatry 143, 590–595. doi: 10.1176/ajp.143.5.590
Simpson, J. B., and Routtenberg, A. (1975). Subfornical organ lesions reduce intravenous angiotensin-induced drinking. Brain Res. 88, 154–161. doi: 10.1016/0006-8993(75)90965-8
Skoluda, N., Strahler, J., Schlotz, W., Niederberger, L., Marques, S., Fischer, S., et al. (2015). Intra-individual psychological and physiological responses to acute laboratory stressors of different intensity. Psychoneuroendocrinology 51, 227–236. doi: 10.1016/j.psyneuen.2014.10.002
Smart, C., Strathdee, G., Watson, S., Murgatroyd, C., and McAllister-Williams, R. H. (2015). Early life trauma, depression and the glucocorticoid receptor gene-an epigenetic perspective. Psychol. Med. 45, 3393–3410. doi: 10.1017/s0033291715001555
Smith, M. A., Davidson, J., Ritchie, J. C., Kudler, H., Lipper, S., Chappell, P., et al. (1989). The corticotropin-releasing hormone test in patients with posttraumatic stress disorder. Biol. Psychiatry 26, 349–355. doi: 10.1016/0006-3223(89)90050-4
Smith, S. M., and Vale, W. W. (2006). The role of the hypothalamic-pituitary-adrenal axis in neuroendocrine responses to stress. Dialogues Clin. Neurosci. 8, 383–395.
Smotherman, W. P., Burt, G., Kimble, D. P., Strickrod, G., BreMiller, R., and Levine, S. (1981). Behavioral and corticosterone effects in conditioned taste aversion following hippocampal lesions. Physiol. Behav. 27, 569–574. doi: 10.1016/0031-9384(81)90224-9
Snow, B. R., Stellman, J. M., Stellman, S. D., and Sommer, J. F. Jr. (1988). Post-traumatic stress disorder among american legionnaires in relation to combat experience in vietnam: associated and contributing factors. Environ. Res. 47, 175–192. doi: 10.1016/s0013-9351(88)80040-9
Snyder, K., Wang, W. W., Han, R., McFadden, K., and Valentino, R. J. (2012). Corticotropin-releasing factor in the norepinephrine nucleus, locus coeruleus, facilitates behavioral flexibility. Neuropsychopharmacology 37, 520–530. doi: 10.1038/npp.2011.218
Southwick, S. M., Bremner, J. D., Rasmusson, A., Morgan, C. A. III., Arnsten, A., and Charney, D. S. (1999). Role of norepinephrine in the pathophysiology and treatment of posttraumatic stress disorder. Biol. Psychiatry 46, 1192–1204. doi: 10.1016/s0006-3223(99)00219-x
Spector, S., Cull, C., and Goldstein, L. H. (2000). Seizure precipitants and perceived self-control of seizures in adults with poorly-controlled epilepsy. Epilepsy Res. 38, 207–216. doi: 10.1016/s0920-1211(99)00093-5
Sperling, M. R., Schilling, C. A., Glosser, D., Tracy, J. I., and Asadi-Pooya, A. A. (2008). Self-perception of seizure precipitants and their relation to anxiety level, depression, and health locus of control in epilepsy. Seizure 17, 302–307. doi: 10.1016/j.seizure.2007.09.003
Spiga, F., Zavala, E., Walker, J. J., Zhao, Z., Terry, J. R., and Lightman, S. L. (2017). Dynamic responses of the adrenal steroidogenic regulatory network. Proc. Natl. Acad. Sci. U S A 114, E6466–E6474. doi: 10.1073/pnas.1703779114
Steptoe, A., Hamer, M., and Chida, Y. (2007). The effects of acute psychological stress on circulating inflammatory factors in humans: a review and meta-analysis. Brain Behav. Immun. 21, 901–912. doi: 10.1016/j.bbi.2007.03.011
Sterling, P., and Eyer, J. (1988). Allostasis A New Paradigm to Explain Arousal Pathology. Handbook of Life Stress Cognition and Health. Oxford: Oxford University Press.
Stevenson, M., Segui-Gomez, M., Lescohier, I., Di Scala, C., and McDonald-Smith, G. (2001). An overview of the injury severity score and the new injury severity score. InJ. Prev. 7, 10–13. doi: 10.1136/ip.7.1.10
Stewart, M. G., Davies, H. A., Sandi, C., Kraev, I. V., Rogachevsky, V. V., Peddie, C. J., et al. (2005). Stress suppresses and learning induces plasticity in CA3 of rat hippocampus: a three-dimensional ultrastructural study of thorny excrescences and their postsynaptic densities. Neuroscience 131, 43–54. doi: 10.1016/j.neuroscience.2004.10.031
Stuber, G. D., Sparta, D. R., Stamatakis, A. M., van Leeuwen, W. A., Hardjoprajitno, J. E., Cho, S., et al. (2011). Excitatory transmission from the amygdala to nucleus accumbens facilitates reward seeking. Nature 475, 377–380. doi: 10.1038/nature10194
Sullivan, R. M., and Gratton, A. (1999). Lateralized effects of medial prefrontal cortex lesions on neuroendocrine and autonomic stress responses in rats. J. Neurosci. 19, 2834–2840. doi: 10.1523/JNEUROSCI.19-07-02834.1999
Sullivan, R. M., and Gratton, A. (2002). Behavioral effects of excitotoxic lesions of ventral medial prefrontal cortex in the rat are hemisphere-dependent. Brain Res. 927, 69–79. doi: 10.1016/s0006-8993(01)03328-5
Sun, S., Yang, S., Mao, Y., Jia, X., and Zhang, Z. (2015). Reduced cholesterol is associated with the depressive-like behavior in rats through modulation of the brain 5-HT1 A receptor. Lipids Health Dis. 14:22. doi: 10.1186/s12944-015-0020-7
Sutker, P. B., Allain, A. N., and Winstead, D. K. (1993). Psychopathology and psychiatric diagnoses of World War II Pacific theater prisoner of war survivors and combat veterans. Am. J. Psychiatry 150, 240–245. doi: 10.1176/ajp.150.2.240
Swanson, L. W., and Hartman, B. K. (1975). The central adrenergic system. An immunofluorescence study of the location of cell bodies and their efferent connections in the rat utilizing dopamine-α-hydroxylase as a marker. J. Comp. Neurol. 163, 467–505. doi: 10.1002/cne.901630406
Swartz, J. R., Knodt, A. R., Radtke, S. R., and Hariri, A. R. (2015). A neural biomarker of psychological vulnerability to future life stress. Neuron 85, 505–511. doi: 10.1016/j.neuron.2014.12.055
Swinkels, W. A. M., Engelsman, M., Kasteleijn-Nolst Trenite, D. G. A., Baal, M. G., de Haan, G. J., and Oosting, J. (1998). Influence of an evacuation in february 1995 in the Netherlands on the seizure frequency in patients with epilepsy: a controlled study. Epilepsia 39, 1203–1207. doi: 10.1111/j.1528-1157.1998.tb01312.x
Takahashi, A., Flanigan, M. E., McEwen, B. S., and Russo, S. J. (2018). Aggression, social stress, and the immune system in humans and animal models. Front. Behav. Neurosci. 12:56. doi: 10.3389/fnbeh.2018.00056
Tan, K. R., Yvon, C., Turiault, M., Mirzabekov, J. J., Doehner, J., Labouèbe, G., et al. (2012). GABA neurons of the VTA drive conditioned place aversion. Neuron 73, 1173–1183. doi: 10.1016/j.neuron.2012.02.015
Tank, A. W., and Lee Wong, D. (2015). Peripheral and central effects of circulating catecholamines. Compr. Physiol. 5, 1–15. doi: 10.1002/cphy.c140007
Timmermans, W., Xiong, H., Hoogenraad, C. C., and Krugers, H. J. (2013). Stress and excitatory synapses: from health to disease. Neuroscience 248, 626–636. doi: 10.1016/j.neuroscience.2013.05.043
Totola, L. T., Takakura, A. C., Oliveira, J. A., Garcia-Cairasco, N., and Moreira, T. S. (2017). Impaired central respiratory chemoreflex in an experimental genetic model of epilepsy. J. Physiol. 595, 983–999. doi: 10.1113/JP272822
Tsai, H.-C., Zhang, F., Adamantidis, A., Stuber, G. D., Bonci, A., de Lecea, L., et al. (2009). Phasic firing in dopaminergic neurons is sufficient for behavioral conditioning. Science 324, 1080–1084. doi: 10.1126/science.1168878
Tsankova, N. M., Berton, O., Renthal, W., Kumar, A., Neve, R. L., and Nestler, E. J. (2006). Sustained hippocampal chromatin regulation in a mouse model of depression and antidepressant action. Nat. Neurosci. 9, 519–525. doi: 10.1038/nn1659
Tynan, R. J., Naicker, S., Hinwood, M., Nalivaiko, E., Buller, K. M., Pow, D. V., et al. (2010). Chronic stress alters the density and morphology of microglia in a subset of stress-responsive brain regions. Brain Behav. Immun. 24, 1058–1068. doi: 10.1016/j.bbi.2010.02.001
Ulrich-Lai, Y. M., and Herman, J. P. (2009). Neural regulation of endocrine and autonomic stress responses. Nat. Rev. Neurosci. 10, 397–409. doi: 10.1038/nrn2647
Umeoka, E. H., Garcia, S. B., Antunes-Rodrigues, J., Elias, L. L., and Garcia-Cairasco, N. (2011). Functional characterization of the hypothalamic-pituitary-adrenal axis of the Wistar Audiogenic Rat (WAR) strain. Brain Res. 1381, 141–147. doi: 10.1016/j.brainres.2011.01.042
Vale, W., Rivier, C., Yang, L., Minick, S., and Guillemin, R. (1978). Effects of purified hypothalamic corticotropin-releasing factor and other substances on the secretion of adrenocorticotropin and α-endorphin-like immunoactivities in vitro . Endocrinology 103, 1910–1915. doi: 10.1210/endo-103-5-1910
Vale, W., Spiess, J., Rivier, C., and Rivier, J. (1981). Characterization of a 41-residue ovine hypothalamic peptide that stimulates secretion of corticotropin and α-endorphin. Science 213, 1394–1397. doi: 10.1126/science.6267699
Valentino, R. J., Miselis, R. R., and Pavcovich, L. A. (1999). Pontine regulation of pelvic viscera: pharmacological target for pelvic visceral dysfunctions. Trends Pharmacol. Sci. 20, 253–260. doi: 10.1016/s0165-6147(99)01332-2
Valentino, R. J., Page, M. E., and Curtis, A. L. (1991). Activation of noradrenergic locus coeruleus neurons by hemodynamic stress is due to local release of corticotropin-releasing factor. Brain Res. 555, 25–34. doi: 10.1016/0006-8993(91)90855-p
Valentino, R. J., Page, M., Van Bockstaele, E., and Aston-Jones, G. (1992). Corticotropin-releasing factor innervation of the locus coeruleus region: distribution of fibers and sources of input. Neuroscience 48, 689–705. doi: 10.1016/0306-4522(92)90412-u
Valentino, R. J., Reyes, B., Van Bockstaele, E., and Bangasser, D. (2012). Molecular and cellular sex differences at the intersection of stress and arousal. Neuropharmacology 62, 13–20. doi: 10.1016/j.neuropharm.2011.06.004
Valentino, R. J., Sheng, C., Yan, Z., and Aston-Jones, G. (1996). Evidence for divergent projections to the brain noradrenergic system and the spinal parasympathetic system from Barrington’s nucleus. Brain Res. 732, 1–15. doi: 10.1016/0006-8993(96)00482-9
Valentino, R. J., and Van Bockstaele, E. (2008). Convergent regulation of locus coeruleus activity as an adaptive response to stress. Eur. J. Pharmacol. 583, 194–203. doi: 10.1016/j.ejphar.2007.11.062
Van Bockstaele, E. J., Colago, E. E., and Valentino, R. J. (1998). Amygdaloid corticotropin-releasing factor targets locus coeruleus dendrites: substrate for the co-ordination of emotional and cognitive limbs of the stress response. J. Neuroendocrinol. 10, 743–757. doi: 10.1046/j.1365-2826.1998.00254.x
Van Bockstaele, E. J., Peoples, J., and Valentino, R. J. (1999). Anatomic basis for differential regulation of the rostrolateral peri-locus coeruleus region by limbic afferents. Biol. Psychiatry 46, 1352–1363. doi: 10.1016/s0006-3223(99)00213-9
van Campen, J. S., Jansen, F. E., de Graan, P. N., Braun, K. P., and Joëls, M. (2014). Early life stress in epilepsy: a seizure precipitant and risk fator for epileptogenesis. Epilepsy Behav. 38, 160–171. doi: 10.1016/j.yebeh.2013.09.029
van Campen, J. S., Jansen, F. E., Steinbusch, L. C., Joëls, M., and Braun, K. P. J. (2012). Stress sensitivity of childhood epilepsy is related to experienced negative life events. Epilepsia 53, 1554–1562. doi: 10.1111/j.1528-1167.2012.03566.x
Verkuyl, J. M., Karst, H., and Joëls, M. (2005). GABAergic transmission in the rat paraventricular nucleus of the hypothalamus is suppressed by corticosterone and stress. Eur. J. Neurosci. 21, 113–121. doi: 10.1111/j.1460-9568.2004.03846.x
Vialou, V., Bagot, R. C., Cahill, M. E., Ferguson, D., Robison, A. J., Dietz, D. M., et al. (2014). Prefrontal cortical circuit for depression- and anxiety-related behaviors mediated by cholecystokinin: role of FosB. J. Neurosci. 34, 3878–3887. doi: 10.1523/JNEUROSCI.1787-13.2014
Vitkovic, L., Konsman, J. P., Bockaert, J., Dantzer, R., Homburger, V., and Jacque, C. (2000). Cytokine signals propagate through the brain. Mol. Psychiatry 5, 604–615. doi: 10.1038/sj.mp.4000813
Vyas, A., Mitra, R., Shankaranarayana Rao, B. S., and Chattarji, S. (2002). Chronic stress induces contrasting patterns of dendritic remodeling in hippocampal and amygdaloid neurons. J. Neurosci. 22, 6810–6818. doi: 10.1523/JNEUROSCI.22-15-06810.2002
Vyas, A., Pillai, A. G., and Chattarji, S. (2004). Recovery after chronic stress fails to reverse amygdaloid neuronal hypertrophy and enhanced anxiety-like behavior. Neuroscience 128, 667–673. doi: 10.1016/j.neuroscience.2004.07.013
Walsh, R. (2011). Lifestyle and mental health. Am. Psychol. 66, 579–592. doi: 10.1037/a0021769
Wang, C. C., and Wang, S. J. (2009). Modulation of presynaptic glucocorticoid receptors on glutamate release from rat hippocampal nerve terminals. Synapse 63, 745–751. doi: 10.1002/syn.20654
Weaver, I. C. G., Cervoni, N., Champagne, F. A., D’Alessio, A. C., Sharma, S., Seckl, J. R., et al. (2004). Epigenetic programming by maternal behavior. Nat. Neurosci. 7, 847–854. doi: 10.1038/nn1276
Wei, J., Zhong, P., Qin, L., Tan, T., and Yan, Z. (2017). Chemicogenetic restoration of the prefrontal cortex to amygdala pathway ameliorates stress-induced deficits. Cereb. Cortex 28, 1980–1990. doi: 10.1093/cercor/bhx104
Weikum, E. R., Knuesel, M. T., Ortlund, E. A., and Yamamoto, K. R. (2017). Glucocorticoid receptor control of transcription: precision and plasticity via allostery. Nat. Rev. Mol. Cell Biol. 18, 159–174. doi: 10.1038/nrm.2016.152
Welsh, D. K., Logothetis, D. E., Meister, M., and Reppert, S. M. (1995). Individual neurons dissociated from rat suprachiasmatic nucleus express independently phased circadian firing rhythms. Neuron 14, 697–706. doi: 10.1016/0896-6273(95)90214-7
Wessa, M., Rohleder, N., Kirschbaum, C., and Flor, H. (2006). Altered cortisol awakening response in posttraumatic stress disorder. Psychoneuroendocrinology 31, 209–215. doi: 10.1016/j.psyneuen.2005.06.010
Widom, C. S., DuMont, K., and Czaja, S. J. (2007). A prospective investigation of major depressive disorder and comorbidity in abused and neglected children grown up. Arch. Gen. Psychiatry 64, 49–56. doi: 10.1001/archpsyc.64.1.49
Wilker, S., and Kolassa, I. T. (2012). Genetic influences on posttraumatic stress disorder (PTSD): inspirations from a memory-centered approach. Psychiatr. Danub. 24, 278–279.
