What Is Thermal Pollution? Causes, Impact, and Mitigation
A growing concern, thermal pollution can disrupt natural systems.
Autumn is an independent journalist and educator who writes about climate, wildlife, biodiversity, and environmental justice and policy.
:max_bytes(150000):strip_icc():format(webp)/Autmn-spann-7bad790dc9394a99a98864432860767a.jpg)
- Columbia University Graduate School of Journalism
- University of California, Santa Cruz
- Western New Mexico University
:max_bytes(150000):strip_icc():format(webp)/ScreenShot2021-04-07at1.57.45PM-bcef177316c94cdf998457c694cce6d5.png)
- University of Tennessee
Jeff Swensen/ Getty Images
- Planet Earth
- Climate Crisis
- Recycling & Waste
- Natural Disasters
- Transportation
Thermal pollution is a rapid change in temperature in a natural body of water. This pollution is most often caused by heated discharge from an industrial facility or another human activity. Thermal pollution can result in disruptions in natural systems and stress, disease, or even death for affected organisms.
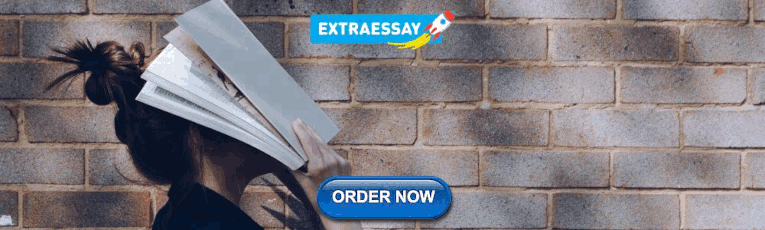
Causes of Thermal Pollution
Natural phenomena such as wildfires, volcanoes, and underwater thermal vents can cause thermal pollution. However, it is more often the result of an industrial process or facility using large amounts of water from a natural source and releasing heated wastewater.
Power Plants and Industrial Facilities
Thermoelectric power plants fueled by coal, natural gas, nuclear, or biomass and other waste products constitute significant causes of thermal pollution. Power plants are typically built next to a river, lake, or ocean, which provide a steady supply of water. This gets converted to steam that drives turbines to generate electricity. Water is also used to cool machinery, which becomes very hot. The water absorbs heat, and what doesn't evaporate is typically discharged back to its source.
In addition to power plants, other industrial facilities—such as petroleum refineries, pulp and paper mills, chemical plants, and steel mills—contribute to thermal pollution. They also use water to cool machinery and discharge water at elevated temperatures.
This process of sucking in water from a lake, ocean, or river for industrial purposes and then releasing heated water back to its source is called once-through cooling. It has long been known to adversely affect aquatic and marine environments. Because of once-through cooling, fish and larvae that get trapped against intake screens are killed, and habitats are altered due to the discharge of warmer, often polluted water.
Desalination Plants
tanukiphoto / Getty Images
Desalination plants also utilize once-through cooling. More than half of the seawater used in desalination gets flushed back into the ocean as wastewater, often at an elevated temperature.
In some parts of the world, desalination plants are clustered together, pouring massive amounts of heated, briny wastewater into shallow coastal regions. This can raise seawater temperature and salinity significantly.
Wastewater, Erosion, and Deforestation
Not all wastewater gets treated before it is released into water bodies. Untreated sewage, urban stormwater, and agricultural runoff can create thermal pollution in nearby water sources as the runoff is often warmer than the streams, lakes, or ocean they flow into.
Human land-use changes cause thermal pollution as well. Deforestation for timber harvesting or clearing land for crops and livestock grazing provokes erosion alongside rivers and streams, which leads to wider, shallower stream beds more prone to warming. In addition, clearing trees and vegetation from lakeshores and riverbanks creates more sun exposure, provoking water warming.
Impacts of Thermal Pollution
When heat moves rapidly into a water source, it generates direct and indirect environmental effects. Aquatic organisms can be highly sensitive to even small changes in water temperature. Some are unable to cope, suffering stress, disease, and even death. When populations of fish and other organisms decline, it may provoke ripple effects through the ecosystem.
Thermal pollution also alters oxygen levels. The introduction of warmer water causes oxygen levels to drop, affecting aquatic life. Warmer water encourages the growth of algae, which absorb sunlight and cause further warming. These effects are often intensified if the discharge water contains a lot of nutrients , as is the case with agricultural runoff and untreated sewage. Warmer temperatures can increase aquatic organisms' vulnerability to chemicals present in these wastewaters, such as ammonia, heavy metals, and pesticides. Together, thermal pollution and nutrient loading can cause hypoxic “ dead zones ,” with very low oxygen levels.
Examples of Thermal Pollution
In a 2016 global analysis of thermal pollution in rivers, the Mississippi River topped the list. Sixty-two percent of the river's heat emissions came from coal-fired power plants, and 28% from nuclear power generation. Other thermal pollution sources included agricultural runoff and wastewater. Europe's Rhine River also suffered significant impacts from power plant emissions, most notably from nuclear plants.
Water-scarce countries, particularly in the Middle East, have turned to desalination as a means of bolstering water security in the face of drought and climate change. A 2020 study of desalination plants in Ashkelon and Hadera along Israel's Mediterranean coast found that the mixing of cooling water to dilute briny wastewater created a heated plume 25% warmer than the natural seawater temperature, placing stress on local benthic organisms near the seafloor.
At California's last operating nuclear power plant, Diablo Canyon near San Luis Obispo, opponents have long raised concerns about the ecosystem impacts of the plant discharging millions of gallons of heated seawater each day back to the ocean. In 2021, PG&E, the utility that owns Diablo Canyon, reached a $5.9 million settlement agreement with the state over violation of permits meant to limit thermal pollution.
Mitigation of Thermal Pollution
Mimi Ditchie Photography / Getty Images
Thermal pollution is a growing concern, especially with climate change exacerbating increases in water temperature from power plants, industry, agriculture, and other human sources. As of 2013, about a third of total power generation in the U.S. came from power plants that used once-through cooling. This is typical of older energy generation facilities.
A 2016 study found that half of the global freshwater heat emissions come from nuclear and coal plants from the 1970s and 1980s. Some older power plants that utilize once-through cooling are shutting down as aging equipment and increasing restrictions on air and water pollution, water consumption, and thermal discharge decrease profitability and increase liability.
In the U.S., thermal pollution is regulated by the federal Clean Water Act, which requires states to set limits for thermal discharges from power plants to protect aquatic organisms and wildlife. Power plants must meet temperature discharge standards in order to qualify for the permits, or alternatively, prove that the discharge temperature has no adverse environmental effects. However, this doesn’t always happen in practice.
There is now a shift away from once-through cooling—not only because of thermal pollution but because of the overall strain that water-intensive processes place on aquatic and marine life, as well as increasingly scarce water resources. In 2010, California enacted a regulation to phase out once-through cooling at coastal power plants that use ocean water.
Existing and emerging technologies provide other means of mitigating thermal pollution from power plants and other industrial sources. These include reducing the amount of water released by such facilities and capturing heated wastewater for other purposes, like desalination, to reduce discharges.
Rosen, Marc A., et al. " Evaluating the Thermal Pollution Caused by Wastewaters Discharged from a Chain of Coal-Fired Power Plants along a River ." Sustainability , vol. 7, no. 5, 2015, pp. 5920-5943., doi:10.3390/su7055920
" Thermoelectric Power Water Use ." United States Geological Survey .
Madden, N., et al. " Thermal Effluent from the Power Sector: An Analysis of Once-Through Cooling System Impacts on Surface Water Temperature ." Environmental Research Letters , vol. 8, no. 3, 2013, pp. 035006., doi:10.1088/1748-9326/8/3/035006
Jones, Edward, et al. " The State of Desalination and Brine Production: A Global Outlook ." Science of the Total Environment , vol. 657, 2019, pp. 1343-1356., doi:10.1016/j.scitotenv.2018.12.076
Omerspahic, Mustafa, et al. "Characteristics of Desalination Brine and Its Impacts on Marine Chemistry and Health, with Emphasis on the Persian/Arabian Gulf: A Review." Frontiers in Marine Science , vol. 9, 2022,, doi:10.3389/fmars.2022.845113
" Nonpoint Source: Urban Areas ." Environmental Protection Agency .
Jane, S.F., et al. " Widespread Deoxygenation of Temperate Lakes ." Nature , vol. 594, 2021, pp. 66-70., doi:10.1038/s41586-021-03550-y
" Climate Change and Harmful Algal Blooms ." Environmental Protection Agency .
" The Effects: Dead Zones and Harmful Algal Blooms ." Environmental Protection Agency .
Raptis, C.E., et al. " Global Thermal Pollution of Rivers from Thermoelectric Power Plants ." Environmental Research Letters , vol. 11, no. 10, 2016, pp. 104011., doi:10.1088/1748-9326/11/10/104011
Kenigsberg, Chen, et al. " The Effects of Long-Term Brine Discharge from Desalination Plants on Benthic Foraminifera ." PLOS ONE , vol. 15, no. 1, 2020., doi:10.1371/journal.pone.0227589
" PG&E Pays $5.9 Million Settlement for Once-Through Cooling Water Discharges from Diablo Canyon Nuclear Power Plant ." California Water Boards , 2021.
" Once-Through Cooling Phase Out ." California Energy Commission , 2019.
- What Is Desalination? How Does It Impact the Environment?
- What Is Water Pollution? Sources, Environmental Impacts, Mitigation
- What Is Nutrient Pollution? Causes, Impacts, and Mitigation
- What Are Fugitive Emissions? Definition and Impact
- What Is BPA? Definition and Environmental Impact
- What Is Soil Pollution? Environmental Impacts and Mitigation
- A Beginner’s Guide to Rainwater Harvesting
- Why the Great Barrier Reef Is in Danger
- How Does Mercury Get in Fish?
- How Does BPA Affect Animals and the Environment?
- 10 Ways to Stop Being a Water Waster
- What Is Geothermal Energy? Definition, Examples, and How It Works
- What Are PFAS? Definition, Sources, and Health Risks
- 10 Examples of Why the Superfund Program Matters
- Is Acrylic Clothing Sustainable? How It's Made & Environmental Impacts
- Top 5 Ways Water Gets Polluted
IEEE Account
- Change Username/Password
- Update Address
Purchase Details
- Payment Options
- Order History
- View Purchased Documents
Profile Information
- Communications Preferences
- Profession and Education
- Technical Interests
- US & Canada: +1 800 678 4333
- Worldwide: +1 732 981 0060
- Contact & Support
- About IEEE Xplore
- Accessibility
- Terms of Use
- Nondiscrimination Policy
- Privacy & Opting Out of Cookies
A not-for-profit organization, IEEE is the world's largest technical professional organization dedicated to advancing technology for the benefit of humanity. © Copyright 2024 IEEE - All rights reserved. Use of this web site signifies your agreement to the terms and conditions.
Academia.edu no longer supports Internet Explorer.
To browse Academia.edu and the wider internet faster and more securely, please take a few seconds to upgrade your browser .
Enter the email address you signed up with and we'll email you a reset link.
- We're Hiring!
- Help Center
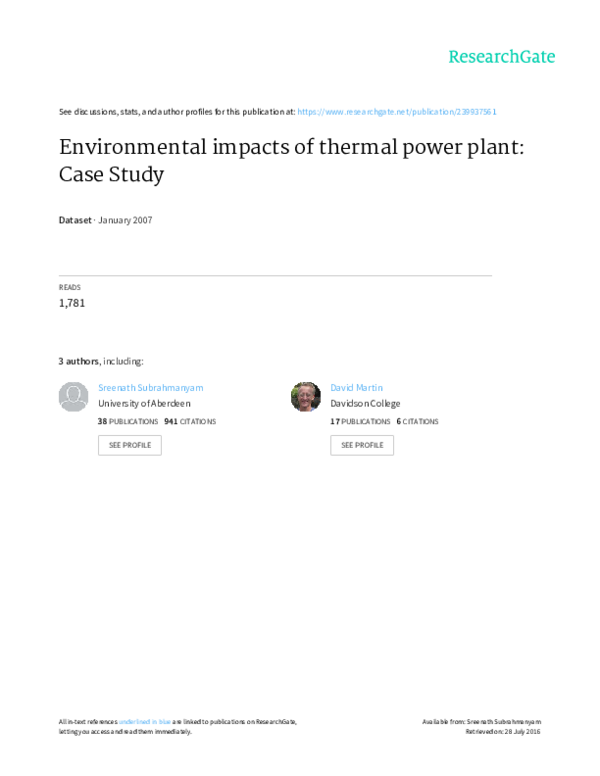
Environmental impacts of thermal power plant: Case Study

Related Papers
Prabir Datta
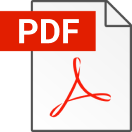
Dr Nethajimariappan Velu Ethirajan
Arun, P. R. (2007) An Environmental appraisal of Jaigad and its environs, the proposed site for the 1200 MW mega thermal Power plant. Green Alternatives Mumbai; -80 p
Sagar Dhara
India’s 102,000 MW commercial power generating capacity, 69% by thermal power, is the second largest in Asia. India’s plans to increase this capacity, largely by thermal power, is one of the world’s largest power augmentation programmes. Over the next half-century, Indian thermal power plants may pose a bigger risk to biodiversity in the Indian subcontinent than all other anthropogenic interferences together did in the past. Sulphur dioxide emissions from thermal power plants will pose the primary risk; and the siting of new power plants closer to river sources, and therefore forests, will pose a secondary risk. Sulphur dioxide emissions, which are currently 6.5 million tonnes, are estimated to grow at 5.5% per annum to 19 million tonnes by 2020. Nitrogen dioxide emissions, currently 10 million tonnes, are estimated to grow at an even faster rate. The synergistic effect of both these acidic gases will put 35 million Ha, or 55% of India’s best forests in the Western and Eastern Ghats, the Himalayas, the Northeast and the Andaman Islands at risk of forest dieback as the soils of these forests have a low buffering capacity and are, therefore sensitive to acidic gas depositions. To minimize coal transport costs, thermal power plants have largely been located at coalmine pitheads in the major coal belts of India in North-central and East India. However, these areas are also water-stressed. Thermal power plants require large quantities of water. In their search for water, new thermal power plants will locate closer to river sources first close to the Eastern Ghats, then the Himalayas and the Western Ghats. These three hill systems are also forested. As other downstream industry locate close to power plants, the growth centres that are nucleated close to the forests will put them and the biodiversity they host under pressure. Short-term, medium-term and long-term measures are required to tackle these new threats to biodiversity. The short-term measures include: studying the impact of thermal power on biodiversity, setting up a monitoring mechanism for acidic depositions and their impacts, encouraging the development of green lungs and using bioindicator plant species to monitor pollution levels around thermal power stations, training bystander populations residing around power plants to monitor the plants, including the cost of externalities in the price of power, formulating environment-friendly power plant siting rules and preparing a toxic release inventory which will put in public domain. The medium-term measures include: adopting an energy and environment policy, instituting a carbon and sulphur tax, having the signatory nations to the Malé Declaration sign a binding protocol on sulphur emission caps, setting emission standards for power plants, encouraging the use of clean fuels, encouraging emissions control technologies and combined cycle plants, initiating demand-side management by encouraging low energy consuming devices, instituting Pollution Prevention Boards and decentralizing the grant of consent for operations to local self governments, minimizing transmission and distribution losses and encouraging the setting up of renewable energy capacities.
International Journal of Current Research
Industrialization poses a great threat to human kind by the way of generating deleterious products followed through rapid urbanization and population growth activities. Different methods of waste management emit a large number of substances, most in small quantities and at extremely low levels. Land filling was the one of the method used for the discarding of industrial waste in Chennai, and the majority of the landfill sites are open dumping areas, which causes severe environmental and social threats. As a result, the hazardous waste generation has tremendously increased. Therefore an environmental assessment was the need of the hour. A field based study was taken up on five land fill sites namely the Madarpakkam, Sathyavedu village, Menallur village, Nemalur village, Pondavkkam village that were within the 10km buffer zone of the landfill site Gummidipoondi in Tamil Nadu, India and the sites were assessed for environmental parameters on air such as SO2, NOx and SPM, ground water and soil on physical, chemical and associated factors. Overall results has showed that air, soil and water quality were within the prescribed limits of the International standards that could be due to buffer distance of 10km from the core region. A transect survey of environmental parameters would have been better to identify environmental impacts of disposal methods could be considered by decision makers in future to suggest the most environmentally and economic method to the policy planners.
BHAT MOHD SKINDER
Ghasem Najafpour
Carbon dioxide causes green house effect and has been considered as a pollutant source forsafe environment. Since combustion of fossil fuel may create tremendous amount of carbon dioxide,detecting any pollutant sources would be important to eliminate the origin of pollution sources.Evaluation of smoke dispersion generated by the power plant utilizing fossil fuel is the objective of thispaper. The concentration of NOx and SOx in the soil till a distance of 15 km from Neka power plant,have been analyzed. The maximum concentrations of SOx and NOx in the distance of 2.5 to 4 km fromthe power plant were 0.13 and 0.36 ppm, respectively. Replacement of fossil fuel (fuel no. 6, Mazout) bynatural gas may increase NOx concentration in the atmosphere; however the use of natural gas wasrecommend by environmental protection agencies as clean fuel. Use of natural gas may not be an actualsolution for prevention of NOx pollution.
Ephraim Musa
Loading Preview
Sorry, preview is currently unavailable. You can download the paper by clicking the button above.
RELATED PAPERS
International Journal of Engineering Research and Technology (IJERT)
IJERT Journal
pratiksha deshmukh
Atmospheric Environment
Shekar Reddy
Tselmuun Chinzorig
Priyabrata Roy
Mircea Georgescu
Dr. Manoj Tiwari
ambuj gupta
Voice of Environment Newsletter
Moharana Choudhury
Pramod Rajak
International Journal of Current Microbiology and Applied Sciences
Shakeel Khan
Sachin Bagul
mitraj gohil
Nargis Shopna
Dr. Chandra Shekhar Kapoor
ankur chaudhary
Andrzej Chmielewski
Michael Akinpelu
Debi Goenka
Journal of Earth Science & Climatic Change
Khurram Shahzad Baig
International Journal of Greenhouse Gas Control
Juan Adanez
Prince Bharti
maría rodriguez
Sergio Capareda
Sol Rodríguez Zerón
IAEME Publication
Dr.Shashidhar thhatikkonda
Greisly Marrugo
samir khureshi
Environmental Monitoring and Assessment
Muyeed Hasan
Environmental …
Liliana Rogozea , Mihaela Badea
International journal of energy research
Subhes Bhattacharyya
ADEWALE ODERINDE
- We're Hiring!
- Help Center
- Find new research papers in:
- Health Sciences
- Earth Sciences
- Cognitive Science
- Mathematics
- Computer Science
- Academia ©2024
Accessibility Links
- Skip to content
- Skip to search IOPscience
- Skip to Journals list
- Accessibility help
- Accessibility Help
Click here to close this panel.

Purpose-led Publishing is a coalition of three not-for-profit publishers in the field of physical sciences: AIP Publishing, the American Physical Society and IOP Publishing.
Together, as publishers that will always put purpose above profit, we have defined a set of industry standards that underpin high-quality, ethical scholarly communications.
We are proudly declaring that science is our only shareholder.
Thermal effluent from the power sector: an analysis of once-through cooling system impacts on surface water temperature
N Madden 1 , A Lewis 2 and M Davis 1
Published 3 July 2013 • © 2013 IOP Publishing Ltd Environmental Research Letters , Volume 8 , Number 3 Focus on Electricity, Water and Climate Connections Citation N Madden et al 2013 Environ. Res. Lett. 8 035006 DOI 10.1088/1748-9326/8/3/035006
Article metrics
26147 Total downloads
Share this article
Author e-mails.
Author affiliations
1 Union of Concerned Scientists, Cambridge, MA 02238-3780, USA
2 Duke University, 2138 Campus Drive, Durham, NC 27705, USA
- Received 31 December 2012
- Accepted 22 April 2013
- Published 3 July 2013
Buy this article in print
In this study, we review federal datasets to assess the impacts of once-through power plant cooling systems on summer freshwater temperatures in the United States from 1996 to 2005. We find that maximum reported temperature discharges averaged 37 ° C (1996–2005) and were 9.5 ° C (1996–2000) to 10 ° C (2001–2005) higher than maximum reported intake temperatures during the summer. More than half of all power plant cooling systems report maximum temperature discharges that exceed 32 ° C and increase water temperatures enough to potentially impact aquatic life. However, current federal data on thermal discharges from power plants are insufficient to adequately assess their impact on in stream temperatures, or their subsequent effects on aquatic ecosystems and biodiversity. A preliminary analysis indicates that certain watersheds, primarily in the Southeastern and Midwestern United States, are good candidates for more focused study of power plant temperature impacts.
Export citation and abstract BibTeX RIS
Content from this work may be used under the terms of the Creative Commons Attribution 3.0 licence . Any further distribution of this work must maintain attribution to the author(s) and the title of the work, journal citation and DOI.
1. Introduction
When river temperatures heat up during summer periods of low flow and high temperatures, staying within defined regulatory temperature limits can require power plants to shut down or curtail their power generation (Kimmell and Veil 2009 , Sovacool and Sovacool 2009 , Van Vliet et al 2012 ). Recent events such as elevated heat and drought across the United States in the summer of 2012 have required thermoelectric power plants to curtail operations or seek variances on thermal discharge regulations (Eaton 2012 ). In addition, concerns have been expressed that increased river temperatures from climate change could constrain energy production in the future (USGCRP 2009 ). On the federal level, it has been proposed that the emergency authority of the US Department of Energy (DOE) should be expanded to override environmental regulations on power plants during periods when grid reliability may be at risk (HR 4273 2012 ). However, the impacts of increased temperatures on electricity production and grid reliability have not been well studied (Mideska and Kallbekken 2010 , Schaeffer et al 2012 ).
A handful of recent studies suggest that increased river temperatures from climate change could impact grid reliability by taking electricity production off-line during summer periods when high temperatures coincide with peak electricity production. The majority of these studies have focused on Europe (Koch and Vögele 2009 , Förster and Lilliestam 2009 , Koch et al 2011 ), although a recent study has been extended to the United States, finding that 4%–16% of the electricity grid could be impacted by increased temperatures by 2050 under various climate change scenarios (Van Vliet et al 2012 ).
In this study, we review federal datasets documenting water temperature at intakes and discharges from power plants during the summer in the United States between 1996 and 2005. Our study focuses on plants with once-through cooling systems, which withdraw and discharge the largest volumes of water compared to other thermoelectric power plant cooling technologies (Macknick et al 2012 ). These systems also have the largest associated heat load, and are the most likely to come into conflict with regulatory limits on thermal discharges (Koch and Vögele 2009 ). We examine the quality and completeness of this dataset for national assessment. In addition, we use spatial analysis to determine watersheds where more focused studies of thermal pollution may be appropriate. A case study of one of these basins, the Upper Catawba River, illustrates some of the issues with regulatory management of power plant thermal effluent.
2. Background
2.1. once-through cooling and reporting of temperature data.