Wilkinson, M. B., Xiao, G., Kumar, A., LaPlant, Q., Renthal, W., Sikder, D., et al. (2009). Imipramine treatment and resiliency exhibit similar chromatin regulation in the mouse nucleus accumbens in depression models. J. Neurosci. 29, 7820–7832. doi: 10.1523/JNEUROSCI.0932-09.2009
Willner, P., Scheel-krüger, J., and Belzung, C. (2013). The neurobiology of depression and antidepressant action. Neurosci. Biobehav. Rev. 37, 2331–2371. doi: 10.1016/j.neubiorev.2012.12.007
Witter, M. P. (2007). The perforant path: projections from the entorhinal cortex to the dentate gyrus. Prog. Brain Res. 163, 43–61. doi: 10.1016/s0079-6123(07)63003-9
Wohleb, E. S., Hanke, M. L., Corona, A. W., Powell, N. D., Stiner, L. M., Bailey, M. T., et al. (2011). β-Adrenergic receptor antagonism prevents anxiety-like behavior and microglial reactivity induced by repeated social defeat. J. Neurosci. 31, 6277–6288. doi: 10.1523/JNEUROSCI.0450-11.2011
Wohleb, E. S., McKim, D. B., Sheridan, J. F., and Godbout, J. P. (2015). Monocyte trafficking to the brain with stress and inflammation: a novel axis of immune-to-brain communication that influences mood and behavior. Front. Neurosci. 8:447. doi: 10.3389/fnins.2014.00447
Wong, M. L., Kling, M. A., Munson, P. J., Listwak, S., Licinio, J., Prolo, P., et al. (2000). Pronounced and sustained central hypernoradrenergic function in major depression with melancholic features: relation to hypercortisolism and corticotropin-releasing hormone. Proc. Natl. Acad. Sci. U S A 97, 325–330. doi: 10.1073/pnas.97.1.325
Wood, G. E., Norris, E. H., Waters, E., Stoldt, J. T., and McEwen, B. S. (2008). Chronic immobilization stress alters aspects of emotionality and associative learning in the rat. Behav. Neurosci. 122, 282–292. doi: 10.1037/0735-7044.122.2.282
Wood, S. K., and Valentino, R. J. (2016). The brain norepinephrine system, stress and cardiovascular vulnerability. Neurosci. Biobehav. Rev. 74, 393–400. doi: 10.1016/j.neubiorev.2016.04.018
Xiong, W., Wei, H., Xiang, X., Cao, J., Dong, Z., Wang, Y., et al. (2004). The effect of acute stress on LTP and LTD induction in the hippocampal CA1 region of anesthetized rats at three different ages. Brain Res. 1005, 187–192. doi: 10.1016/j.brainres.2004.01.051
Xiong, F., and Zhang, L. (2013). Role of the hypothalamic-pituitary-adrenal axis in developmental programming of health and disease. Front. Neuroendocrinol. 34, 27–46. doi: 10.1016/j.yfrne.2012.11.002
Yang, J. C., Park, K., Eun, S. J., Lee, M. S., Yoon, J. S., Shin, I. S., et al. (2008). Assessment of cerebrocortical areas associated with sexual arousal in depressive women using functional MR imaging. J. Sex. Med. 5, 602–609. doi: 10.1111/j.1743-6109.2007.00737.x
Yehuda, R., Boisoneau, D., Mason, J. W., and Giller, E. L. (1993). Glucocorticoid receptor number and cortisol excretion in mood, anxiety, and psychotic disorders. Biol. Psychiatry 34, 18–25. doi: 10.1016/0006-3223(93)90252-9
Yehuda, R., Golier, J. A., Halligan, S. L., and Harvey, P. D. (2004a). Learning and memory in Holocaust survivors with posttraumatic stress disorder. Biol. Psychiatry 55, 291–295. doi: 10.1016/s0006-3223(03)00641-3
Yehuda, R., Golier, J. A., Halligan, S. L., Meaney, M., and Bierer, L. M. (2004b). The ACTH response to dexamethasone in PTSD. Am. J. Psychiatry 161, 1397–1403. doi: 10.1176/appi.ajp.161.8.1397
Yehuda, R., Hoge, C. W., McFarlane, A. C., Vermetten, E., Lanius, R. A., Nievergelt, C. M., et al. (2015). Post-traumatic stress disorder. Nat. Rev. Dis. Primers 1:15057. doi: 10.1038/nrdp.2015.57
Yehuda, R., Southwick, S. M., Nussbaum, G., Wahby, V., Giller, E. L. Jr., and Mason, J. W. (1990). Low urinary cortisol excretion in patients with posttraumatic stress disorder. J. Nerv. Ment. Dis. 178, 366–369. doi: 10.1097/00005053-199006000-00004
Young, E. A., Abelson, J., and Lightman, S. L. (2004). Cortisol pulsatility and its role in stress regulation and health. Front. Neuroendocrinol. 25, 69–76. doi: 10.1016/j.yfrne.2004.07.001
Yuen, E. Y., Wei, J., Liu, W., Zhong, P., Li, X., and Yan, Z. (2012). Repeated stress causes cognitive impairment by suppressing glutamate receptor expression and function in prefrontal cortex. Neuron 73, 962–977. doi: 10.1016/j.neuron.2011.12.033
Zalachoras, I., Houtman, R., Atucha, E., Devos, R., Tijssen, A. M. I., Hu, P., et al. (2013). Differential targeting of brain stress circuits with a selective glucocorticoid receptor modulator. Proc. Natl. Acad. Sci. U S A 110, 7910–7915. doi: 10.1073/pnas.1219411110
Zhang, D. Y., and Anderson, A. S. (2014). The sympathetic nervous system and heart failure. Cardiol. Clin. 32, 33–45. doi: 10.1016/j.ccl.2013.09.010
Zhang, J., Abdallah, C. G., Chen, Y., Huang, T., Huang, Q., Xu, C., et al. (2013). Behavioral deficits, abnormal corticosterone, and reduced prefrontal metabolites of adolescent rats subject to early life stress. Neurosci. Lett. 545, 132–137. doi: 10.1016/j.neulet.2013.04.035
Zheng, C., and Zhang, T. (2015). Synaptic plasticity-related neural oscillations on hippocampus-prefrontal cortex pathway in depression. Neuroscience 292, 170–180. doi: 10.1016/j.neuroscience.2015.01.071
Zhou, M., Hoogenraad, C. C., Joëls, M., and Krugers, H. J. (2012). Combined β-adrenergic and corticosteroid receptor activation regulates AMPA receptor function in hippocampal neurons. J. Psychopharmacol. 26, 516–524. doi: 10.1177/0269881111424930
Zhu, W., Umegaki, H., Suzuki, Y., Miura, H., and Iguchi, A. (2001). Involvement of the bed nucleus of the stria terminalis in hippocampal cholinergic system-mediated activation of the hypothalamo-pituitary-adrenocortical axis in rats. Brain Res. 916, 101–106. doi: 10.1016/s0006-8993(01)02871-2
Ziegler, D. R., Cass, W. A., and Herman, J. P. (1999). Excitatory influence of the locus coeruleus in hypothalamic-pituitary-adrenocortical axis responses to stress. J. Neuroendocrinol. 11, 361–369. doi: 10.1046/j.1365-2826.1999.00337.x
Zoccal, D. B., Furuya, W. I., Bassi, M., Colombari, D. S. A., and Colombari, E. (2014). The nucleus of the solitary tract and the coordination of respiratory and sympathetic activities. Front. Physiol. 5:238. doi: 10.3389/fphys.2014.00238
Zoladz, P. R., and Diamond, D. M. (2013). Current status on behavioral and biological markers of PTSD: a search for clarity in a conflicting literature. Neurosci. Biobehav. Rev. 37, 860–895. doi: 10.1016/j.neubiorev.2013.03.024
Zoladz, P. R., and Diamond, D. M. (2016). Predator-based psychosocial stress animal model of PTSD: preclinical assessment of traumatic stress at cognitive, hormonal, pharmacological, cardiovascular and epigenetic levels of analysis. Exp. Neurol. 284, 211–219. doi: 10.1016/j.expneurol.2016.06.003
Zorn, J. V., Schür, R. R., Boks, M. P., Kahn, R. S., Joëls, M., and Vinkers, C. H. (2017). Cortisol stress reactivity across psychiatric disorders: a systematic review and meta-analysis. Psychoneuroendocrinology 77, 25–36. doi: 10.1016/j.psyneuen.2016.11.036
Keywords: stress history, stress response, physical and psychological stressors, neuroanatomy, SAM axis, HPA axis, HPA axis time-domain, clinical implications of stress
Citation: Godoy LD, Rossignoli MT, Delfino-Pereira P, Garcia-Cairasco N and Umeoka EHL (2018) A Comprehensive Overview on Stress Neurobiology: Basic Concepts and Clinical Implications. Front. Behav. Neurosci. 12:127. doi: 10.3389/fnbeh.2018.00127
Received: 15 March 2018; Accepted: 06 June 2018; Published: 03 July 2018.
Reviewed by:
Copyright © 2018 Godoy, Rossignoli, Delfino-Pereira, Garcia-Cairasco and Umeoka. This is an open-access article distributed under the terms of the Creative Commons Attribution License (CC BY) . The use, distribution or reproduction in other forums is permitted, provided the original author(s) and the copyright owner(s) are credited and that the original publication in this journal is cited, in accordance with accepted academic practice. No use, distribution or reproduction is permitted which does not comply with these terms.
*Correspondence: Norberto Garcia-Cairasco, [email protected] Eduardo Henrique de Lima Umeoka, [email protected]
† These authors have contributed equally to this work.
Disclaimer: All claims expressed in this article are solely those of the authors and do not necessarily represent those of their affiliated organizations, or those of the publisher, the editors and the reviewers. Any product that may be evaluated in this article or claim that may be made by its manufacturer is not guaranteed or endorsed by the publisher.
- MEMBER LOGIN
- MEDIA RELATIONS
Military Stress
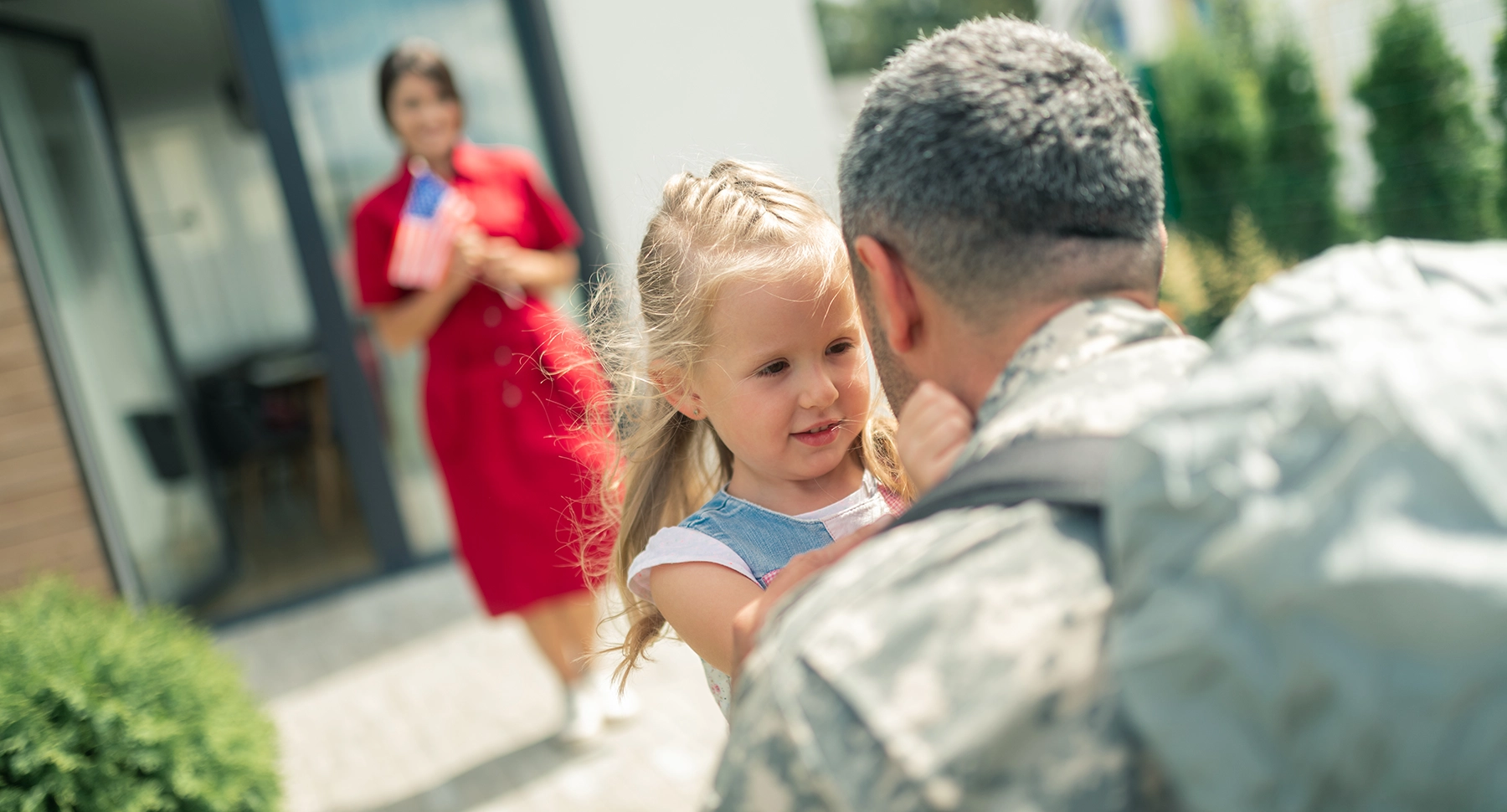
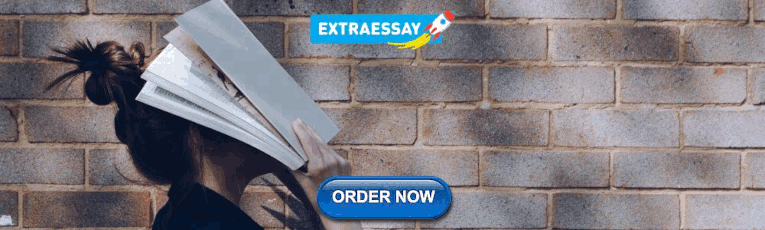
Stress Research
“The difficulty in science is often not so much how to make the discovery but rather to know that one has made it.”
– J.D. Bernal
Causes & Sources of Stress
Living conditions, the political climate, financial insecurity, and work issues are some stressors US adults cite as the cause of their stress. Ineffective communications increase work stress to the point of frustration that workers want to quit. These stressors, unfortunately, are not something people can just ignore. Quitting a job would result in debt and financial instability which, in turn, would be added stressors.
- 35% of workers say their boss is a cause of their workplace stress.
- 80% of US workers experience work stress because of ineffective company communications.
- 39% of North American employees report their workload the main source of the work stress.
- 49% of 18 – 24 year olds who report high levels of stress felt comparing themselves to others is a stressor.
- 71% of US adults with private health insurance say the cost of healthcare causes them stress while 53% with public insurance say the same.
- 54% of Americans want to stay informed about the news but following the news causes them stress.
- 42% of US adults cite personal debt as a source of significant stress.
- 1 in 4 American adults say discrimination is a significant source of stress.
- Mass shootings are a significant source of stress across all races; 84% of Hispanic report this, the highest among the races.
Stress Statistics
Two years after the World Health Organization declared COVID-19 a global pandemic, inflation, money issues and the war in Ukraine have pushed U.S. stress to alarming levels, according to polls conducted for the American Psychological Association.
A late-breaking poll, fielded March 1-3 by The Harris Poll on behalf of APA, revealed striking findings, with more adults rating inflation and issues related to the invasion of Ukraine as stressors than any other issue asked about in the 15-year history of the Stress in AmericaTM poll. This comes on top of money stress at the highest recorded level since 2015, according to a broader Stress in America poll fielded last month.
Top sources of stress were the rise in prices of everyday items due to inflation (e.g., gas prices, energy bills, grocery costs, etc.) (cited by 87%), followed by supply chain issues (81%), global uncertainty (81%), Russia’s invasion of Ukraine (80%) and potential retaliation from Russia (e.g., in the form of cyberattacks or nuclear threats) (80%).
Adults also reported separation and conflict as causes for straining and/or ending of relationships. Half of adults (51%, particularly essential workers at 61%) said they have loved ones they have not been able to see in person in the past two years as a result of the COVID-19 pandemic. Strikingly, more than half of all U.S. adults (58%) reported experiencing a relationship strain or end as a result of conflicts related to the COVID-19 pandemic, including canceling events or gatherings due to COVID-19 concerns (29%); difference of opinion over some aspect of vaccines (25%); different views of the pandemic overall (25%); and difference of opinion over mask-wearing (24%).
- 30% of Us adults eat comfort food “more than the usual” when faced with a challenging or stressful event.
- 51% of US adults engage in prayer—a routine activity—when faced with a challenge or stressful situation.
- Coping mechanisms of Gen Z and Millenials experiencing stress in the US 44% of Gen Z and 40% of Millenials sleep in while exercising counts for 14% and 20% respectively.
- 49% of US adults report enduring stressful situations as a coping behavior to handle stress.
- Less than 25% of those with depression worldwide have access to mental health treatments.
Sources: CompareCamp, American Psychological Association
Stress Management Statistics
A look at the stress management techniques employed by US adults to deal with their stress, an overwhelming majority are self-care practices. Though very helpful, it does not address the stressor at the root of the problem. Stress management programs would be beneficial not only for employees but for the company in the long run.
Stress Research from the National Library of Medicine
- Stress and Cardiovascular Disease
- Stress and Cancer
- Stress and Diabetes
- Post Traumatic Stress Disorder
- Stress and Aging
- Stress in Adolenscents
- Stress and Meditation
- Stress and Yoga
- Workplace Stress
Cardiac Coherence and Post-traumatic Stress Disorder in Combat Veterans
Jay P. Ginsberg, Ph.D.; Melanie E. Berry, M.S.; Donald A Powell, Ph.D.
Alternative Therapies in Health and Medicine, A Peer-Reviewed Journal, 2010;16 (4):52-60. PDF version of the complete paper: Cardiac Coherence and PTSD in Combat Veterans
The Effect of a Biofeedback-based Stress Management Tool on Physician Stress: A Randomized Controlled Clinical Trial
Jane B. Lemaire, Jean E. Wallace, Adriane M. Lewin, Jill de Grood, Jeffrey P. Schaefer
Open Medicine 2011; 5(4)E154. PDF version of the complete paper
Coherence Training In Children With Attention-Deficit Hyperactivity Disorder: Cognitive Functions and Behavioral Changes
Anthony Lloyd, Ph.D.; Davide Brett, B.Sc.; Ketith Wesnes, Ph.D.
Alternative Therapies in Health and Medicine, A Peer-Reviewed Journal, 2010; 16 (4):34-42. PDF version of the complete paper
Coherence and Health Care Cost – RCA Actuarial Study: A Cost-Effectiveness Cohort Study
Woody Bedell; Mariette Kaszkin-Bettag, Ph.D.
Alternative Therapies in Health and Medicine, A Peer-Reviewed Journal, 2010;16 (4):26-31. PDF version of the complete paper
Recent developments in stress and anxiety research
- Published: 01 September 2021
- Volume 128 , pages 1265–1267, ( 2021 )
Cite this article
- Urs M. Nater 1 , 2
6660 Accesses
3 Citations
Explore all metrics
Avoid common mistakes on your manuscript.
Stress and anxiety are virtually omnipresent in today´s society, pervading almost all aspects of our daily lives. While each and every one of us experiences “stress” and/or “anxiety” at least to some extent at times, the phenomena themselves are far from being completely understood. In stress research, scientists are particularly grappling with the conceptual issue of how to define stress, also with regard to delimiting stress from anxiety or negative affectivity in general. Interestingly, there is no unified theory of stress, despite many attempts at defining stress and its characteristics. Consequently, the available literature relies on a variety of different theoretical approaches, though the theories of Lazarus and Folkman ( 1984 ) or McEwen ( 1998 ) are relatively pervasive in the literature. One key issue in conceptualizing stress is that research has not always differentiated between the perception of a stimulus or a situation as a stressor and the subsequent biobehavioral response (often called the “stress response”). This is important, since, for example, psychological factors such as uncontrollability and social evaluation, i.e. factors that may influence how an individual perceives a potentially stressful stimulus or situation, have been identified as characteristics that elicit particularly powerful physiological stressful responses (Dickerson and Kemeny 2004 ). At the core of the physiological stress response is a complex physiological system, which is located in both the central nervous system (CNS) and the body´s periphery. The complexity of this system necessitates a multi-dimensional assessment approach involving variables that adequately reflect all relevant components. It is also important to consider that the experience of stress and its psychobiological correlates do not occur in a vacuum, but are being shaped by numerous contextual factors (e.g. societal and cultural context, work and leisure time, family and dyadic systems, environmental variables, physical fitness, nutritional status, etc.) and dispositional factors (e.g. genetics, personality, resilience, regulatory capacities, self-efficacy, etc.). Thus, a theoretical framework needs to incorporate these factors. In sum, as stress is considered a multi-faceted and inherently multi-dimensional construct, its conceptualization and operationalization needs to reflect this (Nater 2018 ).