In the United States, there are currently 425 power plants that use once-through cooling, comprising just under a third of total electricity generation (UCS 2012 ). Once-through cooling systems circulate water through a plant a single time to provide cooling during generation. These systems require large volumes of water, which is extracted from rivers, lakes, or the ocean, on the order of 20 000–60 000 gallon MWh −1 of electricity produced (Macknick et al 2012 ). This water is cycled through the cooling system and then is discharged, transferring waste heat from the power plant into the discharge body of water. The discharge water body is usually also the source of the cooling water, causing a local temperature increase. Typical discharge temperatures from once-through cooling systems are 8 to 12 °C above intake temperatures, with some systems raising temperatures as much as 15 °C (Langford 2001 ). This heated discharge water then mixes with the receiving water body, with temperature impacts dissipating downstream through radiant transfer or evaporation into the atmosphere (Edinger et al 1968 ). Variability between power plant discharge temperatures can be due to differences in local climates, plant efficiencies, or volumes of water withdrawn for cooling. Under the Federal Energy Administration Act of 1974 (Public Law 93-275), the US Department of Energy (DOE) energy information administration (EIA) is required to collect data on the operations, management, and ownership of electricity generators and distribution companies in the United States. Within this process, the EIA collects environmental data such as water withdrawals, discharges and associated temperatures at the request of the US Environmental Protection Agency. This data is nominally required for all thermoelectric power plants with nameplate capacities greater than 100 MW, although reporting is voluntary (Wirman 2012 ). Prior to 2010, only the maximum intake and discharge temperatures were collected for the summer and winter months with peak electrical generation (EIA Form 767).
2.2. Aquatic organism impacts
Due to the biological sensitivity of many aquatic organisms to water temperature, temperature increases caused by power plant discharges may have multiple impacts on aquatic ecosystems (Langford 2001 , De Vries et al 2008 , Hester and Doyle 2011 ). Aquatic organisms are highly dependent on specific thermal conditions in aquatic environments; water temperatures above or below optimal thermal regimes can cause stress or even death (Beitinger et al 1999 , Caissie 2006 ). It has been concretely demonstrated that fish cannot survive at temperatures above a critical thermal maximum for a certain duration. This critical maximum rarely occurs naturally outside a power plant's effluent stream, and is high enough to kill many entrained larvae and animals (Beitinger et al 1999 , Kelso and Milburn 1979 ). For example, a suitable habitat for rainbow trout ( Oncorhynchus mykiss ) has an optimal temperature range of 13–15 °C, with the lethal maximum of 24.3 °C (Bear et al 2007 ). Temperatures above the critical thermal maximum create uninhabitable conditions for the rainbow trout. Higher stream temperatures also decrease oxygen solubility while increasing respiration rates, both of which reduce the availability of dissolved oxygen. Lowered oxygen in aquatic environments can limit the distribution of fish and macro-invertebrates, reduce growth rates, and alter nutrient and carbon cycling (Langford 1990 ). Elevated temperatures can also stress organisms, increase the toxicity of chemicals, and inhibit biological processes. One review of 151 toxicology studies found that high temperatures typically increase aquatic organism vulnerability to chemicals such as ammonia, heavy metals, and pesticides (Heugens et al 2001 ). Another review of 48 studies found that on average, a 7 °C change in temperature (Δ T ) reduced biological processes such as growth, development, and reproduction for aquatic organisms by 50%, with a 10% reduction in biological processes occurring with a temperature change of only 1 °C (Hester and Doyle 2011 ). While the thermal impacts of discharges dissipate downstream from power plants, the magnitude of temperature increases (Δ T ) in effluent near the discharge point can be high enough to potentially impact aquatic life.
2.3. Regulation of thermal discharges
In the United States, discharges of thermal effluent are regulated by section 316(a) of the Clean Water Act, which requires states to set limits for power plant thermal effluent in order to 'assure the protection and propagation of a balanced, indigenous population of shellfish, fish, and wildlife in and on that body of water' (CWA 1972 ). The primary method of enforcing this law is through the National Pollution Discharge Elimination System (NPDES) permit program, which regulates point sources that discharge pollutants into waters of the United States. The NPDES program is usually administered by state environmental agencies to meet water quality standards, and is overseen at the federal level by the United States Environmental Protection Agency (EPA). In order to obtain permits to discharge heated water, power plants are either required to meet water quality temperature standards, or to obtain a temperature variance, by proving their thermal effluent does not have adverse environmental impacts. Water quality standards vary by state, but typically require surface water to remain under 32 °C (see supplementary data for further information available at stacks.iop.org/ERL/8/035006/mmedia ). The duration of NPDES permits is limited by the Clean Water Act to five years, after which the permit must be renewed with the appropriate regulatory agency (EPA 2012 ). In practice, the stringency in enforcing the Clean Water Act varies on a case-to-case basis, and limits are not always adequately set or enforced to protect aquatic life (Duhigg 2009 , GAO 2009 ). In 2011, the EPA found the North Carolina Division of Water Quality had issued temperature variances to power plants that did not provide enough information to determine whether the revised thermal limits harmed aquatic life (EPA 2011a ).
3.1. Reported temperatures from once-through cooling systems
Datasets for power plant characteristics, summer peak intake temperature ( T pin ), and outflow water temperature ( T pout ) from once-through freshwater cooling systems were compiled from the US Energy Information Administration form 767 for the years 1996–2005 (EIA 1996–2005 ). The data required that maximum peak temperature be collected at the intake station and the outflow stations for each cooling system during the winter and summer season. Plants were allowed to estimate the water temperature when readings were unavailable and collected only one data point for each season over the given time period (EIA 2005 ). The temperature provided indicates the maximum cooling water temperature at the intake and outflow for the 'peak load month', the month of greatest electrical generation at the plant. The seasons are defined as winter, from October to March and summer from April to September. Our study focuses on temperature data from the summer season, when electricity generation peaks and causes the largest associated thermal impacts.
This data was vetted to select for power plants with primary cooling systems designated by the EIA as once-through and using freshwater, with five years of complete, non-zero records for the periods 1996–2000 and 2001–2005. The time periods 1996–2000 and 2001–2005 were analyzed separately as the EIA did not require nuclear power plants to report to the EIA after 2000. A number of power plants did not report their cooling technologies to the EIA, so we compared the plants in our dataset to a more complete and detailed database of United States power plants in 2008 (Averyt et al 2013 , UCS 2012 ) to determine what percentage of total fleet of once-through cooled plants were reporting temperature data. The temperature data was averaged over 1996–2000 ( n = 418) and 2001–2005 ( n = 403) and converted from Fahrenheit to Celsius. We also calculated Δ T p , or the difference between peak intake and discharge temperatures, where Δ T p = T pout − T pin . We used histogram analysis to evaluate the distribution of Δ T p , T pout , and T pin , and gauge the normality of the data.
3.2. Spatial analysis of HUC-8 basins with potential ecological impacts
We outlined potential impacts of thermal discharges from power plants on aquatic species by comparing two indicators: peak discharge temperatures against counts of threatened and endangered aquatic obligate species at the watershed level. Along with temperature, power plants pose a number of adverse impacts to aquatic species, including impingement (when organisms are trapped against intake screens), entrainment (when organisms get drawn through the plant cooling system), and chemical pollution. However peak discharge temperatures are both reported nationally and regulated, making it an appropriate indicator for broad-scale analysis. While discharge temperatures do not necessarily affect endangered species counts, high discharge temperatures can lower the concentration of dissolved oxygen, inhibit biological processes, and stress sensitive aquatic species by exceeding thermal tolerances (Cairns et al 1975 , Langford 1990 , Heugens et al 2001 , Hester and Doyle 2011 ). To protect aquatic ecosystems, states limit temperatures through their water quality standards, typically to 32 °C or lower (see supplementary data for further information available at stacks.iop.org/ERL/8/035006/mmedia ).
Aquatic obligate species are defined as any species that belong to any taxonomic group that can be classified as aquatic in the absence of habitat data. These data were obtained from a dataset compiled from state-level natural heritage programs (NatureServe 2011 ) mapped at the USGS Hydrologic Unit Code-8 (HUC-8) level using a digitization of USGS watershed boundaries (Steves and Nebert 1994 ). Power plant locations were obtained using latitude and longitudes from the Union of Concerned Scientists Energy-Water Database (UCS 2012 ). At the location of each power plant, average T pout (2001–2005) was mapped and projected onto HUC-8 watersheds with counts of aquatic obligate species that were either G1 (critically imperiled), G2 (imperiled) or federal status endangered species under the US Endangered Species Act (NatureServe 2011 ). We also identified watersheds where aquatic ecosystems with high aquatic biodiversity were potentially at risk from high discharge temperatures, defined as T pout ≥ 32 °C. As a proxy for high aquatic biodiversity, we selected the 220 HUC-8 watersheds that had more than 10 G1, G2 or endangered aquatic obligate species, out of 1963 watersheds with data. To scope areas for more focused study, we identified and mapped watersheds that had both high discharge temperature and high aquatic biodiversity.
3.3. Case study of the Upper Catawba watershed
From the results of the spatial analysis, we selected one watershed, the Upper Catawba, for a more focused case study. The Upper Catawba was designated as a watershed where high biodiversity was potentially at risk from thermal pollution, as power plants in the Upper Catawba basin had peak discharge temperatures that exceeded regulatory temperature limits during the summer. The Upper Catawba also illustrates some of the regulatory issues that occur with enforcement of the Clean Water Act in regards to thermal effluent.
The Upper Catawba River basin spans both North and South Carolina, supplies millions of residents with drinking water and recreational areas, and provides five major (>100 MW) thermoelectric power plants with cooling water, generating over 30% of North Carolina's electricity (Milazi 2009 , UCS 2012 ). Of these 5 power plants, 3 are coal-fired, 2 are nuclear, and all 5 utilize once-through cooling systems. The Upper Catawba provides aquatic habitats for numerous organisms, including thirty-nine separate species of fish (SCDNR 2008 , NatureServe 2011 ).
In order to analyze the thermal impacts of the 5 power plants along this river, data were distilled from the EIA temperature dataset compiled from 1996–2005. Additionally, NPDES permits were obtained for each plant (NCDENR 2005 , SCDHEC 2005 , NCDENR 2011a , 2011b , 2011c , 2011d ) in order to compare reported data with state and federal regulations for power plant thermal effluent (NCDENR 2007 , SCDHEC 2008 ).
4.1. Reported temperatures from once-through cooling systems
As table 1 indicates, average Δ T p is between 9.5° and 10 °C. While this was calculated specifically for peak discharge temperatures in the summer, these values are generally comparable to Δ T values found by other studies (Langford 2001 , Hester and Doyle 2011 ). In 1996–2000, 233 out of 418, or 56% of cooling systems had T pout values that were above the benchmark temperature of 32 °C in all years. In 2001–2005, 230 out of 403, or 57% of cooling systems had peak discharge temperatures that were above the benchmark temperature of 32 °C in all years.
Table 1. Average and standard deviations for peak intake, peak discharge, and Δ T during summer months, 1996–2000 and 2001–2005.
Years | Average (° C) | Average (° C) | Average Δ (° C) | |
---|---|---|---|---|
1996–2000 | 418 | 27° (±5.9°) | 37° (±6.8°) | 9.5° (±4.8°) |
2001–2005 | 403 | 27° (±5.8°) | 37° (±6.5°) | 10° (±5.0°) |
We also looked at how completely plants reported temperature data, both on the scale of cooling systems and individual plants. Of the cooling systems that reported temperatures, we found that 68% of individual cooling systems and 61% of all power plants had complete, non-zero temperature records for the years in our study (see supplementary data for further information available at stacks.iop.org/ERL/8/035006/mmedia ). Nuclear plants had the most incomplete temperature records. From 1996–2000, 66% of nuclear power plants reported complete, non-zero intake and discharge temperatures, and nuclear power plants did not report any temperature data to the EIA after 2000.
4.2. Spatial analysis of HUC-8 basins with potential ecological impacts
Mapping discharge temperatures against watershed counts of G1 and G2 aquatic obligate species (as an indicator of high aquatic biodiversity) allowed us to identify watersheds that where further analysis of potential ecological risks may be useful. Figure 1 shows that the majority of once-through freshwater cooling systems are located in the eastern half of the United States (GAO 2009 ). In the period 2001–2005, 220 HUC-8 watersheds had 10 or G1 and G2 aquatic obligate species and 136 HUC-8 watersheds had average T pout equal to or greater than 32 °C. 33 basins had both high temperature discharges and high biodiversity, primarily located in the South-Atlantic Gulf, Tennessee, and Ohio River Watershed Resource Regions (figure 2 ). We selected one of the 33 basins, the Upper Catawba River Basin, for further analysis. The Upper Catawba watershed had 11 counts of G1+G2 aquatic obligate species and an average T pout of 37 °C in 2001–2005.