The goal of the World Association for Stress Related and Anxiety Disorders (WASAD) is to promote and make available basic and clinical research on stress-related and anxiety disorders. Coinciding with WASAD’s 3rd International Congress held in September 2021 in Vienna, Austria, this journal publishes a Special Issue encompassing state-of-the art research in the field of stress and anxiety. This special issue collects answers to a number of important questions that need to be addressed in current and future research. Among the most relevant issues are (1) the multi-dimensional assessment that arises as a consequence of a multi-faceted consideration of stress and anxiety, with a particular focus on doing so under ecologically valid conditions. Skoluda et al. 2021 (in this issue) argue that hair as an important source of the stress hormone cortisol should not only be taken as a complementary stress biomarker by research staff, but that lay persons could be also trained to collect hair at the study participants’ homes, thus increasing the ecological validity of studies incorporating this important measure; (2) the incongruence between psychological and biological facets of stress and anxiety that has been observed both in laboratory and field research (Campbell and Ehlert 2012 ). Interestingly, there are behavioral constructs that do show relatively high congruence. As shown in the paper of Vatheuer et al. ( 2021 ), gaze behavior while exposed to an acute social stressor correlates with salivary cortisol, thus indicating common underlying mechanisms; (3) the complex dynamics of stress-related measures that may extend over shorter (seconds to minutes), medium (hours and diurnal/circadian fluctuations), and longer (months, seasonal) time periods. In particular, momentary assessment studies are highly qualified to examine short to medium term fluctuations and interactions. In their study employing such a design, Stoffel and colleagues (Stoffel et al. 2021 ) show ecologically valid evidence for direct attenuating effects of social interactions on psychobiological stress. Using an experimental approach, on the other hand, Denk et al. ( 2021 ) examined the phenomenon of physiological synchrony between study participants; they found both cortisol and alpha-amylase physiological synchrony in participants who were in the same group while being exposed to a stressor. Importantly, these processes also unfold over time in relation to other biological systems; al’Absi and colleagues showed in their study (al’Absi et al. 2021 ) the critical role of the endogenous opioid system and its relation to stress-related analgesia; (4) the influence of contextual and dispositional factors on the biological stress response in various target samples (e.g., humans, animals, minorities, children, employees, etc.) both under controlled laboratory conditions and in everyday life environments. In this issue, Sattler and colleagues show evidence that contextual information may only matter to a certain extent, as in their study (Sattler et al. 2021 ), the biological response to a gay-specific social stressor was equally pronounced as the one to a general social stressor in gay men. Genetic information is probably the most widely researched dispositional factor; Kuhn et al. show in their paper (Kuhn et al. 2021 ) that the low expression variant of the serotonin transporter gene serves as a risk factor for increased stress reactivity, thus clearly indicating the important role of dispositional factors in stress processing. An interesting factor combining both aspects of dispositional and contextual information is maternal care; Bentele et al. ( 2021 ) in their study are able to show that there was an effect of maternal care on the amylase stress response, while no such effect was observed for cortisol. In a similar vein, Keijser et al. ( 2021 ) showed in their gene-environment interaction study that the effects of FKBP5, a gene very closely related to HPA axis regulation, and early life stress on depressive symptoms among young adults was moderated by a positive parenting style; and (5) the role of stress and anxiety as transdiagnostic factors in mental disorders, be it as an etiological factor, a variable contributing to symptom maintenance, or as a consequence of the condition itself. Stress, e.g., as a common denominator for a broad variety of psychiatric diagnoses has been extensively discussed, and stress as an etiological factor holds specific significance in the context of transdiagnostic approaches to the conceptualization and treatment of mental disorders (Wilamowska et al. 2010 ). The HPA axis, specifically, is widely known to be dysregulated in various conditions. Fischer et al. ( 2021 ) discuss in their comprehensive review the role of this important stress system in the context of patients with post-traumatic disorder. Specifically focusing on the cortisol awakening response, Rausch and colleagues provide evidence for HPA axis dysregulation in patients diagnosed with borderline personality disorder (Rausch et al. 2021 ). As part of a longitudinal project on ADHD, Szep et al. ( 2021 ) investigated the possible impact of child and maternal ADHD symptoms on mothers’ perceived chronic stress and hair cortisol concentration; although there was no direct association, the findings underline the importance of taking stress-related assessments into consideration in ADHD studies. As the HPA axis is closely interacting with the immune system, Rhein et al. ( 2021 ) examined in their study the predicting role of the cytokine IL-6 on psychotherapy outcome in patients with PTSD, indicating that high reactivity of IL-6 to a stressor at the beginning of the therapy was associated with a negative therapy outcome. The review of Kyunghee Kim et al. ( 2021 ) also demonstrated the critical role of immune pathways in the molecular changes due to antidepressant treatment. As for the therapy, the important role of cognitive-behavioral therapy with its key elements to address both stress and anxiety reduction have been shown in two studies in this special issue, evidencing its successful application in obsessive–compulsive disorder (Ivarsson et al. 2021 ; Hollmann et al. 2021 ). Thus, both stress and anxiety are crucial transdiagnostic factors in various mental disorders, and future research needs elaborate further on their role in etiology, maintenance, and treatment.
In conclusion, a number of important questions are being asked in stress and anxiety research, as has become evident above. The Special Issue on “Recent developments in stress and anxiety research” attempts to answer at least some of the raised questions, and I want to invite you to inspect the individual papers briefly introduced above in more detail.
al’Absi M, Nakajima M, Bruehl S (2021) Stress and pain: modality-specific opioid mediation of stress-induced analgesia. J Neural Transm. https://doi.org/10.1007/s00702-021-02401-4
Article PubMed Google Scholar
Bentele UU, Meier M, Benz ABE, Denk BF, Dimitroff SJ, Pruessner JC, Unternaehrer E (2021) The impact of maternal care and blood glucose availability on the cortisol stress response in fasted women. J Neural Transm (Vienna). https://doi.org/10.1007/s00702-021-02350-y
Article Google Scholar
Campbell J, Ehlert U (2012) Acute psychosocial stress: does the emotional stress response correspond with physiological responses? Psychoneuroendocrinology 37(8):1111–1134. https://doi.org/10.1016/j.psyneuen.2011.12.010
Denk B, Dimitroff SJ, Meier M, Benz ABE, Bentele UU, Unternaehrer E, Popovic NF, Gaissmaier W, Pruessner JC (2021) Influence of stress on physiological synchrony in a stressful versus non-stressful group setting. J Neural Transm (Vienna). https://doi.org/10.1007/s00702-021-02384-2
Dickerson SS, Kemeny ME (2004) Acute stressors and cortisol responses: a theoretical integration and synthesis of laboratory research. Psychol Bull 130(3):355–391
Fischer S, Schumacher T, Knaevelsrud C, Ehlert U, Schumacher S (2021) Genes and hormones of the hypothalamic-pituitary-adrenal axis in post-traumatic stress disorder. What is their role in symptom expression and treatment response? J Neural Transm (vienna). https://doi.org/10.1007/s00702-021-02330-2
Hollmann K, Allgaier K, Hohnecker CS, Lautenbacher H, Bizu V, Nickola M, Wewetzer G, Wewetzer C, Ivarsson T, Skokauskas N, Wolters LH, Skarphedinsson G, Weidle B, de Haan E, Torp NC, Compton SN, Calvo R, Lera-Miguel S, Haigis A, Renner TJ, Conzelmann A (2021) Internet-based cognitive behavioral therapy in children and adolescents with obsessive compulsive disorder: a feasibility study. J Neural Transm. https://doi.org/10.1007/s00702-021-02409-w
Ivarsson T, Melin K, Carlsson A, Ljungberg M, Forssell-Aronsson E, Starck G, Skarphedinsson G (2021) Neurochemical properties measured by 1 H magnetic resonance spectroscopy may predict cognitive behaviour therapy outcome in paediatric OCD: a pilot study. J Neural Transm. https://doi.org/10.1007/s00702-021-02407-y
Keijser R, Olofsdotter S, Nilsson WK, Åslund C (2021) Three-way interaction effects of early life stress, positive parenting and FKBP5 in the development of depressive symptoms in a general population. J Neural Transm. https://doi.org/10.1007/s00702-021-02405-0
Kuhn L, Noack H, Skoluda N, Wagels L, Rohr AK, Schulte C, Eisenkolb S, Nieratschker V, Derntl B, Habel U (2021) The association of the 5-HTTLPR polymorphism and the response to different stressors in healthy males. J Neural Transm (Vienna). https://doi.org/10.1007/s00702-021-02390-4
Kyunghee Kim H, Zai G, Hennings J, Müller DJ, Kloiber S (2021) Changes in RNA expression levels during antidepressant treatment: a systematic review. J Neural Transm. https://doi.org/10.1007/s00702-021-02394-0
Lazarus RS, Folkman S (1984) Stress, appraisal, and coping. Springer Publisher Company Inc, New York
Google Scholar
McEwen BS (1998) Protective and damaging effects of stress mediators. N Engl J Med 338(3):171–179
Article CAS Google Scholar
Nater UM (2018) The multidimensionality of stress and its assessment. Brain Behav Immun 73:159–160. https://doi.org/10.1016/j.bbi.2018.07.018
Rausch J, Flach E, Panizza A, Brunner R, Herpertz SC, Kaess M, Bertsch K (2021) Associations between age and cortisol awakening response in patients with borderline personality disorder. J Neural Transm. https://doi.org/10.1007/s00702-021-02402-3
Rhein C, Hepp T, Kraus O, von Majewski K, Lieb M, Rohleder N, Erim Y (2021) Interleukin-6 secretion upon acute psychosocial stress as a potential predictor of psychotherapy outcome in posttraumatic stress disorder. J Neural Transm (Vienna). https://doi.org/10.1007/s00702-021-02346-8
Sattler FA, Nater UM, Mewes R (2021) Gay men’s stress response to a general and a specific social stressor. J Neural Transm (Vienna). https://doi.org/10.1007/s00702-021-02380-6
Skoluda N, Piroth I, Gao W, Nater UM (2021) HOME vs. LAB hair samples for the determination of long-term steroid concentrations: a comparison between hair samples collected by laypersons and trained research staff. J Neural Transm (Vienna). https://doi.org/10.1007/s00702-021-02367-3
Stoffel M, Abbruzzese E, Rahn S, Bossmann U, Moessner M, Ditzen B (2021) Covariation of psychobiological stress regulation with valence and quantity of social interactions in everyday life: disentangling intra- and interindividual sources of variation. J Neural Transm (Vienna). https://doi.org/10.1007/s00702-021-02359-3
Szep A, Skoluda N, Schloss S, Becker K, Pauli-Pott U, Nater UM (2021) The impact of preschool child and maternal attention-deficit/hyperactivity disorder (ADHD) symptoms on mothers’ perceived chronic stress and hair cortisol. J Neural Transm (Vienna). https://doi.org/10.1007/s00702-021-02377-1
Vatheuer CC, Vehlen A, von Dawans B, Domes G (2021) Gaze behavior is associated with the cortisol response to acute psychosocial stress in the virtual TSST. J Neural Transm (Vienna). https://doi.org/10.1007/s00702-021-02344-w
Wilamowska ZA, Thompson-Hollands J, Fairholme CP, Ellard KK, Farchione TJ, Barlow DH (2010) Conceptual background, development, and preliminary data from the unified protocol for transdiagnostic treatment of emotional disorders. Depress Anxiety 27(10):882–890. https://doi.org/10.1002/da.20735
Download references
Author information
Authors and affiliations.
Department of Clinical and Health Psychology, Faculty of Psychology, University of Vienna, Vienna, Austria
Urs M. Nater
University Research Platform ‘The Stress of Life – Processes and Mechanisms Underlying Everyday Life Stress’, University of Vienna, Vienna, Austria
You can also search for this author in PubMed Google Scholar
Corresponding author
Correspondence to Urs M. Nater .
Additional information
Publisher's note.
Springer Nature remains neutral with regard to jurisdictional claims in published maps and institutional affiliations.
Rights and permissions
Reprints and permissions
About this article
Nater, U.M. Recent developments in stress and anxiety research. J Neural Transm 128 , 1265–1267 (2021). https://doi.org/10.1007/s00702-021-02410-3
Download citation
Accepted : 13 August 2021
Published : 01 September 2021
Issue Date : September 2021
DOI : https://doi.org/10.1007/s00702-021-02410-3
Share this article
Anyone you share the following link with will be able to read this content:
Sorry, a shareable link is not currently available for this article.
Provided by the Springer Nature SharedIt content-sharing initiative
- Find a journal
- Publish with us
- Track your research
Advances in stress and depression research
Affiliation.
- 1 Department of Psychology, University of British Columbia, Vancouver, British Columbia, Canada.
- PMID: 36194148
- DOI: 10.1097/YCO.0000000000000831
Purpose of review: Stress plays a central role in the onset and course of depression. However, only a subset of people who encounter stressful life events go on to experience a depressive episode. The current review highlights recent advances in understanding when, why, and for whom the stress-depression link occurs, and we identify avenues for future research.
Recent findings: In the last 18 months, researchers have taken a more nuanced perspective on the biopsychosocial mechanisms critical to the stress-depression link. For example, examination of specific facets of emotion regulation, including emotion regulation flexibility and interpersonal emotion regulation, has been critical to understanding its role in depression. Similarly, refined investigations of social support allowed researchers to identify distinct - and occasionally opposite - outcomes depending on the context or manner in which the support was provided. Researchers also documented that the stress-depression link was enhanced by dysregulation of several stress-sensitive biological systems, such as the immune system, microbiome, endocrine system, and neuroanatomical substrates.
Summary: Recent studies highlight the importance of adopting a nuanced understanding of mechanisms and moderators that explain the stress-depression link. We also encourage continued engagement in collaborative, open science that uses multiple methods to study the full breadth of human diversity.
Copyright © 2022 Wolters Kluwer Health, Inc. All rights reserved.
Publication types
- Research Support, Non-U.S. Gov't
- Depression* / psychology
- Social Support*
Here’s how you know
- U.S. Department of Health and Human Services
- National Institutes of Health
Let’s Talk About Stress—and Stress Research
Director’s Page Helene M. Langevin, M.D.
July 26, 2022
Stress is a part of life for everyone, and when it comes in short bursts, it’s not necessarily bad. Our natural “fight-or-flight” response can help us mobilize our resources to meet a challenge. But when stress persists (chronic stress), it can lead to both mental and physical health problems. In fact, longstanding evidence from multiple areas of research demonstrates that chronic stress acts like a toxin, permeating our organs and cells and triggering a negative cascade on our hormones, sleep, muscles, metabolism, immune system, and inflammatory responses. And chronic systemic inflammation is emerging as a key factor underlying more than half of all deaths from chronic diseases including heart disease, cancer, diabetes, chronic kidney disease, and nonalcoholic fatty liver.
Chronic stress has long been a focus of research for the National Center for Complementary and Integrative Health (NCCIH), and the interplay between the effects of stress on the mind and the body is an important area of research to me, personally. Many NCCIH-supported studies have looked at the potential role of complementary and integrative health interventions in helping people manage stress and its consequences. Let’s look at one area of research—the effect of stress management interventions on people who are living with a long-term health condition.
With funding from NCCIH, a group of researchers led by Dr. Lori Scott-Sheldon, who was then with The Miriam Hospital in Rhode Island, performed a series of systematic reviews on stress management for people with chronic diseases. The reviews looked at both psychological and physical effects of the interventions, and their findings were promising. For example:
- A review of studies on stress management interventions for adults with heart failure showed improvements in anxiety, depressive symptoms, quality of life, and exercise capacity in people who participated in these interventions.
- A review on mindfulness-based interventions for people living with HIV/AIDS showed reductions in anxiety and depressive symptoms and improved quality of life.
- A review of the evidence on yoga interventions for people living with HIV/AIDS showed improvements in perceived stress, positive affect, and anxiety.
- A review on mindfulness-based interventions for adults with cardiovascular disease showed improvements in anxiety, depression, distress, perceived stress, and systolic blood pressure.
- And finally, a review of yoga interventions for people with type 2 diabetes linked participation in yoga with improvements in measures of glycemic control (HbA1c and both fasting and postprandial [after eating] blood glucose) and in several risk factors for complications of diabetes—blood lipids, body mass index, waist/hip ratio, and cortisol levels (a measure related to the body’s stress response).
The diabetes review doesn’t give us final answers about the value of yoga, though, for two reasons: First, all the studies included in the review were performed outside the United States (most of them in India), and findings obtained in one population may not apply to another. Second, the studies were of only low-to-medium methodological quality, and some important study details were not available.
Fortunately, new research supported by NCCIH may help fill these gaps. Dr. Beth Bock, also of The Miriam Hospital, is leading rigorous NCCIH-funded studies to evaluate the potential role of yoga in diabetes management in U.S. populations, and this work is in progress now. An earlier pilot study showed that the yoga intervention Dr. Bock is testing was highly feasible and acceptable among people with diabetes and that it produced improvements in both psychosocial measures and blood glucose.
Whether or not we face major challenges in our lives the way that people with diabetes or other chronic health problems do, we all need to recognize the importance of stress and the impact it may be having on us. Addressing stress isn’t just about feeling better; it’s about fundamentally promoting health. We can all benefit from learning methods to mitigate stress that have well-documented beneficial effects on inflammation, oxidative stress, stress hormones, blood pressure, blood sugar, and sleep. Some of these tools, such as deep breathing and relaxation techniques, are easy to learn and can help “press reset on stress” —anytime, anywhere. And doing so can have cumulative effects—preventing stress from building up during the day, and giving us a better night’s sleep and more energy the next day for physical activity, which itself helps relieve stress. Think of it as a “positive snowball” effect, leading to better health.
And for those of us in the research community, including NCCIH, learning more about how to prevent, manage, and mitigate stress—and how best to put existing stress management tools into practice—will continue to be a high priority.