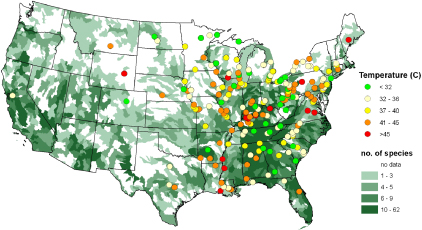
Figure 1. Average T pout (°C) from once-through cooled plants in 2001–2005 mapped against counts of G1+G2 aquatic obligate species (NatureServe 2011 ).
Download figure:
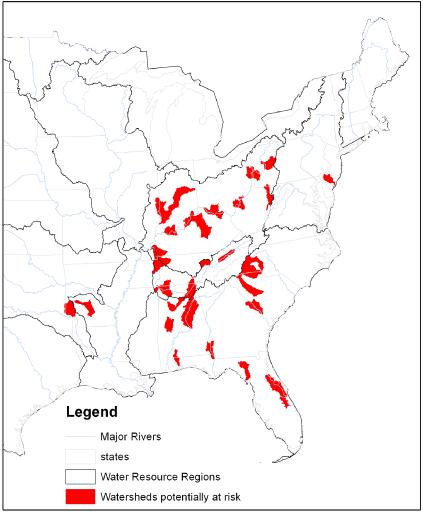
Figure 2. There are 33 HUC-8 watersheds (Steves and Nebert 1994 ) where high aquatic biodiversity (G1+G2 aquatic obligates ≥10) is potentially at risk from high temperature discharges (avg. T pout ≥ 32 °C, 2001–2005) from once-through cooling systems.
4.3. Case study of temperature reporting on the Upper Catawba watershed
While EIA temperature data was available for all five major power plants from 1996 to 2000, the two nuclear plants (Catawba Nuclear Station and McGuire Nuclear Station) were not required to report temperature data from 2001–2005 (EIA 2010 ). Temperature data was reported only as summer peak intake and discharge temperatures for the highest month of electrical generation, disguising diurnal variations, sampling rates, and the frequency of thermal discharges above thermal limits. All power plants with available data reported discharging water that exceeded state limits on Δ T and maximum discharge temperatures during the summer (figure 3 ). However, their NPDES permits revealed that all five power plants had been granted thermal variances that allowed them to exceed state water quality limits, instead requiring power plants to keep thermal discharges below higher monthly averages and Δ T values (NCDENR 2005 , 2011b , 2011c , 2011d , SCDHEC 2005 ). These variances may not be sufficient for Clean Water Act compliance. In the summer of 2007, the combined effects of drought and high temperatures caused Duke Energy to scale back power production at the GG Allen Steam Station and the Riverbend Steam Station when the discharge temperatures at these two plants exceeded their permit limits (Beshears 2007 ). As part of an ongoing corrective action, the NPDES permits at the Marshall and McGuire power plants have been targeted for direct review by the EPA (EPA 2011b ).
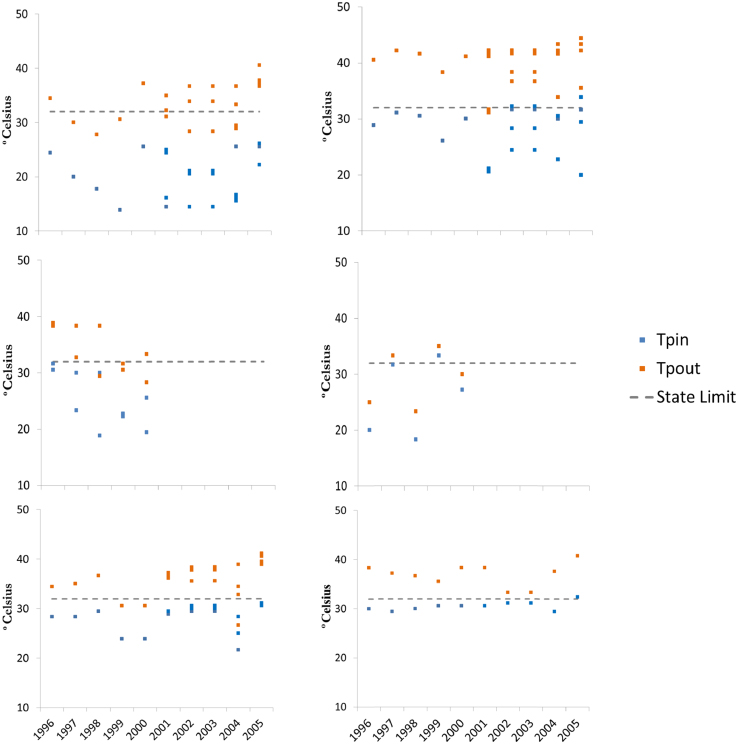
Figure 3. Summer peak intake and peak discharge temperatures (°C) from power plant cooling systems versus state temperature limits in the Upper Catawba River basin, 1996–2005.
5. Discussion
5.1. power plant thermal discharges are high enough to potentially impact aquatic life.
Our study found that once-through cooling plants discharged freshwater with peak temperatures of 9.5–10 °C above ambient stream temperatures during the summer from 1996–2005. Other studies have indicated that substantial aquatic ecosystem impacts can occur with temperature increases of 5 °C or higher (De Vries et al 2008 , Hester and Doyle 2011 ), which suggests that the peak temperature discharges during the summer could affect aquatic ecosystems and the organisms that inhabit them. In addition, it appears that once-through cooling systems are currently facing ambient cooling water temperatures close to known regulatory limits and discharging waters that may exceed them. Our study indicated that high peak discharge temperatures most often intersected with the highest counts of imperiled aquatic obligate species in the South-Atlantic Gulf, Tennessee, and Ohio River basins, where aquatic biodiversity is generally high and once-through cooling is more common (figure 2 ). This corroborates the findings of Van Vliet et al ( 2012 ), which found that climate change will raise the likelihood of plants running into conflict with regulatory limits as ambient temperatures increase.
5.2. Current federal data is insufficient to assess the ecological impacts of thermal discharge
While thermal discharges from once-through cooling systems appear likely to impact aquatic ecosystems, the current datasets available from the EIA are insufficient to evaluate watershed-scale impacts to aquatic biodiversity and ecosystems. Given the inherent problems with the availability of data, the scope of our assessment has been limited. In order to assess the impact of thermal discharges on in stream temperatures, local information on power plant and river discharge rates, mixing regime, and spatially and temporally comprehensive records of temperatures are needed. The EIA collects data on power plant discharges but it has been found that power plant reporting on the volume of water consumed and discharged may be inaccurate (Averyt et al 2013 ). Data reporting to the EIA has improved as of 2010 to include average and peak temperatures on a monthly basis going forward (EIA 2010 ). While the new reporting requirements will provide more comprehensive data in the future, it will take several years before a time series is available to assess temperature trends in cooling source waters or thermal discharges. Although the federal datasets used in this study do not provide enough detail for a full assessment on the impact of once-through cooling systems on aquatic biodiversity, peak temperature data may be comprehensive enough to at least evaluate the risk of current and future regulatory violations. Almost two thirds of US power plants using once-through cooling who are required to report peak intake and discharge temperatures did so continuously from 1996–2005, and less than 1% of these cooling systems reported obviously erroneous data.
6. Conclusion
This study identifies a number of questions for future research. Firstly, the temperature data currently available from the EIA is insufficient to usefully evaluate the ecological impacts of thermal discharges from power plants. More robust analyses may be possible using the physics of heat exchange as a proxy for thermal loading, and some studies are beginning to incorporate this approach (Miara and Vorosmarty 2013 , Stewart et al 2013 ). However, long-term datasets on the ambient intake and discharge water temperatures from power plants may be useful to help calibrate such models.
Climate change appears likely to exacerbate regulatory conflicts in rivers such as the Upper Catawba basin, where thermal discharge temperatures already exceed state limits. River temperatures in the United States are already facing a warming trend due to urbanization and higher air temperatures, the latter of which is expected to increase under climate change (Webb and Nobilis 2007 , Kaushal et al 2010 ). Variations in precipitation from climate scenarios indicate that the impacts of climate change on rivers will not be homogeneous (Milly et al 2008 ). In particular, rivers experiencing decreases in flow will be more strongly affected by warmer air temperatures (Van Vliet et al 2011 ). There is a need for additional risk assessment to identify the specific regions and assess where conflicts between rising river temperatures and regulatory limits are most likely to occur.
In particular, the energy industry needs to prepare for problems related to power generation. Analysis of rising temperatures and the costs of meeting associated regulatory restrictions should be factored in to decisions extending the life of a once-through cooled power plant versus closing or updating its cooling system. For example, the Tennesee Valley Authority has modeled the impact of increased temperatures on power plant cooling and reservoir operations along the Tennessee River (Miller et al 1992 ). There is a need for more local studies of the ecosystem impacts of thermal discharges from power plants, under both current conditions and future warming scenarios. In the United States, it may be useful to focus these studies in the South-Atlantic Gulf, Tennessee, and Ohio River basins, particularly in those watersheds where aquatic biodiversity is high and thermal discharges have already reached regulatory limits.
More than half of power plant cooling systems are already reporting discharging water at maximum temperatures above typical regulatory limits. While the practice of granting thermal variances in NPDES permits offers some flexibility by allowing plants to circumvent existing water quality standards, it is unclear how this can protect aquatic ecosystems that are facing accelerated warming under climate change (Craig 2010 ). Force water quality standards and those who protect endangered species, as well as utilities and other stakeholders. It may also be valuable to consider how watershed management can best adapt to balance human needs for electricity with aquatic ecosystem health.
Acknowledgments
We gratefully acknowledge funding for this research from The Kresge Foundation, Wallace Research Foundation, and Roger and Vicki Sant, and the research oversight provided by the EW3 Scientific Advisory Committee—Peter Frumhoff (Union of Concerned Scientists), George Hornberger (Vanderbilt University), Robert Jackson (Duke University), Robin Newmark (NREL), Jonathan Overpeck (University of Arizona), Brad Udall (University of Colorado Boulder, NOAA Western Water Assessment), and Michael Webber (University of Texas at Austin). In addition, we are thankful for review and guidance from Kristen Averyt (University of Colorado Boulder), James Meldrum (University of Colorado Boulder), and Erika Spanger-Siegfried (Union of Concerned Scientists).
Supplementary data. (267 KB PDF)
Environmental Impacts of Thermal Power Plant: Case Study - Tamil Nadu
- January 2007
- In book: Environmental Impact Assessment: Indo – Australian Perspective (pp.277-293)
- Publisher: New Delhi: Bookwell
- Editors: Behnam Ta’i, Peter Murphy, P.S. Rana

- Center for Advanced Spatial and Environmental Research

- Cranfield University

- Davidson College
Abstract and Figures

Discover the world's research
- 25+ million members
- 160+ million publication pages
- 2.3+ billion citations
- Kathleen Segerson
- David W. Pearce

- William H. Smith
- Brian J. Staskawicz
- David Pearce

- N Jayaraman
- J Govindarajan
- D W Harrington
- A J Buonicore
- Narendran S Sreenath
- David W Martin
- Recruit researchers
- Join for free
- Login Email Tip: Most researchers use their institutional email address as their ResearchGate login Password Forgot password? Keep me logged in Log in or Continue with Google Welcome back! Please log in. Email · Hint Tip: Most researchers use their institutional email address as their ResearchGate login Password Forgot password? Keep me logged in Log in or Continue with Google No account? Sign up
Advertisement
Mitigation strategies for reducing air pollution
- Trend Editorial
- Published: 11 April 2020
- Volume 27 , pages 19226–19235, ( 2020 )
Cite this article
- Daniele Sofia 1 , 2 ,
- Filomena Gioiella 1 ,
- Nicoletta Lotrecchiano 1 , 2 &
- Aristide Giuliano 1 , 3
76k Accesses
120 Citations
51 Altmetric
Explore all metrics
Today, it is increasingly recognized that air pollution hurts human health. Consequently, efficient mitigation strategies need to be implemented for substantial environmental and health co-benefits. A valid approach to reducing the air pollution effects on the environment and human health is proposed. Specific guidelines have been elucidated by differentiating them on the base of the final stakeholders (citizens, enterprises, and public authorities), of the emission sources (transport, household energy, industry, and energy generation sector, agriculture, and shipping area), and of the field of implementation (urban and extra-urban context). This paper can provide useful information for governments for the implementation of a strategic plan focused on emphasizing multi-pollutant emission reductions and overall air pollution-related risk.
Avoid common mistakes on your manuscript.
Introduction
Today, air pollution is the main responsible for environmental quality worsening in many cities all over the world, with adverse outcomes on people’s health (Vlachokostas et al. 2011 ). According to the last World Health Organization (WHO), more than 80% of people living in the urban context are subjected to air quality levels above the emission limits regarding air pollution. The primary atmospheric pollutants are carbon monoxide (CO), particulate matter (PM), nitrogen oxides (NO x ), volatile organic compounds (VOC s ), polycyclic aromatic hydrocarbons (PAH s ), ozone (O 3 ), and sulfur dioxide (SO 2 ). The increase in emission amounts of these pollutants is due to the rapid industrialization and urbanization of developing countries (Fu and Chen 2017 ).
The worsening of air quality in urban environments has considerable interest in the scientific community and public opinion due to the strong relationship between air pollution exposure and increased harmful short- and long-term effects on human health (Masiol et al. 2014 ). Recently, significant epidemiological studies in the literature have found that air pollution contributes to increased morbidity (especially at respiratory and cardiovascular levels), premature mortality, and finally, cancer (Brancato et al. 2018 ). Figure 1 summarizes the different area of diseases: neuronal (Dales et al. 2009 ) (Power et al. 2015 ) (Power et al. 2016 ) (Levy 2015 ) (Block and Calderon-Garciduenas 2009 ), respiratory (Brugha and Grigg 2014 ) (Guan et al. 2016 ) (Kurmi et al. 2010 ) (Goss et al. 2004 ) (Fajersztajn et al. 2013 ), cardiovascular (Zanobetti and Schwartz 2005 ) (Anderson et al. 2012 ) (Maheswaran 2016 ) (Yang et al. 2017 ), and oncology area (Raaschou-Nielsen et al. 2013 ) (Crouse et al. 2010 ). Each disease is affected by own pollutant exposures (PM, NO 2 , SO 2 , CO 2 , O 3 ) with the corresponding level of risk. Human exposure to air pollutions is strongly affected by the lifestyle and by the prevailing life environment (Buonanno et al. 2014 ). In their work, Buonanno et al. ( 2012 ) demonstrated that essential contributions to children exposure are due to the time spent at home for cooking/eating as well as for the time spent in traffic jams moving to or from school (Buonanno et al. 2012 ). In addition to human health risks associated with gases and particles inhalation, urban air pollution also causes damages at an environmental level, for example, by increasing the corrosion and deterioration of materials and damaging historical monuments and buildings (Vlachokostas et al. 2011 ). The effects of air pollution have been studied on many assets of the Italian artistic heritage Depending on where they are located; it was possible to verify the effects of the typical pollutants of the context itself. Sulfur dioxide reflecting the severe air pollution of this very large city can be dangerous for stone monuments as the marble Arch of Titus in Rome (Metallo et al. 1995 ); the particulate matter and the heavy metals as Pb and Zn can damage monuments like the Vittoriano Monument in Rome that is exposed to intense road traffic (Barca et al. 2014 ). The exposure to air pollution can influence also the stability of public utility buildings such as bridges as occurred in Genoa during 2018 when, due to the aggressive environmental condition that corroded the strands, the Polcevera Bridge collapsed causing 43 deaths and more than 5000 evacuated people (Invernizzi et al. 2019 ).
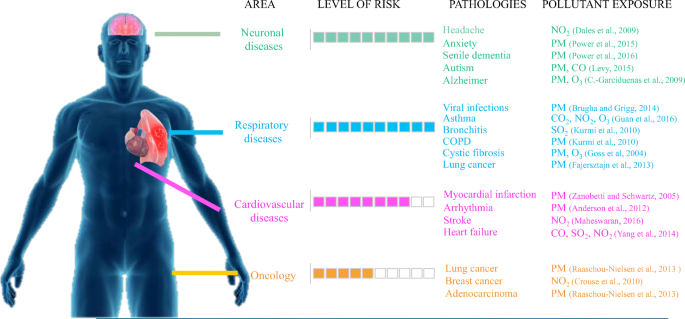
Different areas of diseases in the human body
To tackle these problems, efficient long-term air pollution mitigation strategies need to be identified and implemented (Rodriguez de San Miguel 2019 ). For this reason, the role of the management to improve the current situation becomes critical (Werner et al. 2015 ). It is, therefore, crucial to define a strategic plan with some actions in compliance with the relevant directives in the field of air quality (Vlachokostas et al. 2011 ). These actions strongly depend on local policy and economy, on available technologies, and on public opinion (Harlan and Ruddell 2011 ).
The aim of this work
This paper aims to contribute to the existing knowledge on environmental pollution literature by investigating how people, companies, and committees can contribute to reducing pollution effects by engaging in pro-environmental behaviors. We firmly believe that if these proposed behavioral recommendations are pursued, positive environmental impacts and health co-benefits are very likely to occur. Among the policies that we intend to propose, the promotion of active transport and sharing mobility, the reduction of energy use in the household environment, the urban planning, the provision of benefits in favor of bio-fueled (Sofia et al. 2013 ), and the use of electric vehicles are included.
Recommendations for citizens
To mitigate the air pollution problem, many efforts have to be taken with the aim to decrease the pollutants emissions coming from people. Each citizen may contribute to the mitigation of air pollution through behavioral changes in their lifestyle as the reduction of energy consumption in transportation, households, and supply.
Public and active transport
Transportation is the central investigated sector for public health benefits obtained after air pollution reduction (Sarigiannis et al. 2017 ) (Lindsay et al. 2011 ). It is well known that vehicular transport produces about 70% of environmental pollution since exhaust fumes from motors are a source of several pollutants (CO, NO 2 , VOC, and PM) (Xia et al. 2015 ). Consequently, programs aiming at changing travel behaviors are essential (Guersola et al. 2017 ). Each citizen should use public transports (bus, tram, subway, train) as much as possible and possibly travel actively (walking and cycling). The shift to active transport by reducing the use of owned cars entails significant benefits for human health and environment (Rabl and de Nazelle 2012 ) (Maizlish et al. 2013 ) (Xia et al. 2015 ). Recently, several studies have shown that the increase in physical activity reduces the incidence of several diseases, especially at cardiovascular level (coronary heart disease, stroke), hypertension, and diabetes (Mueller et al. 2015 ) (Scheepers et al. 2014 ). Furthermore, in this way, significant reductions of colon and breast cancer and the improvement of mental health can be achieved (Rabl and de Nazelle 2012 ). Of course, benefits from physical activity are obtained by minimizing exposure to atmospheric pollution; therefore, the outdoor activity has to be carried out in the environment with healthy air (Rabl and de Nazelle 2012 ). Furthermore, commuters should be encouraged to use low-cost public bicycle sharing systems to combine benefits concerning health and air pollution reduction (Rojas-Rueda et al. 2011 ).
Household sector
Nowadays, household air pollution is attributed to the residential use of the solid fuels from cooking activities (Stabile et al. 2014 ) and space heating systems (Stabile et al. 2018 ), leading a significant hazard for the health of exposed populations (Gao et al. 2018 ). Accordingly, actions to reduce energy use by households and buildings are essential because of their great contribution to gas emissions (Datta et al. 2017 ). One of the appropriate strategies is the improvement of combustion efficiency of solid household fuels (Venkataraman et al. 2010 ). Generally, traditional fuels have low combustion efficiency producing accordingly large amounts of products due to incomplete combustion, with consequences for both environment and human health. In their work, Marchetti et al. ( 2019 ) demonstrated how the particles deriving from the combustion different fuels (pellet, wood, charcoal) could activate toxicological pathways, finally producing cytotoxic effects on human health (Marchetti et al. 2019 ). The fuel toxicity is dependent on the chemical composition of the particulate matter characterizing the quality of the combustion and fuel. The energy generated from renewable sources (biomass) should lead health benefits for citizen because of a cleaner environment with low emission production (Harlan and Ruddell 2011 ) with respect to the traditional fossil fuel used.
Nevertheless, more stringent regulations are required to guarantee high-quality biomass fuels and safer combustion technologies (Marchetti et al. 2019 ). On their side, each citizen has to adopt some behavior actions to reduce energy consumption and emissions deriving from home heating. Another essential structural adaptation is the introduction of new technologies to reduce energy use in new buildings (Ruparathna et al. 2017 ). Porritt et al. ( 2012 ) showed how limited changes in building are able to eliminate overheating during heat wave periods and reduce space energy use for internal climatization, such as external wall insulation, solar reflective coatings (external shutters), and painting of the outer walls in lighter colors (Porritt et al. 2011 ) (Porritt et al. 2012 ). Furthermore, green roof technologies can help to reduce local outdoor temperatures and improve the appropriate cooling inside buildings (Harlan and Ruddell 2011 ).
Despite efforts to reduce particle emissions deriving from outdoor activities, most of air pollution is related to indoor microenvironment (Buonanno et al. 2017 ). The air quality inside buildings is affected by the air circulation, the construction materials, the use of cleaning products, and the habits of occupants (smoking). A vast range of pollutants can concentrate in indoor environments produced by individual activities in addition to outdoor concentrations (Settimo 2015 ). As a consequence, air exchange with particle filters, ventilating (Debnath et al. 2017 ), and air-conditioning systems are a distinct way of reducing air pollution in indoor spaces, like homes or shared communities (offices, schools, hospitals, sport facilities, restaurants, cinemas, and public transport) (Kwong et al. 2019 ). Among public buildings, school is one of the worrisome indoor environments since children represent a susceptible population to air pollution due to their age (Mainka et al. 2015 ).
Healthy diet
Beyond mitigation strategies to reduce air pollution, each citizen can adopt some eating habits that can influence own health status (Biesbroek et al. 2014 ). It was well demonstrated that the increased intake of antioxidants in foods could hinder and reduce the adverse effects of atmospheric pollution (Kelly et al. 2003 ). Precisely, the antioxidants are substances able to neutralize free radicals generated by some air pollutants (ozone and nitrogen dioxide). In this way, injury to respiratory tract like asthma can be avoided after their oxidant exposure (Romieu et al. 2002 ). Therefore, it is necessary to reduce the consumption of food deriving from animal source by promoting a healthy diet with higher consumption of fruit and vegetables.
Recommendations to small, medium, and large enterprises
Globally, one of the main contributors to emissions of atmospheric pollutants and a significant user of energy is the industrial sector (Conti et al. 2015 ). The pollutants deriving from industrial activities are transported into the urbanized areas. Consequently, the development of strategies to reduce air pollution is crucial. In this section, possible measures relating to industrial, agriculture, and shipping sector are introduced such as energy reduction (Pask et al. 2017 ), advanced technologies and process performance promotion (Contreras-Zarazúa et al. 2018 ), improvement of the efficiency of livestock farming and manure management, and electrification of the port docks.