Additional Resources
- Stress Fact Sheet
- Feeling Stressed? Ways To Improve Your Well-Being (NIH News in Health)
- Stress Reset Video (The Healthy US Collaborative)
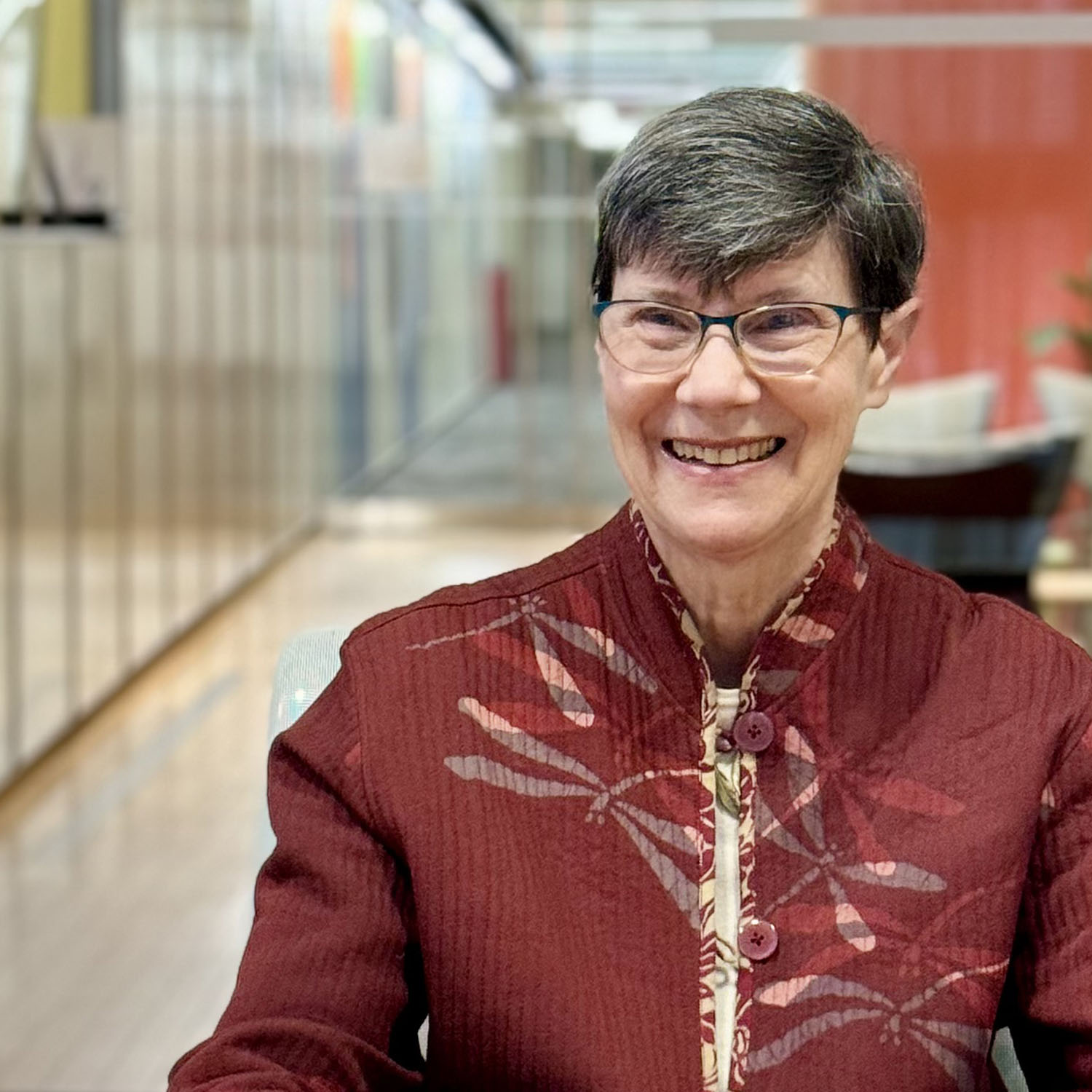
Past Messages From the Director
BRAIN at 10: A View from the National Center for Complementary and Integrative Health November 1, 2024
Expanding the Horizon in Pain Research October 29, 2024
The Promise of Applying a Whole Person Framework to Pain September 30, 2024
More messages
An official website of the United States government
Official websites use .gov A .gov website belongs to an official government organization in the United States.
Secure .gov websites use HTTPS A lock ( Lock Locked padlock icon ) or https:// means you've safely connected to the .gov website. Share sensitive information only on official, secure websites.
- Publications
- Account settings
- Advanced Search
- Journal List
The impact of stress on body function: A review
Habib yaribeygi, yunes panahi, hedayat sahraei, thomas p johnston, amirhossein sahebkar.
- Author information
- Article notes
- Copyright and License information
*To whom correspondence should be addressed: Yunes Panahi, Clinical Pharmacy Department, Faculty of Pharmacy, Baqiyatallah University of Medical Sciences, Tehran, Iran; Tel/Fax: +982188211524, E-mail: [email protected]
Received 2017 May 20; Accepted 2017 Jul 18; Collection date 2017.
This is an Open Access article distributed under the terms of the Creative Commons Attribution Licence ( http://creativecommons.org/licenses/by/4.0/ ) You are free to copy, distribute and transmit the work, provided the original author and source are credited.
Any intrinsic or extrinsic stimulus that evokes a biological response is known as stress. The compensatory responses to these stresses are known as stress responses. Based on the type, timing and severity of the applied stimulus, stress can exert various actions on the body ranging from alterations in homeostasis to life-threatening effects and death. In many cases, the pathophysiological complications of disease arise from stress and the subjects exposed to stress, e.g. those that work or live in stressful environments, have a higher likelihood of many disorders. Stress can be either a triggering or aggravating factor for many diseases and pathological conditions. In this study, we have reviewed some of the major effects of stress on the primary physiological systems of humans.
Keywords: stress, physiology, homeostasis
Abbreviations
ACTH: Adrenocorticotropic hormone
CNS: Central nervous system
CRH: Corticotropin releasing hormone
GI: Gastrointestinal
LTP: Long-term potentiation
NMDA : N-methyl-D-aspartate
VTA: Ventral tegmental area
Stress and the Brain Function Complications
For a long time, researchers suggested that hormones have receptors just in the peripheral tissues and do not gain access to the central nervous system (CNS) (Lupien and Lepage, 2001[ 63 ]). However, observations have demonstrated the effect of anti-inflammatory drugs (which are considered synthetic hormones) on behavioral and cognitive disorders and the phenomenon called “Steroid psychosis” (Clark et al., 1952[ 16 ]). In the early sixties, neuropeptides were recognized as compounds devoid of effects on the peripheral endocrine system. However, it was determined that hormones are able to elicit biological effects on different parts of the CNS and play an important role in behavior and cognition (De Kloet, 2000[ 22 ]). In 1968, McEven suggested for the first time that the brain of rodents is capable of responding to glucocorticoid (as one of the operators in the stress cascade). This hypothesis that stress can cause functional changes in the CNS was then accepted (McEwen et al., 1968[ 74 ]). From that time on, two types of corticotropic receptors (glucocorticosteroids and mineralocorticoids) were recognized (de Kloet et al., 1999[ 23 ]). It was determined that the affinity of glucocorticosteroid receptors to cortisol and corticosterone was about one tenth of that of mineralocorticoids (de Kloet et al., 1999[ 23 ]). The hippocampus area has both types of receptors, while other points of the brain have only glucocorticosteroid receptors (de Kloet et al., 1999[ 23 ]).
The effects of stress on the nervous system have been investigated for 50 years (Thierry et al., 1968[ 115 ]). Some studies have shown that stress has many effects on the human nervous system and can cause structural changes in different parts of the brain (Lupien et al., 2009[ 65 ]). Chronic stress can lead to atrophy of the brain mass and decrease its weight (Sarahian et al., 2014[ 100 ]). These structural changes bring about differences in the response to stress, cognition and memory (Lupien et al., 2009[ 65 ]). Of course, the amount and intensity of the changes are different according to the stress level and the duration of stress (Lupien et al., 2009[ 65 ]). However, it is now obvious that stress can cause structural changes in the brain with long-term effects on the nervous system (Reznikov et al., 2007[ 89 ]). Thus, it is highly essential to investigate the effects of stress on different aspects of the nervous system (Table 1 (Tab. 1) ; References in Table 1: Lupien et al., 2001[ 63 ]; Woolley et al., 1990[ 122 ]; Sapolsky et al., 1990[ 99 ]; Gould et al., 1998[ 35 ]; Bremner, 1999[ 10 ]; Seeman et al., 1997[ 108 ]; Luine et al., 1994[ 62 ]; Li et al., 2008[ 60 ]; Scholey et al., 2014[ 101 ]; Borcel et al., 2008[ 9 ]; Lupien et al., 2002[ 66 ]).
Table 1. Destructive effects of stress of CNS function.
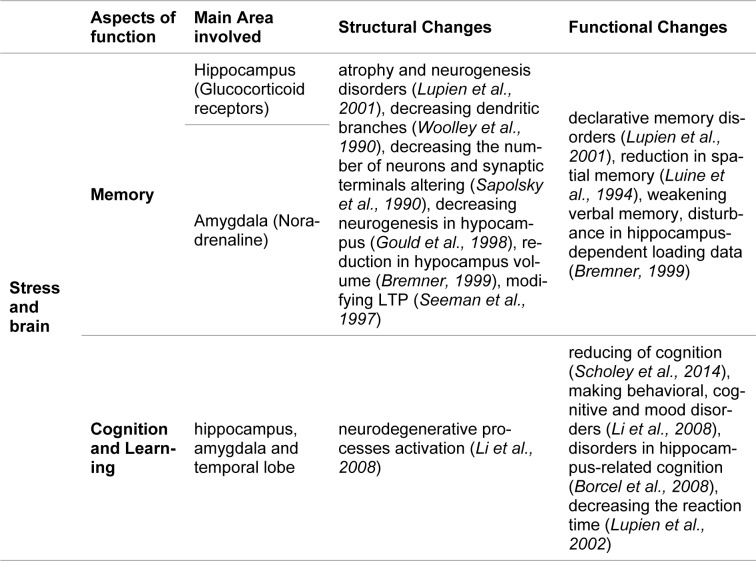
Stress and Memory
Memory is one of the important functional aspects of the CNS and it is categorized as sensory, short term, and long-term. Short term memory is dependent on the function of the frontal and parietal lobes, while long-term memory depends on the function of large areas of the brain (Wood et al., 2000[ 121 ]). However, total function of memory and the conversion of short term memory to long-term memory are dependent on the hippocampus; an area of the brain that has the highest density of glucocorticosteroid receptors and also represents the highest level of response to stress (Scoville and Milner, 1957[ 107 ]; Asalgoo et al., 2015[ 1 ]). Therefore, during the past several decades, the relationship between the hippocampus and stress have been hotly debated (Asalgoo et al., 2015[ 1 ]; Lupien and Lepage, 2001[ 63 ]). In 1968, it was proven that there were cortisol receptors in the hippocampus of rats (McEwen et al., 1968[ 74 ]). Later, in 1982, by using specific agonists of glucocorticosteroid and mineralocorticoid receptors, the existence of these two receptors in the brain and hippocampus area of rats was proven (Veldhuis et al., 1982[ 119 ]). It should also be noted that the amygdala is very important to assessing the emotional experiences of memory (Roozendaal et al., 2009[ 91 ]).
The results of past studies have demonstrated the effect of stress on the process of memory (Ghodrat et al., 2014[ 32 ]). Various studies have shown that stress can cause functional and structural changes in the hippocampus section of the brain (McEwen, 1999[ 72 ]). These structural changes include atrophy and neurogenesis disorders (Lupien and Lepage, 2001[ 63 ]). Also, chronic stress and, consequently, an increase in plasma cortisol, leads to a reduction in the number of dendritic branches (Woolley et al., 1990[ 122 ]) and the number of neurons (Sapolsky et al., 1990[ 99 ]), as well as structural changes in synaptic terminals (Sapolsky et al., 1990[ 99 ]) and decreased neurogenesis in the hippocampus tissue (Gould et al., 1998[ 35 ]). Glucocorticosteroids can induce these changes by either effecting the cellular metabolism of neurons (Lawrence and Sapolsky, 1994[ 58 ]), or increasing the sensitivity of hippocampus cells to stimulatory amino acids (Sapolsky and Pulsinelli, 1985[ 98 ]) and/or increasing the level of extracellular glutamate (Sapolsky and Pulsinelli, 1985[ 98 ]).
High concentrations of stress hormones can cause declarative memory disorders (Lupien and Lepage, 2001[ 63 ]). Animal studies have shown that stress can cause a reversible reduction in spatial memory as a result of atrophy of the hippocampus (Luine et al., 1994[ 62 ]). In fact, high plasma concentrations of glucocorticosteroids for extended periods of time can cause atrophy of the hippocampus leading to memory disorders (Issa et al., 1990[ 45 ]). Additionally, people with either Cushing's syndrome (with an increased secretion of glucocorticosteroids), or people who receive high dosages of exogenous synthetic anti-inflammatory drugs, are observed to have atrophy of the hippocampus and associated memory disorders (Ling et al., 1981[ 61 ]). MRI images taken from the brains of people with post-traumatic stress disorder (PTSD) have demonstrated a reduction in the volume of the hippocampus along with neurophysiologic effects such as a weak verbal memory (Bremner, 1999[ 10 ]). Several human studies have suggested that even common therapeutic doses of glucocorticosteroids and dexamethasone can cause problems with explicit memory (Keenan et al., 1995[ 49 ]; Kirschbaum et al., 1996[ 53 ]). Thus, there is an inverse relationship between the level of cortisol and memory (Ling et al., 1981[ 61 ]), such that increasing levels of plasma cortisol following prolonged stress leads to a reduction in memory (Kirschbaum et al., 1996[ 53 ]), which improves when the level of plasma cortisol decreases (Seeman et al., 1997[ 108 ]).
Stress also has negative effects on learning. Results from hippocampus-dependent loading data demonstrate that subjects are not as familiar with a new environment after having been exposed to a new environment (Bremner, 1999[ 10 ]). Moreover, adrenal steroids lead to alteration in long-term potentiation (LTP), which is an important process in memory formation (Bliss and Lømo, 1973[ 7 ]).
Two factors are involved in the memory process during stress. The first is noradrenaline, which creates emotional aspects of memories in the basolateral amygdala area (Joëls et al., 2011[ 47 ]). Secondly, this process is facilitated by corticosteroids. However, if the release of corticosteroids occurs a few hours earlier, it causes inhibition of the amygdala and corresponding behaviors (Joëls et al., 2011[ 47 ]). Thus, there is a mutual balance between these two hormones for creating a response in the memory process (Joëls et al., 2011[ 47 ]).
Stress does not always affect memory. Sometimes, under special conditions, stress can actually improve memory (McEwen and Lupien, 2002[ 71 ]). These conditions include non-familiarity, non-predictability, and life-threatening aspects of imposed stimulation. Under these specific conditions, stress can temporarily improve the function of the brain and, therefore, memory. In fact, it has been suggested that stress can sharpen memory in some situations (Schwabe et al., 2010[ 105 ]). For example, it has been shown that having to take a written examination can improve memory for a short period of time in examination participants. Interestingly, this condition is associated with a decrease in the level of cortisol in the saliva (Vedhara et al., 2000[ 118 ]). Other studies have shown that impending stress before learning occurs can also lead to either an increase in the power of memory (Domes et al., 2002[ 27 ]; Schwabe et al., 2008[ 102 ]), or decrease in the capacity for memory (Diamond et al., 2006[ 26 ]; Kirschbaum et al., 1996[ 53 ]). This paradox results from the type of imposed stress and either the degree of emotional connection to the stressful event (Payne et al., 2007[ 83 ]; Diamond et al., 2007[ 25 ]), or the period of time between the imposing stress and the process of learning (Diamond et al., 2007[ 25 ]).
The process of strengthening memory is usually reinforced after stress (Schwabe et al., 2012[ 103 ]). Various studies on animal and human models have shown that administration of either glucocorticosteroids, or stress shortly after learning has occurred facilitates memory (Schwabe et al., 2012[ 103 ]). Also, it has been shown that glucocorticosteroids (not mineralocorticoids) are necessary to improve learning and memory (Lupien et al., 2002[ 66 ]). However, the retrieval of events in memory after exposure to stress will be decreased (Schwabe et al., 2012[ 103 ]), which may result from the competition of updated data for storage in memory in a stressful state (de Kloet et al., 1999[ 23 ]). Some investigations have shown that either exposure to stress, or injection of glucocorticosteroids before a test to assess retention, decreases the power of memory in humans and rodents (Schwabe and Wolf, 2009[ 104 ]).
In summary, it has been concluded that the effect of stress on memory is highly dependent on the time of exposure to the stressful stimulus and, in terms of the timing of the imposed stress, memory can be either better or worse (Schwabe et al., 2012[ 103 ]). Moreover, recent studies have shown that using a specific-timed schedule of exposure to stress not only affects hippocampus-dependent memory, but also striatum-dependent memory, which highlights the role of timing of the imposed stressful stimulus (Schwabe et al., 2010[ 105 ]).
Stress, Cognition and Learning
Cognition is another important feature of brain function. Cognition means reception and perception of perceived stimuli and its interpretation, which includes learning, decision making, attention, and judgment (Sandi, 2013[ 95 ]). Stress has many effects on cognition that depend on its intensity, duration, origin, and magnitude (Sandi, 2013[ 95 ]). Similar to memory, cognition is mainly formed in the hippocampus, amygdala, and temporal lobe (McEwen and Sapolsky, 1995[ 73 ]). The net effect of stress on cognition is a reduction in cognition and thus, it is said that any behavioral steps undertaken to reduce stress leads to increase in cognition (Scholey et al., 2014[ 101 ]). In fact, stress activates some physiological systems, such as the autonomic nervous system, central neurotransmitter and neuropeptide system, and the hypothalamus-pituitary-adrenal axis, which have direct effects on neural circuits in the brain involved with data processing (Sandi, 2013[ 95 ]). Activation of stress results in the production and release of glucocorticosteroids. Because of the lipophilic properties of glucocorticosteroids, they can diffuse through the blood-brain barrier and exert long-term effects on processing and cognition (Sandi, 2013[ 95 ]).
It appears that being exposed to stress can cause pathophysiologic changes in the brain, and these changes can be manifested as behavioral, cognitive, and mood disorders (Li et al., 2008[ 60 ]). In fact, studies have shown that chronic stress can cause complications such as increased IL-6 and plasma cortisol, but decreased amounts of cAMP responsive element binding protein and brain-derived neurotrophic factor (BDNF), which is very similar to what is observed in people with depression and mood disorders that exhibit a wide range of cognitive problems (Song et al., 2006[ 114 ]). Additionally, the increased concentrations of inflammatory factors, like interleukins and TNF-α (which play an important role in creating cognitive disorders), proves a physiologic relationship between stress and mood-based cognitive disorders (Solerte et al., 2000[ 113 ]; Marsland et al., 2006[ 68 ]; Li et al., 2008[ 60 ]). Studies on animals suggest that cognitive disorders resulting from stress are created due to neuroendocrine and neuroamine factors and neurodegenerative processes (Li et al., 2008[ 60 ]). However, it should be noted that depression may not always be due to the over activation of the physiological-based stress response (Osanloo et al., 2016[ 81 ]).