Industrial sector
Even today, the primary source of energy are fossil fuels, responsible for the production of some pollutants notably particulate matter (PM) (Salehi et al. 2015 ), nitrogen oxides (NO x ), and sulfur oxides (SO x ) (Chao 2008 ). The reduction of power generation from fossil fuel sources (coal, oil, gas) imply health benefits by reducing local air pollutants, especially micronic and submicronic particles (Karka et al. 2017 ). Recently, several initiatives to replace fossil fuels with alternative renewable fuels have been taken into consideration (Ribeiro et al. 2015 ). Among the various technologies for energy production from renewable sources, the biomass combustion can represent a valid alternative technology of fossil fuels (Sripada et al. 2017 ) (Giuliano et al. 2018a ). Shrestha and Shakya ( 2012 ) showed that the implementation of the cost minimization energy system MARKAL, based on the market allocation framework, reduces the local pollutant emissions, improving the efficiency of the national overall energy consumption. This strategy includes energy supply, conversion and process technology, end-use service demand, and environmental emissions promoting the use of renewable energy resources (Shrestha and Shakya 2012 ). In this way, cities will have benefits if they will move toward low carbon technologies (Ren et al. 2012 ).
Among the various industrial sectors, one of the primary sources of the main pollutants (VOCs, toxins, PAH) is the chemical industry (Lee and Cho 2003 ). As a consequence, proper air pollution control techniques have to be applied to reduce the negative environmental impact (Contreras-Zarazúa et al. 2018 ). Another mitigation strategy to reduce air pollution from the industrial sector is the implementation of advanced technologies in the industrial process (Babar and Shareefdeen 2014 ). For example, clean coal technologies (CCT) can treat and use coal in an efficient way without a substantial environmental impact (Giuliano et al. 2018b ). Besides, it was demonstrated that retrofitted technologies such as catalytic converters and desulfurization reduce only local air pollution.
On the other hand, the benefits of integrated environmental strategies are higher than the ones given by air quality management plans and measures for GHG reduction. This result is highlighted in the study of Chae and Park, who demonstrated that using compressed natural gas and an efficient heating and cooling systems, both local and global air pollution reduction can be achieved (Chae and Park 2011 ). Furthermore, the best available techniques (BAT) are promoted to reduce the environmental impacts deriving from industrial activities since they operate minimizing costs (Ibáñez-Forés et al. 2013 ). This technology has to be “available” that means usable to the operator and economically and technically feasible. Additionally, it has to be “best” that means provide a high level of environmental protection as a whole (Liu and Wen 2012 ). As a consequence, plant owners of different industrial sectors have to select the BAT that is appropriate for their conditions.
Finally, the change in average working hours in a very efficient way that could have a good impact on consumption and related environmental pressure (Bergh et al. 2011 ).
Even if the literature available is still low, some studies demonstrated that changing the times of going to work, shifts, brackets, rationalizing home-office travel times, and the rigidity of schedules can reduce traffic congestion and CO 2 and fine dust emissions, PM10, and PM2.5 as well as employee stress with a positive return on the quality of work and the competitiveness of businesses (Ge et al. 2018 ). Furthermore, it is possible to improve air quality by promoting online work, avoiding not strictly necessary car moving. Another example is to support the vertical part-time (fewer days a week but more hours a day), halving the mowing toward the workplace.
Agriculture and food sector
The majority of fine particulate originates not only from combustion processes in traffic, power plants, industry, and household energy use but also from sources related to agriculture (Martins et al. 2015 ). One of the particulate precursors is ammonia (NH 3 ) after the reaction with the sulfuric and nitric acid (Erisman and Schaap 2004 ). It has been estimated that about 80% of NH 3 entering in the atmosphere is produced by agricultural activities in Europe (Velthof et al. 2012 ). In agriculture, the main sources responsible for NH 3 production are the excretion of urine by livestock and the manure storage (Velthof et al. 2012 ). There are many specific changes to mitigate NH 3 emissions in agriculture (Giannadaki et al. 2018 ). These imply the improvement of the technology and the management of agricultural productions, but also include the reduction of food wastes combined with human diet optimization (Zhao et al. 2017 ). In fact, the NH 3 emission levels depend on the animal typology, with higher amounts for beef and sheep, and a lower amount for pigs and poultry. Four strategies are identified in this study to reduce NH 3 by focusing mainly on livestock:
Improvement in livestock farming efficiency: The livestock farming efficiency can be improved by supporting local farmers’ markets and community gardens, in order to reduce the traveled distances of transported goods. Agriculture and land use increases the demand for deforestation, increasing the levels of atmospheric CO 2 produced promoting climate change (Younger et al. 2008 ).
Manure management optimization: Besides NH 3 emission, livestock manure contributes to other substances, mainly methane (CH 4 ) and nitrous oxide (N 2 O). These emissions derive from various phases in the use of manure ranging from the handling and storage to the application as a fertilizer to soils (Mohankumar et al. 2017 ). Some abatement options need to be developed like lowering the dietary crude protein content, external slurry storage via acidification, frequent removal of manure, and covers of straw or artificial films (Mohankumar et al. 2017 ) (Hou et al. 2015 ).
Reduction in the use of fossil fuels: Another revolution in agricultural sector concerns the reduction of dependence on non-renewable energy. Oil is also used to produce nitrogenous fertilizers (McMichael et al. 2007 ).
Reduction in the production and consumption of foods from animal sources: It is necessary to promote more healthy diets with low consumption of foods from animal sources (Friel et al. 2009 ).
Shipping sector
Nowadays, the shipping sector provides low-cost and reliable delivery services in the economic field (Arunachalam et al. 2015 ). Nevertheless, shipping-related activities have a considerable impact on air pollution, especially in coastal areas but also globally (Buccolieri et al. 2016 ). The primary air pollutants are PM, VOCs, NO x , O 3 , SO 2 , and CO (Bailey and Solomon 2004 ). As a consequence, a wide range of options toward “greener” seaports is needed (Bailey and Solomon 2004 ). Some of these measures are easy to adopt such as the regulation of fuel quality (by using low-sulfur alternative fuels), the speed reduction (Lack et al. 2011 ), and the use of alternative transportation equipment (Lai et al. 2011 ).
Furthermore, a variety of technical strategies for reducing ship emissions have to be adopted. NO x and SO x emission reduction strategies consist in lowering combustion temperature, switching to lower sulfur marine fuels and using seawater scrubbing (Han 2010 ). In addition, it is essential to operate in modifying the entry and the docking of ships in the harbor to reduce local emissions. A precautionary approach includes the dock electrification that means the shore-side power for docked vessels to avoid the motor power on during the stop (Dhupia et al. 2011 ).
Recommendations to local/provincial/regional/national authorities
Rapid industrialization, as well as urbanization in developing countries, has led to an increase in air pollution with adverse effects on human health. As a consequence, the development of city action plans that includes mitigation and adaptions strategies to emphasize pollutant emission reduction is an important step toward the better well-being of life. Therefore, the responsibility for urban areas such as governance bodies (local/provincial/regional/national authorities) is involved in planning the correct strategies aimed at improving air quality. In this section, more comprehensive management measures focused on emphasizing pollutant and emission sources reductions at both local and regional levels are proposed to mitigate the air pollution issue. The options include the implementation of new regulations, urban planning reorganization, and promotion of hybrid vehicles with low emissions.
New regulations
Air quality management policies have to fix new air quality standards that maximize overall population benefits, reduce illness related to air pollution and gas emissions from industrial, urban, or domestic activities (Fann et al. 2011 ). It is essential to identify effective structural and exceptional measures throughout the national territory.
Advisory and prevention
Frequently, acting with mitigation strategies after critical levels of pollution does not solve the pollution emergency. For this reason, it is necessary to move toward a “preventive approach to the emergency” by promoting effective measures before reaching critical levels of pollution (Bandyopadhyay et al. 2014 ). In this context, the authorities should support new technologies for air pollution monitoring (Mishra et al. 2015 ). Air pollution monitoring networks offer the possibility to measure the spatiotemporal distribution of air pollution in the urban environment for the health and safety of citizens (Singla et al. 2018 ) (Sofia et al. 2018a ). For example, sensor networks offer the potential to focus on air pollution monitoring reflecting high spatial and temporal variability in pollutant levels (Knox et al. 2013 ) (Sofia et al. 2018b ). In this way, if a particular pollutant exceeds the target limit, efficient strategies should be adopted to mitigate the air pollution issue and find the pollution sources. Furthermore, air quality prediction models are another way to make a rational decision by political leaders (Vicente et al. 2018 ). The combination of air quality monitoring and modeling is a valid approach for regulatory purposes (Vlachokostas et al. 2011 ).
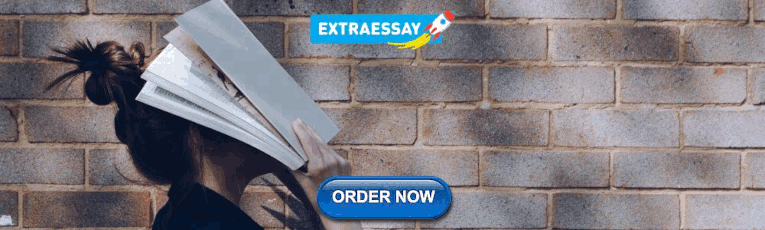
Urban planning
Rapid urbanization has involved significant challenges in urban areas with dramatic consequences in air quality. The primary source of atmospheric pollution is vehicular traffic (Pospisil and Jicha 2017 ). The emissions from vehicles are different throughout the day with a maximum concentration during the more congested hours (Kumar et al. 2016 ). Therefore, policymakers have to support the implementation of strategies and actions aimed at reducing air pollution in urban areas while promoting economic growth and higher quality of life (Vranckx et al. 2015 ). In this context, the concept of “smart city” has emerged as a way to respond to the inhabitants’ needs more efficiently and sustainably. In urban planning, smart mobility represents a crucial factor. Since a major part of pollutant emissions in cities are due to traffic, an appropriate transport design in the urban area is needed (Cariolet et al. 2018 ). Political leaders have to promote changes in travel behaviors by supporting public transport (Sellitto et al. 2015 ) and the sharing of mobility. The strong inclination toward traffic congestion reduction promoted by policymakers is not always conformed to health promotion. Appropriate safety interventions must be proposed to have health benefits, especially for cyclists and pedestrians (Rojas-Rueda et al. 2016 ).
Regarding public bicycle sharing, it is now spreading in different countries in Europe, Asia, and America as a healthier transport system in the urban context (Rojas-Rueda et al. 2011 ). In addition, the reduction in private car use can be reached only ensuring public transport availability, cycling infrastructure, and green spaces (Panter et al. 2016 ). Currently, many cities in the world are moving toward mobility solutions implementing car-free days, strengthening the infrastructures and public transport (Nieuwenhuijsen and Khreis 2016 ). The objective is to reduce the traffic-related air pollution and provide strong opportunities to increase free spaces that can be used to improve the urban green with parks and open areas or attractive places (public squares, shops) for citizens and tourists (Nieuwenhuijsen and Khreis 2016 ). Furthermore, the reduction in vehicular traffic will certainly lead other human health benefits like the reduction of road accidents (Nieuwenhuijsen and Khreis 2016 ). All these mitigation measures have to be promoted in the long-term to obtain significant changes in emission reductions and human health benefits. In fact, in evaluating the effects of commonly adopted mitigation strategies such as car free-days in a large city of Po Valley (Northern Italy), Masiol and their colleagues (Masiol et al. 2014 ) did not find significant changes probably due to the very short time of mitigation procedure (Masiol et al. 2014 ).
In addition, the reduction in private cars use promotes the increase in public space for vegetation and retail goals. Roadside vegetation barriers can be a potential mitigation strategy for near-road air pollution (Isakov et al. 2017 ). In their work, Tong et al. ( 2016 ) demonstrated that a wide vegetation barrier combined with a solid barrier reduces pollutant concentrations significantly (Tong et al. 2016 ). Urban vegetation impacts our ecosystem positively by filtrating airborne particulate matter, providing a scenic public landscape and reducing flooding consequences (Al-thani et al. 2018 ).