Cognitive disorders following exposure to stress have been reported in past studies (Lupien and McEwen, 1997[ 64 ]). Stress has effects on cognition both acutely (through catecholamines) and chronically (through glucocorticosteroids) (McEwen and Sapolsky, 1995[ 73 ]). Acute effects are mainly caused by beta-adrenergic effects, while chronic effects are induced in a long-term manner by changes in gene expression mediated by steroids (McEwen and Sapolsky, 1995[ 73 ]). In general, many mechanisms modulate the effects of stress on cognition (McEwen and Sapolsky, 1995[ 73 ]; Mendl, 1999[ 75 ]). For instance, adrenal steroids affect the function of the hippocampus during cognition and memory retrieval in a biphasic manner (McEwen and Sapolsky, 1995[ 73 ]). In chronic stress, these steroids can destroy neurons with other stimulatory neurotransmitters (Sandi, 2013[ 95 ]). Exposure to stress can also cause disorders in hippocampus-related cognition; specifically, spatial memory (Borcel et al., 2008[ 9 ]; Sandi et al., 2003[ 96 ]). Additionally, stress can halt or decrease the genesis of neurons in the dentate gyrus area of the hippocampus (this area is one of the limited brain areas in which neurogenesis occurs in adults) (Gould and Tanapat, 1999[ 34 ]; Köhler et al., 2010[ 54 ]). Although age is a factor known to affect cognition, studies on animals have demonstrated that young rats exposed to high doses of adrenal steroids show the same level of decline in their cognition as older adult animals with normal plasma concentrations of glucocorticoids (Landfield et al., 1978[ 57 ]). Also, a decrease in the secretion of glucocorticosteroids causes preservation of spatial memory in adults and has also been shown to have neuroprotective effects (Montaron et al., 2006[ 78 ]). Other studies have shown that stress (or the injection of adrenal steroids) results in varied effects on cognition. For instance, injection of hydrocortisone at the time of its maximum plasma concentration (in the afternoon) leads to a decrease in reaction time and improves cognition and memory (Lupien et al., 2002[ 66 ]).
In summary, the adverse effects of stress on cognition are diverse and depend on the type, timing, intensity, and duration (Sandi, 2013[ 95 ]). Generally, it is believed that mild stress facilitates an improvement in cognitive function, especially in the case of virtual or verbal memory. However, if the intensity of stress passes beyond a predetermined threshold (which is different in each individual), it causes cognitive disorders, especially in memory and judgment. The disruption to memory and judgment is due to the effects of stress on the hippocampus and prefrontal cortex (Sandi, 2013[ 95 ]). Of course, it must be realized that factors like age and gender may also play a role in some cognitive disorders (Sandi, 2013[ 95 ]). Importantly, it should be emphasized that different people may exhibit varied responses in cognition when exposed to the very same stressful stimulus (Hatef et al., 2015[ 39 ]).
Stress and Immune System Functions
The relationship between stress and the immune system has been considered for decades (Khansari et al., 1990[ 50 ]; Dantzer and Kelley, 1989[ 21 ]). The prevailing attitude between the association of stress and immune system response has been that people under stress are more likely to have an impaired immune system and, as a result, suffer from more frequent illness (Khansari et al., 1990[ 50 ]). Also, old anecdotes describing resistance of some people to severe disease using the power of the mind and their thought processes, has promoted this attitude (Khansari et al., 1990[ 50 ]). In about 200 AC, Aelius Galenus (Galen of Pergamon) declared that melancholic women (who have high levels of stress and, thus, impaired immune function) are more likely to have cancer than women who were more positive and exposed to less stress (Reiche et al., 2004[ 88 ]). This may be the first recorded case about the relationship between the immune system and stress. In an old study in the early 1920's, researchers found that the activity of phagocytes in tuberculosis decreased when emotional stress was induced. In fact, it was also suggested that living with stress increases the risk of tuberculosis by suppressing the immune system (Ishigami, 1919[ 44 ]). Following this study, other researchers suggested that the probability of disease appearance increases following a sudden, major, and extremely stressful life style change (Holmes and Rahe, 1967[ 41 ]; Calabrese et al., 1987[ 12 ]).
Over the past several decades, there have been many studies investigating the role of stress on immune system function (Dantzer and Kelley, 1989[ 21 ]; Segerstrom and Miller, 2004[ 109 ]). These studies have shown that stress mediators can pass through the blood-brain barrier and exert their effects on the immune system (Khansari et al., 1990[ 50 ]). Thus, the effect of stress on the immune system is now an accepted relationship or association.
Stress can affect the function of the immune system by modulating processes in the CNS and neuroendocrine system (Khansari et al., 1990[ 50 ]; Kiecolt-Glaser and Glaser, 1991[ 51 ]). Following stress, some neuroendocrine and neural responses result in the release of corticotropin-releasing hormone (CRH), adrenocorticotropic hormone (ACTH), and other stress mediators (Carrasco and Van de Kar, 2003[ 13 ]). However, evidence suggests that the lymphatic system, which is a part of the immune system, also plays a role in releasing these mediators (Khansari et al., 1990[ 50 ]). For instance, thymus peptides, such as thymopentine, thymopoietin, and thymosin fraction-5, cause an increase in ACTH production (Goya et al., 1993[ 36 ]). Additionally, the existence of CRH in thymus has been proven (Redei, 1992[ 87 ]). It has also been proven that interleukin-1 released from phagocytes has a role in ACTH secretion (Berkenbosch et al., 1987[ 4 ]). On the other hand, natural or synthetic glucocorticosteroids (which are the final stress operators) are known as anti-inflammatory drugs and immune suppressants and their role in the inhibition of lymphocytes and macrophages has been demonstrated as well (Elenkov et al., 1999[ 28 ]; Reiche et al., 2004[ 88 ]). Moreover, their role in inhibiting the production of cytokines and other immune mediators and decreasing their effect on target cells during exposure to stress has also been determined (Reiche et al., 2004[ 88 ]).
In addition to adrenal steroids, other hormones are affected during stress. For example, the secretion of growth hormone will be halted during severe stress. A study showed that long-term administration of CRH into the brain ventricles leads to a cessation in the release of growth hormone (Rivier and Vale, 1985[ 90 ]). Stress also causes the release of opioid peptides to be changed during the time period over which the person is exposed to stress (McCarthy et al., 2001[ 70 ]). In fact, stress modifies the secretion of hormones that play a critical role in the function of the immune system (Khansari et al., 1990[ 50 ]). To date, it has been shown that various receptors for a variety of hormones involved in immune system function are adversely affected by stress. For example, ACTH, vasoactive intestinal peptide (VIP), substance P, growth hormone, prolactin, and steroids all have receptors in various tissues of the immune system and can modulate its function (De la Fuente et al., 1996[ 24 ]; Gala, 1991[ 30 ]; Mantyh, 1991[ 67 ]). In addition, active immune cells are also able to secrete several hormones; thus, some researchers believe that these hormones, as mediators of immune system, play a significant role in balancing its function (Blalock et al., 1985[ 6 ]).
Severe stress can lead to malignancy by suppressing the immune system (Reiche et al., 2004[ 88 ]). In fact, stress can decrease the activity of cytotoxic T lymphocytes and natural killer cells and lead to growth of malignant cells, genetic instability, and tumor expansion (Reiche et al., 2004[ 88 ]). Studies have shown that the plasma concentration of norepinephrine, which increases after the induction stress, has an inverse relationship with the immune function of phagocytes and lymphocytes (Reiche et al., 2004[ 88 ]). Lastly, catecholamines and opioids that are released following stress have immune-suppressing properties (Reiche et al., 2004[ 88 ]).
Stress and the Function of the Cardiovascular System
The existence of a positive association between stress and cardiovascular disease has been verified (Rozanski et al., 1999[ 93 ]). Stress, whether acute or chronic, has a deleterious effect on the function of the cardiovascular system (Rozanski et al., 1999[ 93 ]; Kario et al., 2003[ 48 ]; Herd, 1991[ 40 ]). The effects of stress on the cardiovascular system are not only stimulatory, but also inhibitory in nature (Engler and Engler, 1995[ 29 ]). It can be postulated that stress causes autonomic nervous system activation and indirectly affects the function of the cardiovascular system (Lazarus et al., 1963[ 59 ]; Vrijkotte et al., 2000[ 120 ]). If these effects occur upon activation of the sympathetic nervous system, then it mainly results in an increase in heart rate, strength of contraction, vasodilation in the arteries of skeletal muscles, a narrowing of the veins, contraction of the arteries in the spleen and kidneys, and decreased sodium excretion by the kidneys (Herd, 1991[ 40 ]). Sometimes, stress activates the parasympathetic nervous system (Pagani et al., 1991[ 82 ]). Specifically, if it leads to stimulation of the limbic system, it results in a decrease, or even a total stopping of the heart-beat, decreased contractility, reduction in the guidance of impulses by the heart stimulus-transmission network, peripheral vasodilatation, and a decline in blood pressure (Cohen et al., 2000[ 17 ]). Finally, stress can modulate vascular endothelial cell function and increase the risk of thrombosis and ischemia, as well as increase platelet aggregation (Rozanski et al., 1999[ 93 ]).
The initial effect of stress on heart function is usually on the heart rate (Vrijkotte et al., 2000[ 120 ]). Depending upon the direction of the shift in the sympatho-vagal response, the heart beat will either increase or decrease (Hall et al., 2004[ 38 ]). The next significant effect of stress on cardiovascular function is blood pressure (Laitinen et al., 1999[ 56 ]). Stress can stimulate the autonomic sympathetic nervous system to increase vasoconstriction, which can mediate an increase in blood pressure, an increase in blood lipids, disorders in blood clotting, vascular changes, atherogenesis; all, of which, can cause cardiac arrhythmias and subsequent myocardial infarction (Rozanski et al., 1999[ 93 ]; Vrijkotte et al., 2000[ 120 ]; Sgoifo et al., 1998[ 111 ]). These effects from stress are observed clinically with atherosclerosis and leads to an increase in coronary vasoconstriction (Rozanski et al., 1999[ 93 ]). Of course, there are individual differences in terms of the level of autonomic-based responses due to stress, which depends on the personal characteristics of a given individual (Rozanski et al., 1999[ 93 ]). Thus, training programs for stress management are aimed at reducing the consequences of stress and death resulting from heart disease (Engler and Engler, 1995[ 29 ]). In addition, there are gender-dependent differences in the cardiovascular response to stress and, accordingly, it has been estimated that women begin to exhibit heart disease ten years later that men, which has been attributed to the protective effects of the estrogen hormone (Rozanski et al., 1999[ 93 ]).
Studies have shown that psychological stress can cause alpha-adrenergic stimulation and, consequently, increase heart rate and oxygen demand (Rozanski et al., 1998[ 92 ], 1999[ 93 ]; Jiang et al., 1996[ 46 ]). As a result, coronary vasoconstriction is enhanced, which may increase the risk of myocardial infarction (Yeung et al., 1991[ 124 ]; Boltwood et al., 1993[ 8 ]; Dakak et al., 1995[ 20 ]). Several studies have demonstrated that psychological stress decreases the microcirculation in the coronary arteries by an endothelium-dependent mechanism and increases the risk of myocardial infarction (Dakak et al., 1995[ 20 ]). On the other hand, mental stress indirectly leads to potential engagement in risky behaviors for the heart, such as smoking, and directly leads to stimulation of the neuroendocrine system as part of the autonomic nervous system (Hornstein, 2004[ 43 ]). It has been suggested that severe mental stress can result in sudden death (Pignalberi et al., 2002[ 84 ]). Generally, stress-mediated risky behaviors that impact cardiovascular health can be summarized into five categories: an increase in the stimulation of the sympathetic nervous system, initiation and progression of myocardial ischemia, development of cardiac arrhythmias, stimulation of platelet aggregation, and endothelial dysfunction (Wu, 2001[ 123 ]).
Stress and Gastrointestinal Complications
The effects of stress on nutrition and the gastrointestinal (GI) system can be summarized with two aspects of GI function.
First, stress can affect appetite (Bagheri Nikoo et al., 2014[ 2 ]; Halataei et al., 2011[ 37 ]; Ranjbaran et al., 2013[ 86 ]). This effect is related to involvement of either the ventral tegmental area (VTA), or the amygdala via N-methyl-D-aspartate (NMDA) glutamate receptors (Nasihatkon et al., 2014[ 80 ]; Sadeghi et al., 2015[ 94 ]). However, it should also be noted that nutrition patterns have effects on the response to stress (Ghanbari et al., 2015[ 31 ]), and this suggests a bilateral interaction between nutrition and stress.
Second, stress adversely affects the normal function of GI tract. There are many studies concerning the effect of stress on the function of the GI system (Söderholm and Perdue, 2001[ 112 ]; Collins, 2001[ 18 ]). For instance, studies have shown that stress affects the absorption process, intestinal permeability, mucus and stomach acid secretion, function of ion channels, and GI inflammation (Collins, 2001[ 18 ]; Nabavizadeh et al., 2011[ 79 ]). Stress also increases the response of the GI system to inflammation and may reactivate previous inflammation and accelerate the inflammation process by secretion of mediators such as substance P (Collins, 2001[ 18 ]). As a result, there is an increase in the permeability of cells and recruitment of T lymphocytes. Lymphocyte aggregation leads to the production of inflammatory markers, activates key pathways in the hypothalamus, and results in negative feedback due to CRH secretion, which ultimately results in the appearance of GI inflammatory diseases (Collins, 2001[ 18 ]). This process can reactivate previous silent colitis (Million et al., 1999[ 76 ]; Qiu et al., 1999[ 85 ]). Mast cells play a crucial role in stress-induced effects on the GI system, because they cause neurotransmitters and other chemical factors to be released that affect the function of the GI system (Konturek et al., 2011[ 55 ]).
Stress can also alter the functional physiology of the intestine (Kiliaan et al., 1998[ 52 ]). Many inflammatory diseases, such as Crohn's disease and other ulcerative-based diseases of the GI tract, are associated with stress (Hommes et al., 2002[ 42 ]). It has been suggested that even childhood stress can lead to these diseases in adulthood (Schwartz and Schwartz, 1983[ 106 ]). Irritable bowel syndrome, which is a disease with an inflammatory origin, is highly related to stress (Gonsalkorale et al., 2003[ 33 ]). Studies on various animals suggest the existence of inflammatory GI diseases following induction of severe stress (Qiu et al., 1999[ 85 ]; Collins et al., 1996[ 19 ]). Additionally, pharmacological interventions, in an attempt to decrease the response of CRH to stress, have been shown to result in an increase in GI diseases in rats (Million et al., 1999[ 76 ]).
Altering the permeability of the mucosal membrane by perturbing the functions of mucosal mast cells may be another way that stress causes its effects on the GI system, since this is a normal process by which harmful and toxic substances are removed from the intestinal lumen (Söderholm and Perdue, 2001[ 112 ]). Also, stress can both decrease the removal of water from the lumen, as well as induce sodium and chloride secretion into the lumen. This most likely occurs by increasing the activity of the parasympathetic nervous system (Barclay and Turnberg, 1987[ 3 ]). Moreover, physical stress, such as trauma or surgery, can increase luminal permeability (Söderholm and Perdue, 2001[ 112 ]) (Table 2 (Tab. 2) ; References in Table 2: Halataei et al., 2011[ 37 ]; Ranjbaran et al., 2013[ 86 ]; Mönnikes et al., 2001[ 77 ]; Collins, 2001[ 18 ]; Nabavizadeh et al., 2011[ 79 ]; Barclay and Turnberg, 1987[ 3 ]; Million et al., 1999[ 76 ]; Gonsalkorale et al., 2003[ 33 ]).
Table 2. Stress has various effects on the function of GI system.
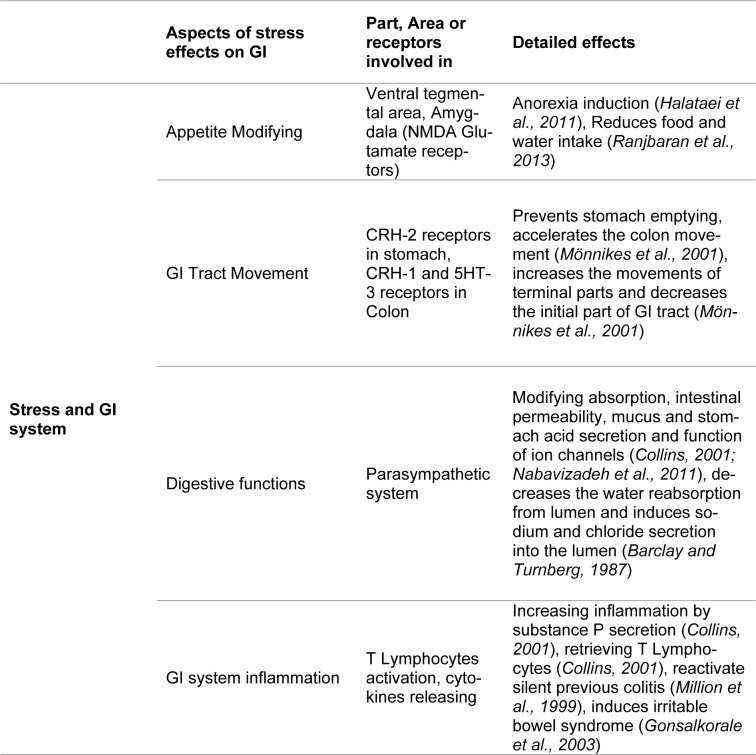
Stress also affects movement of the GI tract. In this way, it prevents stomach emptying and accelerates colonic motility (Mönnikes et al., 2001[ 77 ]). In the case of irritable bowel syndrome, stress increases the movement (contractility and motility) of the large intestine (Mönnikes et al., 2001[ 77 ]). Previous studies have revealed that CRH increases movement in the terminal sections of the GI tract and decreases the movements in the proximal sections of the GI tract (Mönnikes et al., 2001[ 77 ]). A delay in stomach emptying is likely accomplished through CRH-2 receptors, while type 1 receptors affect the colon (Mönnikes et al., 2001[ 77 ]). The effects produced by CRH are so prominent that CRH is now considered an ideal candidate for the treatment of irritable bowel syndrome (Martinez and Taché, 2006[ 69 ]). When serotonin is released in response to stress (Chaouloff, 2000[ 14 ]), it leads to an increase in the motility of the colon by stimulating 5HT-3 receptors (Mönnikes et al., 2001[ 77 ]). Moreover, it has also been suggested that stress, especially mental and emotional types of stress, increase visceral sensitivity and activate mucosal mast cells (Mönnikes et al., 2001[ 77 ]). Stimulation of the CNS by stress has a direct effect on GI-specific nervous system ( i.e. , the myenteric system or plexus) and causes the above mentioned changes in the movements of the GI tract (Bhatia and Tandon, 2005[ 5 ]). In fact, stress has a direct effect on the brain-bowel axis (Konturek et al., 2011[ 55 ]). Various clinical studies have suggested a direct effect of stress on irritable bowel syndrome, intestinal inflammation, and peptic ulcers (Konturek et al., 2011[ 55 ]).
In conclusion, the effects of stress on the GI system can be classified into six different actions: GI tract movement disorders, increased visceral irritability, altered rate and extent of various GI secretions, modified permeability of the intestinal barrier, negative effects on blood flow to the GI tract, and increased intestinal bacteria counts (Konturek et al., 2011[ 55 ]).
Stress and the Endocrine System
There is a broad and mutual relationship between stress and the endocrine system. On one hand, stress has many subtle and complex effects on the activity of the endocrine system (Sapolsky, 2002[ 97 ]; Charmandari et al., 2005[ 15 ]), while on the other hand, the endocrine system has many effects on the response to stress (Ulrich-Lai and Herman, 2009[ 117 ]; Selye, 1956[ 110 ]). Stress can either activate, or change the activity of, many endocrine processes associated with the hypothalamus, pituitary and adrenal glands, the adrenergic system, gonads, thyroid, and the pancreas (Tilbrook et al., 2000[ 116 ]; Brown-Grant et al., 1954[ 11 ]; Thierry et al., 1968[ 115 ]; Lupien and McEwen, 1997[ 64 ]). In fact, it has been suggested that it is impossible to separate the response to stress from the functions of the endocrine system. This premise has been advanced due to the fact that even a minimal amount of stress can activate the hypothalamic-pituitary-adrenal axis, which itself is intricately involved with the activation of several different hormone secreting systems (Sapolsky, 2002[ 97 ]). In different locations throughout this article, we have already discussed the effects of stress on hormones and various endocrine factors and, thus, they will not be further addressed.
Altogether, stress may induce both beneficial and harmful effects. The beneficial effects of stress involve preserving homeostasis of cells/species, which leads to continued survival. However, in many cases, the harmful effects of stress may receive more attention or recognition by an individual due to their role in various pathological conditions and diseases. As has been discussed in this review, various factors, for example, hormones, neuroendocrine mediators, peptides, and neurotransmitters are involved in the body's response to stress. Many disorders originate from stress, especially if the stress is severe and prolonged. The medical community needs to have a greater appreciation for the significant role that stress may play in various diseases and then treat the patient accordingly using both pharmacological (medications and/or nutraceuticals) and non-pharmacological (change in lifestyle, daily exercise, healthy nutrition, and stress reduction programs) therapeutic interventions. Important for the physician providing treatment for stress is the fact that all individuals vary in their response to stress, so a particular treatment strategy or intervention appropriate for one patient may not be suitable or optimal for a different patient.
Yunes Panahi and Amirhossein Sahebkar (Department of Medical Biotechnology, School of Medicine, Mashhad University of Medical Sciences, Mashhad, Iran, P.O. Box: 91779-48564, Iran; Tel: 985118002288, Fax: 985118002287, E-mail: [email protected], [email protected]) contributed equally as corresponding authors.
Conflict of interest
The authors declare that have no conflict of interest in this study.
Acknowledgement
The authors would like to thank the "Neurosciences Research Center of Baqiyatallah University of Medical Sciences" and the “Clinical Research Development Center of Baqiyatallah (a.s.) Hospital” for providing technical supports.
- 1. Asalgoo S, Jahromi G, Meftahi G, Sahraei H. Posttraumatic stress disorder (PTSD): mechanisms and possible treatments. Neurophysiology. 2015;47:482–489. [ Google Scholar ]
- 2. Bagheri Nikoo G, Khosravi M, Sahraei H, Ranjbaran M, Sarahian N, Zardooz H, et al. Effects of systemic and intra-accumbal memantine administration on the impacts of plantar electrical shock in male NMRI mice. Physiol Pharmacol. 2014;18:61–71. [ Google Scholar ]
- 3. Barclay G, Turnberg L. Effect of psychological stress on salt and water transport in the human jejunum. Gastroenterology. 1987;93:91–97. doi: 10.1016/0016-5085(87)90319-2. [ DOI ] [ PubMed ] [ Google Scholar ]
- 4. Berkenbosch F, Van Oers J, Del Rey A, Tilders F, Besedovsky H. Corticotropin-releasing factor-producing neurons in the rat activated by interleukin-1. Science. 1987;238(4826):524–526. doi: 10.1126/science.2443979. [ DOI ] [ PubMed ] [ Google Scholar ]
- 5. Bhatia V, Tandon RK. Stress and the gastrointestinal tract. J Gastroenterol Hepatol. 2005;20:332–339. doi: 10.1111/j.1440-1746.2004.03508.x. [ DOI ] [ PubMed ] [ Google Scholar ]
- 6. Blalock JE, Harbour-McMenamin D, Smith EM. Peptide hormones shared by the neuroendocrine and immunologic systems. J Immunol. 1985;13:858s–861s. [ PubMed ] [ Google Scholar ]
- 7. Bliss TV, Lømo T. Long‐lasting potentiation of synaptic transmission in the dentate area of the anaesthetized rabbit following stimulation of the perforant path. J Physiol. 1973;232:331–356. doi: 10.1113/jphysiol.1973.sp010273. [ DOI ] [ PMC free article ] [ PubMed ] [ Google Scholar ]
- 8. Boltwood MD, Taylor CB, Burke MB, Grogin H, Giacomini J. Anger report predicts coronary artery vasomotor response to mental stress in atherosclerotic segments. Am J Cardiol. 1993;72:1361–1365. doi: 10.1016/0002-9149(93)90180-k. [ DOI ] [ PubMed ] [ Google Scholar ]
- 9. Borcel E, Pérez-Alvarez L, Herrero AI, Brionne T, Varea E, Berezin V, et al. Chronic stress in adulthood followed by intermittent stress impairs spatial memory and the survival of newborn hippocampal cells in aging animals: prevention by FGL, a peptide mimetic of neural cell adhesion molecule. Behav Pharmacol. 2008;19:41–49. doi: 10.1097/FBP.0b013e3282f3fca9. [ DOI ] [ PubMed ] [ Google Scholar ]
- 10. Bremner JD. Does stress damage the brain? Biol Psychiatry. 1999;45:797–805. doi: 10.1016/s0006-3223(99)00009-8. [ DOI ] [ PubMed ] [ Google Scholar ]
- 11. Brown-Grant K, Harris G, Reichlin S. The effect of emotional and physical stress on thyroid activity in the rabbit. J Physiol. 1954;126:29–40. doi: 10.1113/jphysiol.1954.sp005189. [ DOI ] [ PMC free article ] [ PubMed ] [ Google Scholar ]
- 12. Calabrese JR, Kling MA, Gold PW. Alterations in immunocompetence during stress, bereavement, and depression: focus on neuroendocrine regulation. Am J Psychiatry. 1987;144:1123–1134. doi: 10.1176/ajp.144.9.1123. [ DOI ] [ PubMed ] [ Google Scholar ]
- 13. Carrasco GA, Van de Kar LD. Neuroendocrine pharmacology of stress. Eur J Pharmacol. 2003;463:235–272. doi: 10.1016/s0014-2999(03)01285-8. [ DOI ] [ PubMed ] [ Google Scholar ]
- 14. Chaouloff F. Serotonin, stress and corticoids. J Psychopharmacol. 2000;14:139–151. doi: 10.1177/026988110001400203. [ DOI ] [ PubMed ] [ Google Scholar ]
- 15. Charmandari E, Tsigos C, Chrousos G. Endocrinology of the stress response. Ann Rev Physiol. 2005;67:259–284. doi: 10.1146/annurev.physiol.67.040403.120816. [ DOI ] [ PubMed ] [ Google Scholar ]
- 16. Clark LD, Bauer W, Cobb S. Preliminary observations on mental disturbances occurring in patients under therapy with cortisone and ACTH. N Engl J Med. 1952;246:205–216. doi: 10.1056/NEJM195202072460601. [ DOI ] [ PubMed ] [ Google Scholar ]
- 17. Cohen H, Benjamin J, Geva AB, Matar MA, Kaplan Z, Kotler M. Autonomic dysregulation in panic disorder and in post-traumatic stress disorder: application of power spectrum analysis of heart rate variability at rest and in response to recollection of trauma or panic attacks. Psychiatry Res. 2000;96:1–13. doi: 10.1016/s0165-1781(00)00195-5. [ DOI ] [ PubMed ] [ Google Scholar ]
- 18. Collins SM. Modulation of intestinal inflammation by stress: basic mechanisms and clinical relevance (IV) Am J Physiol. 2001;280:G315–G318. doi: 10.1152/ajpgi.2001.280.3.G315. [ DOI ] [ PubMed ] [ Google Scholar ]
- 19. Collins SM, McHugh K, Jacobson K, Khan I, Riddell R, Murase K, et al. Previous inflammation alters the response of the rat colon to stress. Gastroenterology. 1996;111:1509–1515. doi: 10.1016/s0016-5085(96)70012-4. [ DOI ] [ PubMed ] [ Google Scholar ]
- 20. Dakak N, Quyyumi AA, Eisenhofer G, Goldstein DS, Cannon RO. Sympathetically mediated effects of mental stress on the cardiac microcirculation of patients with coronary artery disease. Am J Cardiol. 1995;76:125–130. doi: 10.1016/s0002-9149(99)80043-5. [ DOI ] [ PubMed ] [ Google Scholar ]
- 21. Dantzer R, Kelley KW. Stress and immunity: an integrated view of relationships between the brain and the immune system. Life Sci. 1989;44:1995–2008. doi: 10.1016/0024-3205(89)90345-7. [ DOI ] [ PubMed ] [ Google Scholar ]
- 22. de Kloet ER. Stress in the brain. Eur J Pharmacol. 2000;405:187–198. doi: 10.1016/s0014-2999(00)00552-5. [ DOI ] [ PubMed ] [ Google Scholar ]
- 23. de Kloet ER, Oitzl MS, Joëls M. Stress and cognition: are corticosteroids good or bad guys? Trends Neurosci. 1999;22:422–426. doi: 10.1016/s0166-2236(99)01438-1. [ DOI ] [ PubMed ] [ Google Scholar ]
- 24. De la Fuente M, Delgado M, Gomariz RP. VIP modulation of immune cell functions. Adv Neuroimmunol. 1996;6:75–91. doi: 10.1016/s0960-5428(96)00002-2. [ DOI ] [ PubMed ] [ Google Scholar ]
- 25. Diamond DM, Campbell AM, Park CR, Halonen J, Zoladz PR. The temporal dynamics model of emotional memory processing: a synthesis on the neurobiological basis of stress-induced amnesia, flashbulb and traumatic memories, and the Yerkes-Dodson law. Neural Plast. 2007;2007:60803. doi: 10.1155/2007/60803. [ DOI ] [ PMC free article ] [ PubMed ] [ Google Scholar ]
- 26. Diamond DM, Campbell AM, Park CR, Woodson JC, Conrad CD, Bachstetter AD, et al. Influence of predator stress on the consolidation versus retrieval of long‐term spatial memory and hippocampal spinogenesis. Hippocampus. 2006;16:571–576. doi: 10.1002/hipo.20188. [ DOI ] [ PubMed ] [ Google Scholar ]
- 27. Domes G, Heinrichs M, Reichwald U, Hautzinger M. Hypothalamic–pituitary–adrenal axis reactivity to psychological stress and memory in middle-aged women: High responders exhibit enhanced declarative memory performance. Psychoneuroendocrinology. 2002;27:843–853. doi: 10.1016/s0306-4530(01)00085-3. [ DOI ] [ PubMed ] [ Google Scholar ]
- 28. Elenkov IJ, Webster EL, Torpy DJ, Chrousos GP. Stress, corticotropin - releasing hormone, glucocorticoids, and the immune/inflammatory response: acute and chronic effects. Ann NY Acad Sci. 1999;876:1–13. doi: 10.1111/j.1749-6632.1999.tb07618.x. [ DOI ] [ PubMed ] [ Google Scholar ]
- 29. Engler MB, Engler MM. Assessment of the cardiovascular effects of stress. J Cardiovasc Nurs. 1995;10:51–63. doi: 10.1097/00005082-199510000-00005. [ DOI ] [ PubMed ] [ Google Scholar ]
- 30. Gala RR. Prolactin and growth hormone in the regulation of the immune system. Exp Biol Med. 1991;198:513–527. doi: 10.3181/00379727-198-43286b. [ DOI ] [ PubMed ] [ Google Scholar ]
- 31. Ghanbari Z, Khosravi M, Hoseini Namvar FS, Zarrin Ehteram B, Sarahian N, Sahraei H. Effect of intermittent feeding on metabolic symptoms of chronic stress in female NMRI mice. Iranian South Med J. 2015;18:982–991. [ Google Scholar ]
- 32. Ghodrat M, Sahraei H, Razjouyan J, Meftahi G. Effects of a saffron alcoholic extract on visual short-term memory in humans: a psychophysical study. Neurophysiology. 2014;46:247–253. [ Google Scholar ]
- 33. Gonsalkorale W, Perrey C, Pravica V, Whorwell P, Hutchinson I. Interleukin 10 genotypes in irritable bowel syndrome: evidence for an inflammatory component? Gut. 2003;52:91–93. doi: 10.1136/gut.52.1.91. [ DOI ] [ PMC free article ] [ PubMed ] [ Google Scholar ]
- 34. Gould E, Tanapat P. Stress and hippocampal neurogenesis. Biol Psychiatry. 1999;46:1472–1479. doi: 10.1016/s0006-3223(99)00247-4. [ DOI ] [ PubMed ] [ Google Scholar ]
- 35. Gould E, Tanapat P, McEwen BS, Flügge G, Fuchs E. Proliferation of granule cell precursors in the dentate gyrus of adult monkeys is diminished by stress. Proc Natl Acad Sci. 1998;95:3168–3171. doi: 10.1073/pnas.95.6.3168. [ DOI ] [ PMC free article ] [ PubMed ] [ Google Scholar ]
- 36. Goya R, Castro M, Hannah M, Sosa Y, Lowry P. Thymosin peptides stimulate corticotropin release by a calcium-dependent mechanism. Neuroendocrinology. 1993;57:230–235. doi: 10.1159/000126364. [ DOI ] [ PubMed ] [ Google Scholar ]
- 37. Halataei BA, Khosravi M, Arbabian S, Sahraei H, Golmanesh L, Zardooz H, et al. Saffron (Crocus sativus) Aqueous Extract and its Constituent Crocin Reduces Stress‐induced Anorexia in Mice. Phytother Res. 2011;25:1833–1838. doi: 10.1002/ptr.3495. [ DOI ] [ PubMed ] [ Google Scholar ]
- 38. Hall M, Vasko R, Buysse D, Ombao H, Chen Q, Cashmere JD, et al. Acute stress affects heart rate variability during sleep. Psychosomatic Med. 2004;66:56–62. doi: 10.1097/01.psy.0000106884.58744.09. [ DOI ] [ PubMed ] [ Google Scholar ]
- 39. Hatef B, Shiri S, Sahraei H. Why human react differently to the same sensory experiences: an emotion-cognition interaction. Neurosci J Shefaye Khatam. 2015;4:63–72. [ Google Scholar ]
- 40. Herd JA. Cardiovascular response to stress. Physiol Rev. 1991;71:305–330. doi: 10.1152/physrev.1991.71.1.305. [ DOI ] [ PubMed ] [ Google Scholar ]
- 41. Holmes TH, Rahe RH. The social readjustment rating scale. J Psychosomatic Res. 1967;11:213–218. doi: 10.1016/0022-3999(67)90010-4. [ DOI ] [ PubMed ] [ Google Scholar ]
- 42. Hommes D, Van Den Blink B, Plasse T, Bartelsman J, Xu C, Macpherson B, et al. Inhibition of stress-activated MAP kinases induces clinical improvement in moderate to severe Crohn's disease. Gastroenterology. 2002;122:7–14. doi: 10.1053/gast.2002.30770. [ DOI ] [ PubMed ] [ Google Scholar ]
- 43. Hornstein C. [Stress, anxiety and cardiovascular disease: an interdisciplinary approach (article in Spanish)]. Vertex (Buenos Aires, Argentina) 2004;15(Suppl 1):21–31. (Ger). [ PubMed ] [ Google Scholar ]
- 44. Ishigami T. The influence of psychic acts on the progress of pulmonary tuberculosis. Am Rev Tuberculosis. 1919;2:470–484. [ Google Scholar ]
- 45. Issa AM, Rowe W, Gauthier S, Meaney MJ. Hypothalamic-pituitary-adrenal activity in aged, cognitively impaired and cognitively unimpaired rats. J Neurosci. 1990;10:3247–3254. doi: 10.1523/JNEUROSCI.10-10-03247.1990. [ DOI ] [ PMC free article ] [ PubMed ] [ Google Scholar ]
- 46. Jiang W, Babyak M, Krantz DS, Waugh RA, Coleman RE, Hanson MM, et al. Mental stress - induced myocardial ischemia and cardiac events. JAMA. 1996;275:1651–1656. doi: 10.1001/jama.275.21.1651. [ DOI ] [ PubMed ] [ Google Scholar ]
- 47. Joëls M, Fernandez G, Roozendaal B. Stress and emotional memory: a matter of timing. Trends Cognit Sci. 2011;15:280–288. doi: 10.1016/j.tics.2011.04.004. [ DOI ] [ PubMed ] [ Google Scholar ]
- 48. Kario K, McEwen B, Pickering T. Disasters and the heart: a review of the effects of earthquake-induced stress on cardiovascular disease. Hypertension Res. 2003;26:355–367. doi: 10.1291/hypres.26.355. [ DOI ] [ PubMed ] [ Google Scholar ]
- 49. Keenan P, Jacobson M, Soleymani R, Newcomer J. Commonly used therapeutic doses of glucocorticoids impair explicit memory. Ann NY Acad Sci. 1995;761:400–402. doi: 10.1111/j.1749-6632.1995.tb31402.x. [ DOI ] [ PubMed ] [ Google Scholar ]
- 50. Khansari DN, Murgo AJ, Faith RE. Effects of stress on the immune system. Immunol Today. 1990;11:170–175. doi: 10.1016/0167-5699(90)90069-l. [ DOI ] [ PubMed ] [ Google Scholar ]
- 51. Kiecolt-Glaser JK, Glaser R. Stress and immune function in humans. In: Ader R, editor. Psychoneuroimmunology. 2nd. London: Academic Press; 1991. p. 849–867. [ Google Scholar ]
- 52. Kiliaan AJ, Saunders PR, Bijlsma PB, Berin MC, Taminiau JA, Groot JA, et al. Stress stimulates transepithelial macromolecular uptake in rat jejunum. Am J Physiol. 1998;275:G1037–G1044. doi: 10.1152/ajpgi.1998.275.5.G1037. [ DOI ] [ PubMed ] [ Google Scholar ]
- 53. Kirschbaum C, Wolf OT, May M, Wippich W, Hellhammer DH. Stress-and treatment-induced elevations of cortisol levels associated with impaired declarative memory in healthy adults. Life Sci. 1996;58:1475–1483. doi: 10.1016/0024-3205(96)00118-x. [ DOI ] [ PubMed ] [ Google Scholar ]
- 54. Köhler S, Thomas AJ, Lloyd A, Barber R, Almeida OP, O’Brien JT. White matter hyperintensities, cortisol levels, brain atrophy and continuing cognitive deficits in late-life depression. Brit J Psychiatry. 2010;196:143–149. doi: 10.1192/bjp.bp.109.071399. [ DOI ] [ PubMed ] [ Google Scholar ]
- 55. Konturek PC, Brzozowski T, Konturek SJ. Stress and the gut: pathophysiology, clinical consequences, diagnostic approach and treatment options. J Physiol Pharmacol. 2011;62:591–599. [ PubMed ] [ Google Scholar ]