Promotion of hybrid vehicles
It is well known that vehicle emissions (NOx, HC, O 3 , VOC, CO, and PM) contribute to air pollution (Wu et al. 2017 ). In this scenario, besides the implementation of increasingly stringent standards for vehicle emissions, the most effective policy is the promotion of the zero-emission vehicle (Perez et al. 2015 ). In particular, by using alternative fuels, respect to the traditional fossil ones, like electricity, bio-fuels, liquefied petroleum gas (LPG), natural gas (CHG, LNG), and, methane, this kind of cars can produce lower concentrations of pollutants (Qiu et al. 2016 ).
With the rapid industrialization, the hybrid electric vehicle (HEV) technology is a valid alternative to the fuel prices rising and to satisfy the more effective environmental policies (Xia et al. 2015 ) (Sabri et al. 2016 ). The combination to active travel with zero-emission vehicles can reduce the cases of ischemic heart disease (Woodcock et al. 2009 ). The government has to make a series of laws aimed at encouraging electric mobility such as tax incentives and lower prices for usage or parking (Leurent and Windisch 2011 ).
Conclusions
This study summarizes the mitigation strategies that can be adopted by different stakeholders (citizens, companies, and committees) to obtain public health co-benefits with air pollution reduction. In particular, specific guidelines were provided in various sectors: transportation, industry, household, energy generation, agriculture, and shipping sector. These guidelines can be considered a basis for governments for the implementation of a strategic plan focused on the reduction of multi-pollutant emission, as well as of the overall air pollution-related risk. Individuals can also adopt environmental friendlier behaviors that together with mitigation policies, can obtain health and environment co-benefits. The strategic measures proposed, differing for the stakeholder (citizens, enterprises, and public authorities) on the application kind (direct/indirect measure), for the emission sources (transport, household energy, industry and energy generation sector, food, and agriculture) and for the area of implementation (urban and extra-urban context) that can be reassumed by Fig. 2 .
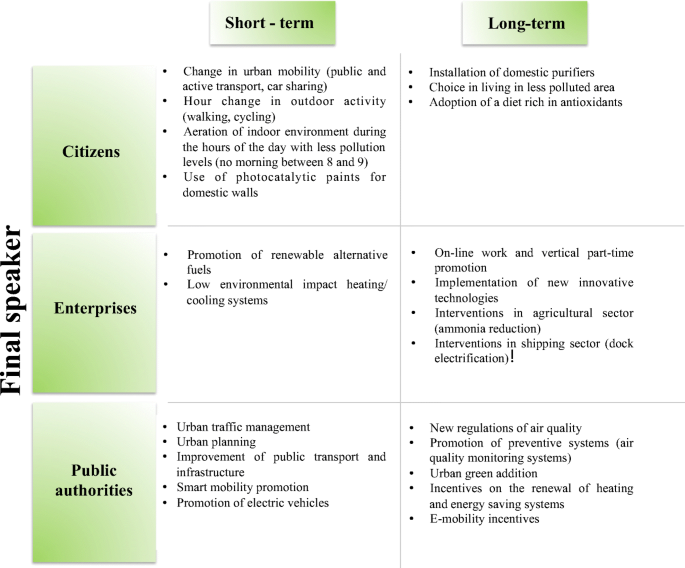
The mitigation strategies or strategic measures proposed that can be adopted by different stakeholders to obtain public health co-benefits with air pollution reduction
Al-thani H, Koç M, Isaifan RJ (2018) A review on the direct effect of particulate atmospheric pollution on materials and its mitigation for sustainable cities and societies. Environ Sci Pollut Res 25(28):27839–27857
CAS Google Scholar
Anderson JO, Thundiyil JG, Stolbach A (2012) Clearing the air: a review of the effects of particulate matter air pollution on human health. J Med Toxicol 8(2):166–175
Arunachalam S, Brantley H, Barzyk TM, Hagler G, Isakov V, Kimbrough E, Naess B, Rice N, Snyder MG, Talgo K, Venkatram A (2015) Assessment of port-related air quality impacts: geographic analysis of population. Int J Environ Pollut 58(4):231–250
Babar ZB, Shareefdeen Z (2014) Management and control of air emissions from electronic industries. Clean Techn Environ Policy 16(1):69–77
Google Scholar
Bailey D, Solomon G (2004) Pollution prevention at ports: clearing the air. Environ Impact Assess Rev 24(7):749–774
Bandyopadhyay A, Bandyopadhyay S, Biswas MN (2014) Fabrication and operation of an online photoelectric particulate matter monitoring device. Clean Techn Environ Policy 17(1):265–271
Barca D, Comite V, Belfiore CM, Bonazza A, La Russa MF, Ruffolo SA, Crisci GM, Pezzino A, Sabbioni C (2014) Impact of air pollution in deterioration of carbonate building materials in Italian urban environments. Appl Geochem 48:122–131
Bergh J, Van Den CJM, Allen W (2011) Environment versus growth — a criticism of ‘degrowth’ and a Plea for ‘a-growth’. Ecol Econ 70(5):881–890
Biesbroek S, Bueno-De-Mesquita HB, Peeters PHM, Verschuren WM, Van Der Schouw YT, Kramer GFH, Tyszler M, Temme EHM (2014) Reducing our environmental footprint and improving our health: greenhouse gas emission and land use of usual diet and mortality in EPIC-NL: a prospective cohort study. Environ Health 13(1):1–9
Block ML, Calderon-Garciduenas L (2009) Air pollution: mechanism of neuroinflammation and CNS disease. Trends Neurosci 32(9):506–516
Brancato V, Gioiella F, Imparato G, Guarnieri D, Urciuolo F, Netti PA (2018) 3D breast Cancer microtissue reveals the role of tumor microenvironment on the transport and efficacy of free-doxorubicin in vitro. Acta Biomater 75:200–212
Brugha R, Grigg J (2014) Urban air pollution and respiratory infections. Paediatr Respir Rev 15(2):194–199
Buccolieri R, Cesari R, Dinoi A, Maurizi A, Tampieri F, Di Sabatino S (2016) Impact of ship emissions on local air quality in a Mediterranean City’s harbour after the European sulphur directive. Int J Environ Pollut 59(1):30–42
Buonanno G, Marini S, Morawska L, Fuoco FC (2012) Individual dose and exposure of Italian children to ultrafine particles. Sci Total Environ 438:271–277
Buonanno G, Stabile L, Morawska L (2014) Personal exposure to ultrafine particles: the influence of time-activity patterns. Sci Total Environ 468-469:903–907
Buonanno G, Stabile L, Morawska L, Giovinco G, Querol X (2017) Do air quality targets really represent safe limits for lung cancer risk? Sci Total Environ 580:74–82
Cariolet JM, Colombert M, Vuillet M, Diab Y (2018) Assessing the resilience of urban areas to traffic-related air pollution: application in greater Paris. Sci Total Environ 615(615):588–596
Chae Y, Park J (2011) Quantifying costs and benefits of integrated environmental strategies of air quality management and greenhouse gas reduction in the Seoul metropolitan area. Energy Policy 39(9):5296–5308
Chao K (2008) A new look at the cross-impact matrix and its application in futures studies. J Futures Stud 12(4):45–52
Conti ME, Ciasullo R, Tudino MB, Matta EJ (2015) “The industrial emissions trend and the problem of the implementation of the industrial emissions directive (IED)” Air Quality . Atmosph Health 8(2):151–161
Contreras-Zarazúa G, Villicaña-García E, Cansino-Loeza B, Vázquez-Castillo JA, Ponce-Ortega JM, Segovia-Hernández JG (2018) Environmental impact and occupational Hazard evaluation on intensified processes to produce diphenyl carbonate. Comput Chem Eng:1–12
Crouse DL, Goldberg MS, Ross NA, Chen H, Labreche F (2010) Postmenopausal breast Cancer is associated with exposure to traffic-related air pollution in Montreal, Canada: a case-control study. Environ Health Perspect 118(11):1578–1583
Dales RE, Cakmak S, Vidal CB (2009) Air pollution and hospitalization for headache in Chile. Am J Epidemiol 170(8):1057–1066
Datta A, Sharma VP, Gaine T, Sehgal M (2017) Mitigation of domestic indoor air pollution in a pristine rural area of India. Manag Environ Qual 28:231–247
Debnath R, Bardhan R, Banerjee R (2017) Taming the killer in the kitchen: mitigating household air pollution from solid-fuel cookstoves through building design. Clean Techn Environ Policy 19(3):705–719
Dhupia J, Adnanes AK, Lee KM Kennedy L (2011) Electrification of port and port operations. Proceedings of MTEC2011, pp. 1–6
Erisman JW, Schaap M (2004) The need for ammonia abatement with respect to secondary PM reductions in Europe. Environ Pollut 129(1):159–163
Fajersztajn L, Veras M, Barrozo LV, Saldiva P (2013) Air pollution: a potentially modifiable risk factor for lung cancer. Nat Rev Cancer 13(9):674–678
Fann N, Roman HA, Fulcher CM, Gentile MA, Hubbell BJ, Wesson K, Levy JI (2011) Maximizing health benefits and minimizing inequality: incorporating local-scale data in the design and evaluation of air quality policies. Risk Anal 31(6):908–922
Friel S, Dangour AD, Garnett T, Lock K, Chalabi Z, Roberts I, Butler A, Butler CD, Waage J, McMichael AJ, Haines A (2009) Public health benefits of strategies to reduce greenhouse-gas emissions: food and agriculture. Lancet 374(9706):2016–2025
Fu H, Chen J (2017) Formation, Features and controlling strategies of severe haze-fog pollutions in China. Sci Total Environ 578:121–138
Gao J, Hou H, Zhai Y, Woodward A, Vardoulakis S, Kovats S, Wilkinson P, Li L, Song X, Xu L, Meng B, Liu X, Wang J, Zhao J, Liu Q (2018) Greenhouse gas emissions reduction in different economic sectors: mitigation measures, health co-benefits, knowledge gaps, and policy implications. Environ Pollut 240(1):683–698
Ge J, Polhill JG, Craig TP (2018) Too much of a good thing? Using a spatial agent-based model to evaluate “unconventional” workplace sharing programmes. J Transp Geogr 69:83–97
Giannadaki D, Giannakis E, Pozzer A, Lelieveld J (2018) Estimating health and economic benefits of reductions in air pollution from agriculture. Sci Total Environ 622-623:1304–1316
Giuliano A, Gioiella F, Sofia D, Lotrecchiano N (2018a) A novel methodology and technology to promote the social acceptance of biomass Power plants avoiding Nimby syndrome. Chem Eng Trans 67:307–312
Giuliano A, Poletto M, Barletta D (2018b) Pure hydrogen co-production by membrane technology in an IGCC power plant with carbon capture. Int J Hydrog Energy 43(41):19279–19292
Goss CH, Newsom SA, Schildcrout JS, Sheppard L, Kaufman JD (2004) Effect of ambient air pollution on pulmonary exacerbations and lung function in cystic fibrosis. Am J Respir Crit Care Med 169(7):816–821
Guan WJ, Zheng XY, Chung KF, Zhong NS (2016) Impact of air pollution on the burden of chronic respiratory diseases in China: time for urgent action. Lancet 388(10054):1939–1951
Guersola M, Steiner MTA, Scarpin CT (2017) A methodology for minimizing LPG transportation impact. Manag Environ Qual 28:807–820
Han CH (2010) Strategies to reduce air pollution in shipping industry. Asian J Ship Logistic 26(1):7–30
Harlan SL, Ruddell DM (2011) Climate change and health in cities: impacts of heat and air pollution and potential co-benefits from mitigation and adaptation. Current Option in Environmental Sustainability 3(3):126–134
Hou Y, Velthof GL, Oenema O (2015) Mitigation of Ammonia, nitrous oxide and methane emissions from manure management chains: a meta-analysis and integrated assessment. Glob Chang Biol 21(3):1293–1312
Ibáñez-Forés V, Bovea MD, Azapagic A (2013) Assessing the sustainability of best available techniques (BAT): methodology and application in the ceramic tiles industry. J Clean Prod 51:162–176