- 56. Laitinen T, Hartikainen J, Niskanen L, Geelen G, Länsimies E. Sympathovagal balance is major determinant of short-term blood pressure variability in healthy subjects. Am J Physiol. 1999;276:H1245–H1252. doi: 10.1152/ajpheart.1999.276.4.H1245. [ DOI ] [ PubMed ] [ Google Scholar ]
- 57. Landfield PW, Waymire J, Lynch G. Hippocampal aging and adrenocorticoids: quantitative correlations. Science. 1978;202:1098–1102. doi: 10.1126/science.715460. [ DOI ] [ PubMed ] [ Google Scholar ]
- 58. Lawrence MS, Sapolsky RM. Glucocorticoids accelerate ATP loss following metabolic insults in cultured hippocampal neurons. Brain Res. 1994;646:303–306. doi: 10.1016/0006-8993(94)90094-9. [ DOI ] [ PubMed ] [ Google Scholar ]
- 59. Lazarus RS, Speisman JC, Mordkoff AM. The relationship between autonomic indicators of psychological stress: Heart rate and skin conductance. Psychosomatic Med. 1963;25:19–30. [ Google Scholar ]
- 60. Li S, Wang C, Wang W, Dong H, Hou P, Tang Y. Chronic mild stress impairs cognition in mice: from brain homeostasis to behavior. Life Sci. 2008;82:934–942. doi: 10.1016/j.lfs.2008.02.010. [ DOI ] [ PubMed ] [ Google Scholar ]
- 61. Ling MH, Perry PJ, Tsuang MT. Side effects of corticosteroid therapy. Psychiatric aspects. Arch Gen Psychiatry. 1981;38:471–477. doi: 10.1001/archpsyc.1981.01780290105011. [ DOI ] [ PubMed ] [ Google Scholar ]
- 62. Luine V, Villegas M, Martinez C, McEwen BS. Repeated stress causes reversible impairments of spatial memory performance. Brain Res. 1994;639:167–170. doi: 10.1016/0006-8993(94)91778-7. [ DOI ] [ PubMed ] [ Google Scholar ]
- 63. Lupien SJ, Lepage M. Stress, memory, and the hippocampus: can't live with it, can't live without it. Behav Brain Res. 2001;127:137–158. doi: 10.1016/s0166-4328(01)00361-8. [ DOI ] [ PubMed ] [ Google Scholar ]
- 64. Lupien SJ, McEwen BS. The acute effects of corticosteroids on cognition: integration of animal and human model studies. Brain Res Rev. 1997;24:1–27. doi: 10.1016/s0165-0173(97)00004-0. [ DOI ] [ PubMed ] [ Google Scholar ]
- 65. Lupien SJ, McEwen BS, Gunnar MR, Heim C. Effects of stress throughout the lifespan on the brain, behaviour and cognition. Nat Rev Neurosci. 2009;10:434–445. doi: 10.1038/nrn2639. [ DOI ] [ PubMed ] [ Google Scholar ]
- 66. Lupien SJ, Wilkinson CW, Brière S, Ménard C, Kin NNY, Nair N. The modulatory effects of corticosteroids on cognition: studies in young human populations. Psychoneuroendocrinology. 2002;27:401–416. doi: 10.1016/s0306-4530(01)00061-0. [ DOI ] [ PubMed ] [ Google Scholar ]
- 67. Mantyh PW. Substance P and the inflammatory and immune response. Ann NY Acad Sci. 1991;632:71. doi: 10.1111/j.1749-6632.1991.tb33114.x. [ DOI ] [ PubMed ] [ Google Scholar ]
- 68. Marsland AL, Petersen KL, Sathanoori R, Muldoon MF, Neumann SA, Ryan C, et al. Interleukin-6 covaries inversely with cognitive performance among middle-aged community volunteers. Psychosomatic Med. 2006;68:895–903. doi: 10.1097/01.psy.0000238451.22174.92. [ DOI ] [ PubMed ] [ Google Scholar ]
- 69. Martinez V, Taché Y. CRF1 receptors as a therapeutic target for irritable bowel syndrome. Curr Pharm Des. 2006;12:4071–4088. doi: 10.2174/138161206778743637. [ DOI ] [ PubMed ] [ Google Scholar ]
- 70. McCarthy L, Wetzel M, Sliker JK, Eisenstein TK, Rogers TJ. Opioids, opioid receptors, and the immune response. Drug Alcohol Depend. 2001;62:111–123. doi: 10.1016/s0376-8716(00)00181-2. [ DOI ] [ PubMed ] [ Google Scholar ]
- 71. McEwen B, Lupien S. Stress: hormonal and neural aspects. In: Ramachandran VS, editor. Encyclopedia of the human brain. Amsterdam: Elsevier; 2002. pp. 463–474. [ Google Scholar ]
- 72. McEwen BS. Stress and hippocampal plasticity. Ann Rev Neurosci. 1999;22:105–122. doi: 10.1146/annurev.neuro.22.1.105. [ DOI ] [ PubMed ] [ Google Scholar ]
- 73. McEwen BS, Sapolsky RM. Stress and cognitive function. Curr Opin Neurobiol. 1995;5:205–216. doi: 10.1016/0959-4388(95)80028-x. [ DOI ] [ PubMed ] [ Google Scholar ]
- 74. McEwen BS, Weiss JM, Schwartz LS. Selective retention of corticosterone by limbic structures in rat brain. Nature. 1968;220(5170):911–912. doi: 10.1038/220911a0. [ DOI ] [ PubMed ] [ Google Scholar ]
- 75. Mendl M. Performing under pressure: stress and cognitive function. Appl Animal Behav Sci. 1999;65:221–244. [ Google Scholar ]
- 76. Million M, Taché Y, Anton P. Susceptibility of Lewis and Fischer rats to stress-induced worsening of TNB-colitis: protective role of brain CRF. Am J Physiol. 1999;276:G1027–G1036. doi: 10.1152/ajpgi.1999.276.4.G1027. [ DOI ] [ PubMed ] [ Google Scholar ]
- 77. Mönnikes H, Tebbe J, Hildebrandt M, Arck P, Osmanoglou E, Rose M, et al. Role of stress in functional gastrointestinal disorders. Digest Dis. 2001;19:201–211. doi: 10.1159/000050681. [ DOI ] [ PubMed ] [ Google Scholar ]
- 78. Montaron M, Drapeau E, Dupret D, Kitchener P, Aurousseau C, Le Moal M, et al. Lifelong corticosterone level determines age-related decline in neurogenesis and memory. Neurobiol Aging. 2006;27:645–654. doi: 10.1016/j.neurobiolaging.2005.02.014. [ DOI ] [ PubMed ] [ Google Scholar ]
- 79. Nabavizadeh F, Vahedian M, Sahraei H, Adeli S, Salimi E. Physical and psychological stress have similar effects on gastric acid and pepsin secretions in rat. J Stress Physiol Biochem. 2011;7:164–174. [ Google Scholar ]
- 80. Nasihatkon ZS, Khosravi M, Bourbour Z, Sahraei H, Ranjbaran M, Hassantash SM, et al. Inhibitory effect of nmda receptors in the ventral tegmental area on hormonal and eating behavior responses to stress in rats. Behav Neurol. 2014;2014:294149. doi: 10.1155/2014/294149. [ DOI ] [ PMC free article ] [ PubMed ] [ Google Scholar ]
- 81. Osanloo N, Najafi-Abedi A, Jafari F, Javid F, Pirpiran M, Memar-Jafari MR, et al. Papaver rhoeas L. hydroalcoholic extract exacerbates forced swimming test-induced depression in mice. Basic Clin Neurosci. 2016;7:195–202. doi: 10.15412/J.BCN.03070304. [ DOI ] [ PMC free article ] [ PubMed ] [ Google Scholar ]
- 82. Pagani M, Mazzuero G, Ferrari A, Liberati D, Cerutti S, Vaitl D, et al. Sympathovagal interaction during mental stress. A study using spectral analysis of heart rate variability in healthy control subjects and patients with a prior myocardial infarction. Circulation. 1991;83:II43–II51. [ PubMed ] [ Google Scholar ]
- 83. Payne JD, Jackson ED, Hoscheidt S, Ryan L, Jacobs WJ, Nadel L. Stress administered prior to encoding impairs neutral but enhances emotional long-term episodic memories. Learning & Memory. 2007;14:861–868. doi: 10.1101/lm.743507. [ DOI ] [ PMC free article ] [ PubMed ] [ Google Scholar ]
- 84. Pignalberi C, Ricci R, Santini M. Psychological stress and sudden death. Ital Heart J (Suppl) 2002;3:1011–1021. [ PubMed ] [ Google Scholar ]
- 85. Qiu B, Vallance B, Blennerhassett P, Collins S. The role of CD4+ lymphocytes in the susceptibility of mice to stress-induced reactivation of experimental colitis. Nat Med. 1999;5:1178–1182. doi: 10.1038/13503. [ DOI ] [ PubMed ] [ Google Scholar ]
- 86. Ranjbaran M, Mirzaei P, Lotfi F, Behzadi S, Sahraei H. Reduction of metabolic signs of acute stress in male mice by papaver Rhoaes hydro-alcoholic extract. Pakistan J Biol Sci. 2013;16:1016–1021. doi: 10.3923/pjbs.2013.1016.1021. [ DOI ] [ PubMed ] [ Google Scholar ]
- 87. Redei E. Immuno-reactive and bioactive corticotropin-releasing factor in rat thymus. Neuroendocrinology. 1992;55:115–118. doi: 10.1159/000126104. [ DOI ] [ PubMed ] [ Google Scholar ]
- 88. Reiche EMV, Nunes SOV, Morimoto HK. Stress, depression, the immune system, and cancer. Lancet Oncol. 2004;5:617–625. doi: 10.1016/S1470-2045(04)01597-9. [ DOI ] [ PubMed ] [ Google Scholar ]
- 89. Reznikov LR, Grillo CA, Piroli GG, Pasumarthi RK, Reagan LP, Fadel J. Acute stress‐mediated increases in extracellular glutamate levels in the rat amygdala: differential effects of antidepressant treatment. Eur J Neurosci. 2007;25:3109–3114. doi: 10.1111/j.1460-9568.2007.05560.x. [ DOI ] [ PubMed ] [ Google Scholar ]
- 90. Rivier C, Vale W. Effect of the long-term administration of corticotropin-releasing factor on the pituitary-adrenal and pituitary-gonadal axis in the male rat. J Clin Invest. 1985;75:689. doi: 10.1172/JCI111748. [ DOI ] [ PMC free article ] [ PubMed ] [ Google Scholar ]
- 91. Roozendaal B, McEwen BS, Chattarji S. Stress, memory and the amygdala. Nat Rev Neurosci. 2009;10:423–433. doi: 10.1038/nrn2651. [ DOI ] [ PubMed ] [ Google Scholar ]
- 92. Rozanski A, Bairey CN, Krantz DS, Friedman J, Resser KJ, Morell M, et al. Mental stress and the induction of silent myocardial ischemia in patients with coronary artery disease. N Engl J Med. 1988;318:1005–1012. doi: 10.1056/NEJM198804213181601. [ DOI ] [ PubMed ] [ Google Scholar ]
- 93. Rozanski A, Blumenthal JA, Kaplan J. Impact of psychological factors on the pathogenesis of cardiovascular disease and implications for therapy. Circulation. 1999;99:2192–2217. doi: 10.1161/01.cir.99.16.2192. [ DOI ] [ PubMed ] [ Google Scholar ]
- 94. Sadeghi B, Sahraei H, Zardooz H, Alibeik H, Sarahian N. Effects of intra-amygdala memantine infusion on metabolic symptoms induced by chronic stress in male NMRI mice. Koomesh. 2015;16:Pe376–83, En350. [ Google Scholar ]
- 95. Sandi C. Stress and cognition. Wiley Interdisciplinary Reviews: Cognitive Science. 2013;4:245–261. doi: 10.1002/wcs.1222. [ DOI ] [ PubMed ] [ Google Scholar ]
- 96. Sandi C, Davies HA, Cordero MI, Rodriguez JJ, Popov VI, Stewart MG. Rapid reversal of stress induced loss of synapses in CA3 of rat hippocampus following water maze training. Eur J Neurosci. 2003;17:2447–2456. doi: 10.1046/j.1460-9568.2003.02675.x. [ DOI ] [ PubMed ] [ Google Scholar ]
- 97. Sapolsky RM. Endocrinology of the stress-response. In: Becker JB, Breedlove SM, Crews D, McCarthy MM, editors. Behavioral endocrinology. 2nd. Cambridge, MA: MIT Press; 2002. pp. 409–450. [ Google Scholar ]
- 98. Sapolsky RM, Pulsinelli WA. Glucocorticoids potentiate ischemic injury to neurons: therapeutic implications. Science. 1985;229:1397–1400. doi: 10.1126/science.4035356. [ DOI ] [ PubMed ] [ Google Scholar ]
- 99. Sapolsky RM, Uno H, Rebert CS, Finch CE. Hippocampal damage associated with prolonged glucocorticoid exposure in primates. J Neurosci. 1990;10:2897–2902. doi: 10.1523/JNEUROSCI.10-09-02897.1990. [ DOI ] [ PMC free article ] [ PubMed ] [ Google Scholar ]
- 100. Sarahian N, Sahraei H, Zardooz H, Alibeik H, Sadeghi B. Effect of memantine administration within the nucleus accumbens on changes in weight and volume of the brain and adrenal gland during chronic stress in female mice. Modares J Med Sci: Pathobiology. 2014;17:71–82. [ Google Scholar ]
- 101. Scholey A, Gibbs A, Neale C, Perry N, Ossoukhova A, Bilog V, et al. Anti-stress effects of lemon balm-containing foods. Nutrients. 2014;6:4805–4821. doi: 10.3390/nu6114805. [ DOI ] [ PMC free article ] [ PubMed ] [ Google Scholar ]
- 102. Schwabe L, Bohringer A, Chatterjee M, Schachinger H. Effects of pre-learning stress on memory for neutral, positive and negative words: Different roles of cortisol and autonomic arousal. Neurobiol Learn Mem. 2008;90:44–53. doi: 10.1016/j.nlm.2008.02.002. [ DOI ] [ PubMed ] [ Google Scholar ]
- 103. Schwabe L, Joëls M, Roozendaal B, Wolf OT, Oitzl MS. Stress effects on memory: an update and integration. Neurosci Biobehav Rev. 2012;36:1740–1749. doi: 10.1016/j.neubiorev.2011.07.002. [ DOI ] [ PubMed ] [ Google Scholar ]
- 104. Schwabe L, Wolf OT. The context counts: congruent learning and testing environments prevent memory retrieval impairment following stress. Cogn Affect Behav Neurosci. 2009;9:229–236. doi: 10.3758/CABN.9.3.229. [ DOI ] [ PubMed ] [ Google Scholar ]
- 105. Schwabe L, Wolf OT, Oitzl MS. Memory formation under stress: quantity and quality. Neurosci Biobehav Rev. 2010;34:584–591. doi: 10.1016/j.neubiorev.2009.11.015. [ DOI ] [ PubMed ] [ Google Scholar ]
- 106. Schwartz RA, Schwartz IK. Psychiatric disorders associated with Crohn's disease. Int J Psychiat Med. 1983;12:67–73. doi: 10.2190/fl8y-1wu6-1n5l-1fqv. [ DOI ] [ PubMed ] [ Google Scholar ]
- 107. Scoville WB, Milner B. Loss of recent memory after bilateral hippocampal lesions. J Neurol Neurosurg Psychiatry. 1957;20:11–21. doi: 10.1136/jnnp.20.1.11. [ DOI ] [ PMC free article ] [ PubMed ] [ Google Scholar ]
- 108. Seeman TE, McEwen BS, Singer BH, Albert MS, Rowe JW. Increase in urinary cortisol excretion and memory declines: MacArthur studies of successful aging. J Clin Endocrinol Metab. 1997;82:2458–2465. doi: 10.1210/jcem.82.8.4173. [ DOI ] [ PubMed ] [ Google Scholar ]
- 109. Segerstrom SC, Miller GE. Psychological stress and the human immune system: a meta-analytic study of 30 years of inquiry. Psychol Bull. 2004;130:601–630. doi: 10.1037/0033-2909.130.4.601. [ DOI ] [ PMC free article ] [ PubMed ] [ Google Scholar ]
- 110. Selye H. Endocrine reactions during stress. Anesthesia & Analgesia. 1956;35:182–193. [ PubMed ] [ Google Scholar ]
- 111. Sgoifo A, De Boer SF, Buwalda B, Korte-Bouws G, Tuma J, Bohus B, et al. Vulnerability to arrhythmias during social stress in rats with different sympathovagal balance. Am J Physiol. 1998;275:H460–H466. doi: 10.1152/ajpheart.1998.275.2.H460. [ DOI ] [ PubMed ] [ Google Scholar ]
- 112. Söderholm JD, Perdue MH., II Stress and intestinal barrier function. Am J Physiol. 2001;280:G7–G13. doi: 10.1152/ajpgi.2001.280.1.G7. [ DOI ] [ PubMed ] [ Google Scholar ]
- 113. Solerte S, Cravello L, Ferrari E, Fioravanti M. Overproduction of IFN‐γ and TNF‐α from natural killer (NK) cells is associated with abnormal NK reactivity and cognitive derangement in Alzheimer's disease. Ann NY Acad Sci. 2000;917:331–340. doi: 10.1111/j.1749-6632.2000.tb05399.x. [ DOI ] [ PubMed ] [ Google Scholar ]
- 114. Song L, Che W, Min-Wei W, Murakami Y, Matsumoto K. Impairment of the spatial learning and memory induced by learned helplessness and chronic mild stress. Pharmacol Biochem Behav. 2006;83:186–193. doi: 10.1016/j.pbb.2006.01.004. [ DOI ] [ PubMed ] [ Google Scholar ]
- 115. Thierry A-M, Javoy F, Glowinski J, Kety SS. Effects of stress on the metabolism of norepinephrine, dopamine and serotonin in the central nervous system of the rat. I. Modifications of norepinephrine turnover. J Pharmacol Exp Ther. 1968;163:163–171. [ PubMed ] [ Google Scholar ]
- 116. Tilbrook A, Turner A, Clarke I. Effects of stress on reproduction in non-rodent mammals: the role of glucocorticoids and sex differences. Rev Reprod. 2000;5:105–113. doi: 10.1530/ror.0.0050105. [ DOI ] [ PubMed ] [ Google Scholar ]
- 117. Ulrich-Lai YM, Herman JP. Neural regulation of endocrine and autonomic stress responses. Nat Rev Neurosci. 2009;10:397–409. doi: 10.1038/nrn2647. [ DOI ] [ PMC free article ] [ PubMed ] [ Google Scholar ]
- 118. Vedhara K, Hyde J, Gilchrist I, Tytherleigh M, Plummer S. Acute stress, memory, attention and cortisol. Psychoneuroendocrinology. 2000;25:535–549. doi: 10.1016/s0306-4530(00)00008-1. [ DOI ] [ PubMed ] [ Google Scholar ]
- 119. Veldhuis HD, Van Koppen C, Van Ittersum M, de Kloet RE. Specificity of the adrenal steroid receptor system in rat hippocampus. Endocrinology. 1982;110:2044–2051. doi: 10.1210/endo-110-6-2044. [ DOI ] [ PubMed ] [ Google Scholar ]
- 120. Vrijkotte TG, Van Doornen LJ, De Geus EJ. Effects of work stress on ambulatory blood pressure, heart rate, and heart rate variability. Hypertension. 2000;35:880–886. doi: 10.1161/01.hyp.35.4.880. [ DOI ] [ PubMed ] [ Google Scholar ]
- 121. Wood ER, Dudchenko PA, Robitsek RJ, Eichenbaum H. Hippocampal neurons encode information about different types of memory episodes occurring in the same location. Neuron. 2000;27:623–633. doi: 10.1016/s0896-6273(00)00071-4. [ DOI ] [ PubMed ] [ Google Scholar ]
- 122. Woolley CS, Gould E, McEwen BS. Exposure to excess glucocorticoids alters dendritic morphology of adult hippocampal pyramidal neurons. Brain Res. 1990;531:225–231. doi: 10.1016/0006-8993(90)90778-a. [ DOI ] [ PubMed ] [ Google Scholar ]
- 123. Wu Z. Epidemiological studies on the relationship between psychosocial factors and cardiovascular disease. Zhongguo yi xue ke xue yuan xue bao. Acta Acad Med Sin. 2001;23:73–7, 82. [ PubMed ] [ Google Scholar ]
- 124. Yeung AC, Vekshtein VI, Krantz DS, Vita JA, Ryan TJ, Jr, Ganz P, et al. The effect of atherosclerosis on the vasomotor response of coronary arteries to mental stress. N Engl J Med. 1991;325:1551–1556. doi: 10.1056/NEJM199111283252205. [ DOI ] [ PubMed ] [ Google Scholar ]
- View on publisher site
- PDF (163.3 KB)
- Collections
Similar articles
Cited by other articles, links to ncbi databases.
- Download .nbib .nbib
- Format: AMA APA MLA NLM
Add to Collections

- Get Press Releases

- Media Contacts
- News Releases
- Photos & B-Roll Downloads
- VUMC Facts and Figures
- Credo Award
- DAISY Award
- Elevate Team Award
- Health, Yes
- Employee Spotlight
- Five Pillar Leader Award
- Patient Spotlight
- Pets of VUMC
- Tales of VUMC Past
- All Voice Stories
Explore by Highlight
- Community & Giving
- Education & Training
- Growth & Finance
- Leadership Perspectives
- VUMC People
Explore by Topic
- Emergency & Trauma
- Genetics & Genomics
- Health Policy
- Infectious Diseases
- Tech & Health
- Women's Health
Explore by Location
- Monroe Carell Jr. Children’s Hospital at Vanderbilt
- Vanderbilt Bedford County Hospital
- Vanderbilt Health One Hundred Oaks
- Vanderbilt Health Affiliated Network
- Vanderbilt-Ingram Cancer Center
- Vanderbilt Kennedy Center
- Vanderbilt Psychiatric Hospital
- Vanderbilt Wilson County Hospital
- Vanderbilt Stallworth Rehabilitation Hospital
- Vanderbilt Tullahoma-Harton Hospital
- Vanderbilt University Hospital

- Photos & B-Roll Downloads
Featured Story
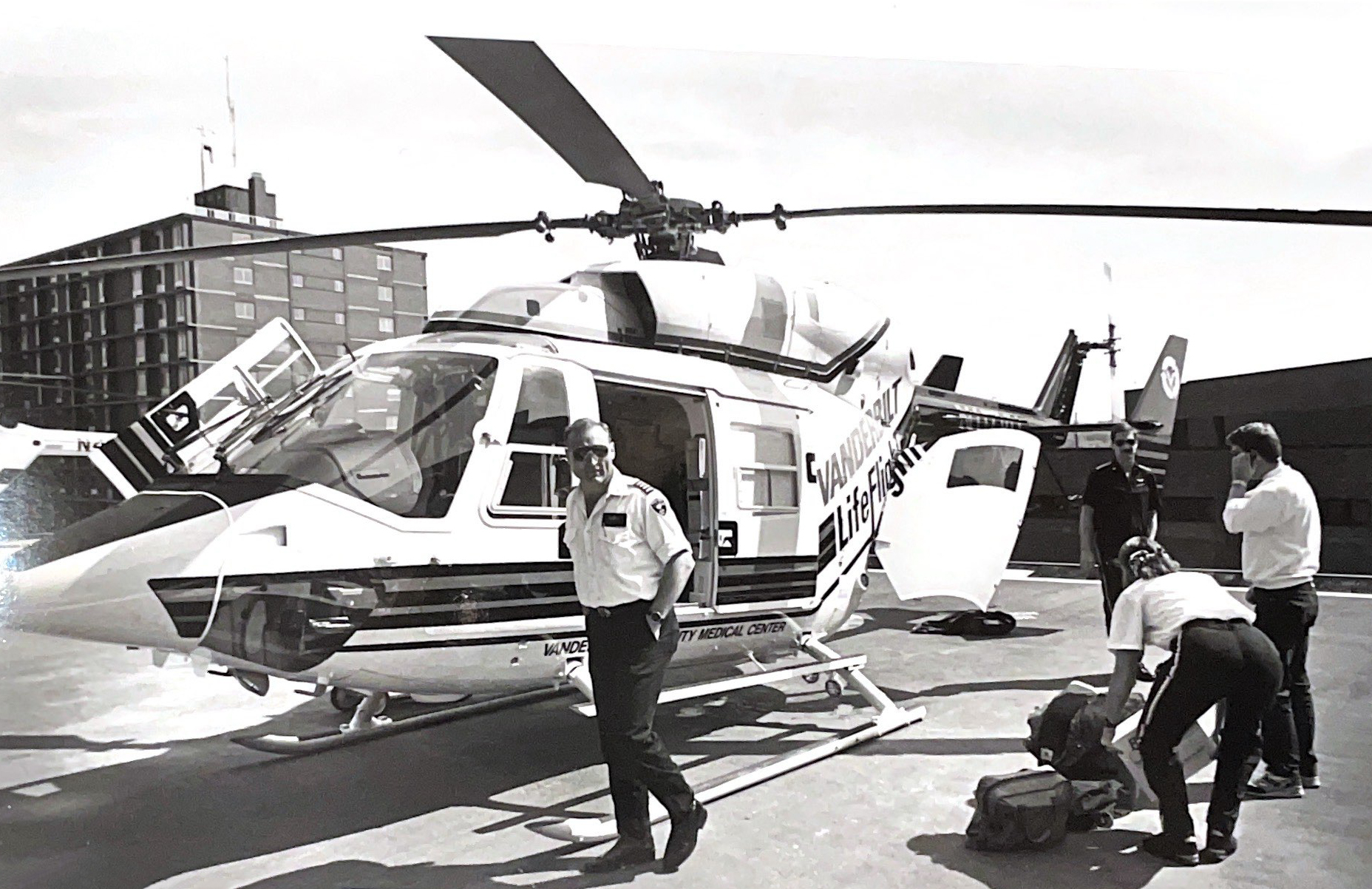
Emergency & Trauma
The lifeflight legacy: 40 years in 40 photos, november 7, 2024, vanderbilt kennedy center stress and early adversity lab research study recruiting expecting families for 18-month study on infant development.
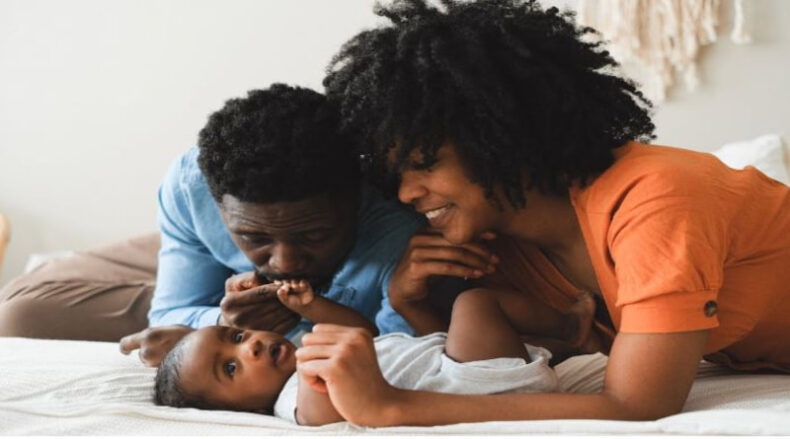
In the Stress and Early Adversity (SEA) Lab at Vanderbilt University, director Kathryn L. Humphreys and her team study how children’s experiences are associated with development. The ABC Study is a research project that aims to understand how children grow and develop through their daily experiences and relationships. As a participant, you and your child’s other caregiver (if applicable) will be involved in study sessions during pregnancy and when your child is 1, 6, 12, and 18 months old. These study sessions will take place at your home, at our research lab at Vanderbilt University, and at the Vanderbilt Imaging Center where your child will undergo magnetic resonance imaging (MRI) scans to see how their brain is growing across the first year of life. MRI is safe for infants and has been used with infants for 20 years. It requires no medication and involves no radiation.
During the study visits, we will be asking you and your family questions about your thoughts, feelings, and experiences. We will also provide wearable devices to study family interaction patterns and learn more about your child’s daily life. Overall, the study will take about 30 hours across the visits. If you complete all parts of the study, you can earn up to $1,300, and we also provide small gifts, including 3D miniatures of your child’s brain, and written reports on your child’s development.
Researchers understand that parents with young children are busy, so we offer flexible scheduling, including daytime, nights, and weekends. If you have other children, we can provide childcare by trained staff members during the sessions, and we can also help provide or cover the costs of transportation. Researchers also know that participating in a study like this might seem like a lot of work, but we hope to find families who are excited to be part of this one-of-a-kind research project.
If interested, call (615) 348-5752. email [email protected] , or visit VUsealab.com .
Related Articles
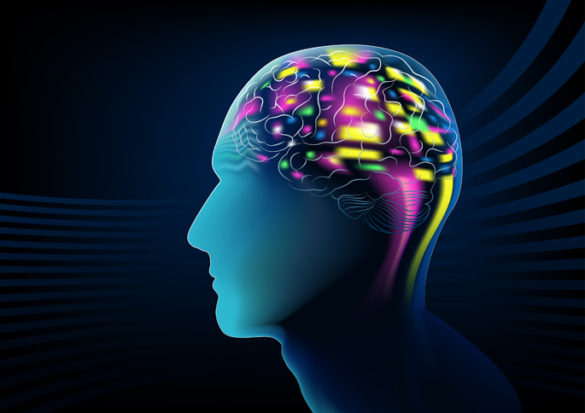
January 10, 2018
Children and adolescents needed for study on reading and learning.
Children and adolescents ages 9-14 are needed for a Vanderbilt University research study to better understand the relationship between reading and learning.
By VUMC News and Communications
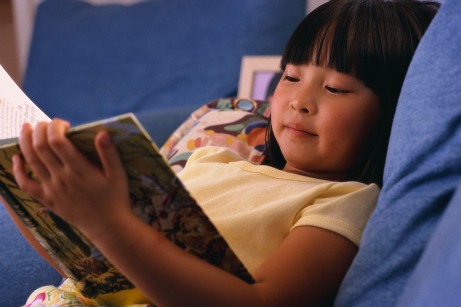
January 29, 2016
Parents and children needed for research study on reading development.
Participants are needed for a research study investigating reading development in children.
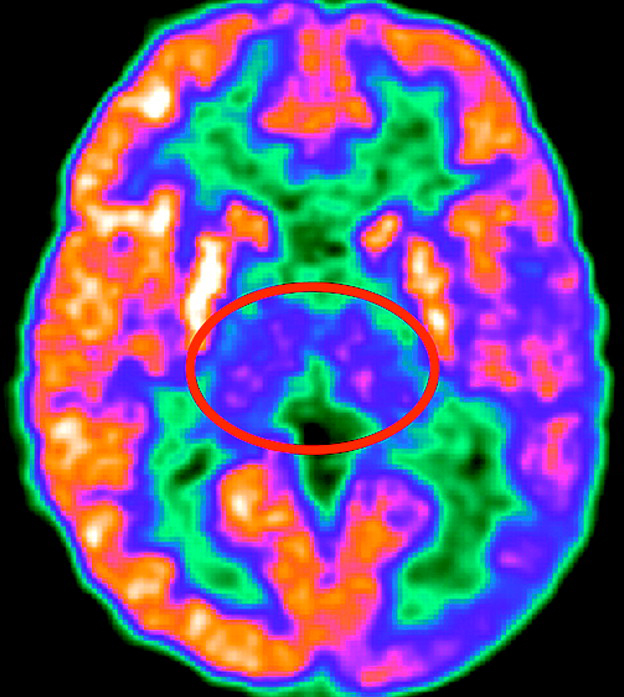
March 1, 2018
Research participants needed for neurofibromatosis study focused on reading difficulties.
The Vanderbilt Education and Brain Sciences Research Lab is seeking participants age 8 to 20 for a research study focusing on reading difficulties and neurofibromatosis.
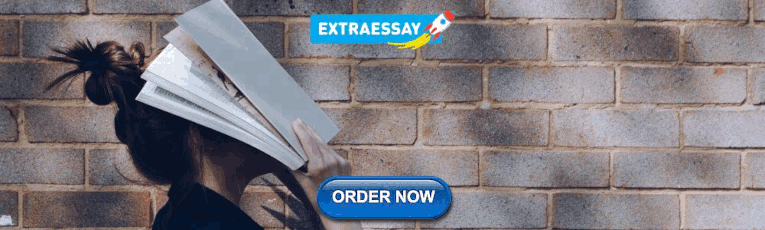
IMAGES
COMMENTS
The cumulative science linking stress to negative health outcomes is vast. Stress can affect health directly, through autonomic and neuroendocrine responses, but also indirectly, through changes in health behaviors. ... The studies reviewed in this article confirm that stress has an impact on multiple biological systems. Future work ought to ...
Abstract. Stressors have a major influence upon mood, our sense of well-being, behavior, and health. Acute stress responses in young, healthy individuals may be adaptive and typically do not impose a health burden. However, if the threat is unremitting, particularly in older or unhealthy individuals, the long-term effects of stressors can ...
Biological & social implications of the latest findings in chronic stress research. These studies significantly improve our understanding of the interactions between the nervous systems and peripheral tissues and organs, and how their alterations can cause illness . An important discovery is that if on one hand chronic stress can cause immune ...
1. Stress can manifest in the body. While stress can seem like a mental and emotional experience, its effects on the body are well-documented. We've all felt how short-term stress, like being startled, can make the heart race. And ongoing stress can accumulate, causing inflammation, wearing on the immune system, and overexposing the body to ...
The cumulative science linking stress to negative health outcomes is vast. Stress can affect health directly, through autonomic and neuroendocrine responses, but also indirectly, through changes in health behaviors. In this review, we present a brief overview of (a) why we should be interested in stress in the context of health; (b) the stress response and allostatic load; (c) some of the key ...
Abstract. Although stress strongly predicts life satisfaction, the psychosocial mechanisms underlying this association remain unclear. To investigate the possible mediating role of coping, we conducted a cross-sectional study that assessed youths' life stress levels, propensity to engage in three different coping styles (i.e., active coping, internal coping, & withdrawal), and life ...
2.1. Epidemiological and clinical stress research. Recent studies have revealed heightened levels of anxiety and depression during the ongoing pandemic, with specific factors such as social or lower economic resources influencing stress perception (Bueno-Notivol et al., 2021; Ettman et al., 2020; Kowal et al., 2020).
Stress is recognized as an important issue in basic and clinical neuroscience research, based upon the founding historical studies by Walter Canon and Hans Selye in the past century, when the concept of stress emerged in a biological and adaptive perspective.
Academic stress may be the single most dominant stress factor that affects the mental well-being of college students. Some groups of students may experience more stress than others, and the coronavirus disease 19 (COVID-19) pandemic could further complicate the stress response. We surveyed 843 colle …
Objective: This study sought to examine the relationship among the amount of stress, the perception that stress affects health, and health and mortality outcomes in a nationally representative sample of U.S. adults. Methods: Data from the 1998 National Health Interview Survey were linked to prospective National Death Index mortality data through 2006.
A 2017 meta-analysis looked at 24 studies—484 participants total—on heart rate variability (HRV) biofeedback and general stress and anxiety. The meta-analysis found that HRV biofeedback is helpful for reducing self-reported stress and anxiety, and the researchers saw it as a promising approach with further development of wearable devices ...
80% of US workers experience work stress because of ineffective company communications. 39% of North American employees report their workload the main source of the work stress. 49% of 18 - 24 year olds who report high levels of stress felt comparing themselves to others is a stressor. 71% of US adults with private health insurance say the ...
Skoluda et al. 2021 (in this issue) argue that hair as an important source of the stress hormone cortisol should not only be taken as a complementary stress biomarker by research staff, but that lay persons could be also trained to collect hair at the study participants' homes, thus increasing the ecological validity of studies incorporating ...
Here we focus on studies of affective responses to daily stressors, which constitute the bulk of existing intensive longitudinal studies of stress. Operationally, affective reactivity has been conceptualized as interindividual differences in the degree of intraindividual coupling of daily stress and affect (Sin et al., 2015). From a resilience ...
Academic-related stress and mental health. Previous research indicates that self-reported stress is associated with the presentation of anxious states ... A multi-method exploratory study of stress, coping, and substance use among high school youth in private schools. Frontiers in Psychology, 6, ARTN 1028. Retrieved from <Go to ISI>://WOS ...
A Qualitative Study of Stressors, Stress Symptoms, and Coping Mechanisms Among College Students Using Nominal Group Process . Helen Graf, PhD, Bridget Melton, EdD, and Stephen Gonzalez, MS . Abstract . Background: Stress is part of the college experience; however, how students deal with stress can greatly impact their behaviors and health status.
Purpose of review: Stress plays a central role in the onset and course of depression. However, only a subset of people who encounter stressful life events go on to experience a depressive episode. The current review highlights recent advances in understanding when, why, and for whom the stress-depression link occurs, and we identify avenues for future research.
Chronic stress has long been a focus of research for the National Center for Complementary and Integrative Health (NCCIH), and the interplay between the effects of stress on the mind and the body is an important area of research to me, personally. Many NCCIH-supported studies have looked at the potential role of complementary and integrative ...
University College Cork Nov 5 2024. A pioneering study has uncovered the vital role that gut microbiota plays in regulating stress responses by interacting with the body's circadian rhythms. The ...
Some studies have shown that stress has many effects on the human nervous system and can cause structural changes in different parts of the brain (Lupien et al., 2009 [65]). Chronic stress can lead to atrophy of the brain mass and decrease its weight (Sarahian et al., 2014 [100]).
Mental Health. Street trees can help reduce the need for antidepressants among city dwellers, according to new research. Its findings back up studies that indicate time spent among foliage and in nature can help relieve a number of stress-related conditions. Socio-economic inequalities often correlate with inequalities in tree distribution.
In the Stress and Early Adversity (SEA) Lab at Vanderbilt University, director Kathryn L. Humphreys and her team study how children's experiences are associated with development. The ABC Study is a research project that aims to understand how children grow and develop through their daily experiences and relationships.