Invernizzi S, Montagnoli F, Carpinteri A (2019) Fatigue assessment of the collapsed XX th century cable-stayed Polcevera bridge in Genoa. Proced Struct Integ 18:237–244
Isakov V, Venkatram A, Baldauf R, Deshmukh P, Zhang M (2017) Evaluation and development of tools to quantify the impacts of roadside vegetation barriers on near-road air quality. Int J Environ Pollut 62(2):127–135
Karka P, Papadokonstantakis S, Kokossis A (2017) Cradle-to-gate assessment of environmental impacts for a broad set of biomass-to-product process chains. Int J Life Cycle Assess 22(9):1418–1440
Kelly FJ, Dunster C, Mudway I (2003) Air pollution and the elderly: oxidant/antioxidant issues worth consideration. Eur Respir J 40:70s–75s
Knox A, Mykhaylova N, Evans GJ, Lee CJ, Karney B, Brook JR (2013) The expanding scope of air pollution monitoring can facilitate sustainable development. Sci Total Environ 448:189–196
Kumar A, Patil RS, Dikshit AK, Kumar R (2016) Comparison of predicted vehicular pollution concentration with air quality standards for different time periods. Clean Techn Environ Policy 18(7):2293–2303
Kurmi OP, Semple S, Simkhada P, Smith WCS, Ayres JG (2010) COPD and chronic bronchitis risk of indoor air pollution from solid fuel: a systematic review and meta-analysis. Thorax 65(3):221–228
Kwong QJ, Abdullah J, Chuan Tan S, Thio THG, Yeaw WS (2019) A field study of indoor air quality and occupant perception in experimental laboratories and workshops. Manag Environ Qual 30:467–482
Lack DA, Cappa CD, Langridge J, Bahreini R, Buffaloe G, Brock C, Cerully K, Coffman D, Hayden K, Holloway J, Lerner B, Massoli P, Li SM, McLaren R, Middlebrook AM, Moore R, Nenes A, Nuaaman I, Onasch TB, Peischl J, Perring A, Quinn PK, Ryerson T, Schwartz JP, Spackman R, Wofsy SC, Worsnop D, Xiang B, Williams E (2011) Impact of fuel quality regulation and speed reductions on shipping emissions: implications for climate and air quality. Environ Sci Technol 45(20):9052–9060
Lai KH, Lun VYH, Wong CWY, Cheng TCE (2011) Green shipping practices in the shipping industry: conceptualization, adoption, and implications. Resour Conserv Recycl 55(6):631–638
Lee BK, Cho SW (2003) Strategies for emission reduction of air pollutants produced from a chemical plant. Environ Manag 31(1):42–49
Leurent F, Windisch E (2011) Triggering the development of electric mobility: a review of public policies. Eur Transp Res Rev 3(4):221–235
Levy RJ (2015) Carbon monoxide pollution and neurodevelopment: A public health concern. Neurotoxicol Teratol 49:31–40
Lindsay G, Macmillan A, Woodward A (2011) Moving urban trips from cars to bicycles: impact on health and emissions. Aust N Z J Public Health 35(1):54–60
Liu X, Wen Z (2012) Best available techniques and pollution control: a case study on China’s thermal power industry. J Clean Prod 23:113–121
Maheswaran R (2016) Air pollution and stroke – an overview of the evidence base. Spatial Spatio-temp Epidemiol 18:74–81
Mainka A, Zajusz-Zubek E, Kaczmarek K (2015) PM10 composition in urban and rural nursery schools in upper Silesia, Poland: trace elements analysis. Int J Environ Res Public Health 12(7):7990–8008
Maizlish N, Woodcock J, Co S, Ostro B, Fanai A, Fairley D (2013) Health cobenefits and transportation-related reductions in greenhouse gas emissions in the San Francisco Bay Area. Am J Public Health 103(4):703–709
Marchetti LE, Bengalli R, Avino P, Stabile L, Buonanno G, Colombo A, Camatini M, Mantecca P (2019) In vitro lung toxicity of indoor PM10 from a stove fueled with different biomasses. Sci Total Environ 649:1422–1433
Martins H, Monteiro A, Ferreira J, Gama C, Ribeiro I, Borrego C, Miranda AI (2015) The role of ammonia on particulate matter pollution over Portugal. Int J Environ Pollut 57(3/4):215–226
Masiol M, Agostinelli C, Formenton G, Tarabotti E, Pavoni B (2014) Thirteen years of air pollution hourly monitoring in a large city: potential sources, trends, cycles and effects of car-free days. Sci Total Environ 494(495):84–96
McMichael AJ, Powles JW, Butler CD, Uauy R (2007) Food, livestock production, energy, climate change, and health. Lancet 370(9594):1253–1263
Metallo MC, Poli AA, Diana M, Persia F, Cirillo MC (1995) Air pollution loads on historical monuments: an air quality model application to the marble arch of Titus in Rome. Sci Total Environ 171:63–172
Mishra RK, Joshi T, Goel NI, Gupta H, Kumar A (2015) Monitoring and analysis of PM 10 concentration at Delhi Metro construction sites. Int J Environ Pollut 57(1/2):27–37
Mohankumar S, Purath E, Winiwarter W, Amon B (2017) Greenhouse gas and ammonia emissions from different stages of liquid manure management chains: abatement options and emission interactions. J Environ Qual 47(1):30–41
Mueller N, Rojas-Rueda D, Cole-Hunter T, de Nazelle A, Dons E, Gerike R, Götschi T, Int Panis L, Kahlmeier S, Nieuwenhuijsen M (2015) Health impact assessment of active transportation: a systematic review. Prev Med 76:103–114
Nieuwenhuijsen MJ, Khreis H (2016) Car free cities: pathway to healthy urban living. Environ Int 94:251–262
Panter J, Heinen E, Mackett R, Ogilvie D (2016) Impact of new transport infrastructure on walking, cycling, and physical activity. Am J Prev Med 50(2):45–53
Pask F, Lake P, Yang A, Tokos H, Sadhunkhan J (2017) Industrial oven improvement for energy reduction and enhanced process performance. Clean Techn Environ Policy 19(1):215–224
Perez L, Trüeb S, Cowie H, Keuken MP, Mudu P, Ragettli MS, Sarigiannis DA, Tobollik M, Tuomisto J, Vienneau D, Sabel C, Künzli N (2015) Transport-related measures to mitigate climate change in Basel, Switzerland: a health-effectiveness comparison study. Environ Int 85:111–119
Porritt S, Shao L, Cropper P, Goodier C (2011) Adapting dwellings for heat waves. Sustain Cities Soc 1(2):81–90
Porritt SM, Cropper PC, Shao L, Goodier CI (2012) Ranking of interventions to reduce dwelling overheating during heat waves. Energy Build 55:16–27
Pospisil J, Jicha M (2017) Influence of vehicle-induced turbulence on pollutant dispersion in street canyon and adjacent urban area. Int J Environ Pollut 62:2–4
Power MC, Kioumourtzoglou M-A, Hart JE, Okereke OI, Laden F, Weisskopf MG (2015) The relation between past exposure to fine particulate air pollution and prevalent anxiety: observational cohort study. BMJ 350:1–9
Power MC, Adar SD, Yanosky JD, Weuve J (2016) Exposure to air pollution as a potential contributor to cognitive function, cognitive decline, brain imaging, and dementia: A systematic review of epidemiologic research. NeuroToxicology 56:235–253
Qiu Z, Li X, Hao Y, Deng S (2016) Potential of diesel emissions reduction strategies in Xi’an, China. Clean Techn Environ Policy 18(8):2717–2724
Raaschou-Nielsen O, Zorana AJ, Beelen R, Samoli E, Stafoggia M, Weinmayr G, Hoffmann B, Fischer P, Nieuwenhuijsen MJ, Brunekreef B, Xun WW, Katsouyanni K, Dimakopoulou K, Sommar J, Forsberg B, Modig L, Oudin A, Oftedal B, Schwarze PE, Nafstad P, De Faire U, Pedersen NL, Östenson CG, Fratiglioni L, Penell J, Korek M et al (2013) Air pollution and lung cancer incidence in 17 European cohorts: prospective analyses from the European study of cohorts for air pollution effects (ESCAPE). Lancet Oncol 14(9):813–822
Rabl A, de Nazelle A (2012) Benefits of shift from car to active transport. Transp Policy 19(1):121–131
Ren WX, Geng Y, Xue B, Fujita T, Ma Z, Jiang P (2012) Pursuing co-benefits in China’s old industrial base: a case of Shenyang. Urban Clim 1(72):55–64
Ribeiro I, Monteiro A, Martins H, Freitas S, Borrego C, Lopes M (2015) How does the use of biodiesel affect urban air quality? Int J Environ Pollut 58:79–88
Rodriguez de San Miguel JA (2019) Management of air pollution in Mexico. Manag Environ Qual 30:578–592
Rojas-Rueda D, de Nazelle A, Tainio M, Nieuwenhuijsen MJ (2011) The health risks and benefits of cycling in urban environments compared with car use: health impact assessment study. Bmj 343:1–8
Rojas-Rueda D, De Nazelle A, Andersen ZJ, Braun-Fahrländer C, Bruha J, Bruhova-Foltynova H, Desqueyroux H, Praznoczy C, Ragettli MS, Tainio M, Nieuwenhuijsen MJ (2016) Health impacts of active transportation in Europe. PLoS One 11(3):1–14
Romieu I, Sienra-Monge JJ, Ramírez-Aguilar M, Téllez-Rojo MM, Moreno-Macías H, Reyes-Ruiz NI, Del Río-Navarro BE, Ruiz-Navarro MX, Hatch G, Slade R, Hernández-Avila M (2002) Antioxidant supplementation and lung functions among children with asthma exposed to high levels of air pollutants. Am J Respir Crit Care Med 166(5):703–709
Ruparathna R, Hewage K, Sadiq R (2017) Rethinking investment planning and optimizing net zero emission buildings. Clean Techn Environ Policy 19(6):1711–1724
Sabri MF, Danapalasingam KA, Rahmat MF (2016) A review on hybrid electric vehicles architecture and energy management strategies. Renew Sust Energ Rev 53:1433–1442
Salehi KH, Sofia D, Barletta D, Poletto M (2015) Dust generation in vibrated cohesive powders. Chem Eng Trans 43(1):769–774
Sarigiannis DA, Kontoroupis P, Nikolaki S, Gotti A, Chapizanis D, Karakitsios S (2017) Benefits on public health from transport-related greenhouse gas mitigation policies in southeastern European cities. Sci Total Environ 579:1427–1438
Scheepers CE, Wendel-Vos GCW, den Broeder JM, van Kempen EEMM, van Wesemael PJV, Schuit AJ (2014) Shifting from car to active transport: a systematic review of the effectiveness of interventions. Transp Res A Policy Pract 70:264–280
Sellitto MA, Borchardt M, Pereira GM, Bubicz ME (2015) Tool for environmental performance assessment of city bus transit operations: case studies. Clean Techn Environ Policy 17(4):1053–1064
Settimo G (2015) Existing guidelines for indoor air quality: the case study of hospital environments. Indoor Air Qual Healthc Facilities:13–27
Shrestha RM, Shakya SR (2012) Benefits of low carbon development in a developing country: case of Nepal. Energy Econ 34(Suppl. 3):S503–S512
Singla S, Bansal D, Misra A (2018) Towards an integrated framework for air quality monitoring and exposure estimation — a review. Environ Monit Assess 190(562)
Sofia D, Joshi YA, Poletto M (2013) Kinetics of bioethanol production from lactose converted by Kluyveromyces Marxianus. Chem Eng Trans 32:1135–1140
Sofia D, Giuliano A, Gioiella F (2018a) Air quality monitoring network for tracking pollutants: the CASE study of Salerno city center. Chem Eng Trans 68:67–72
Sofia D, Giuliano A, Gioiella F, Barletta D, Poletto M (2018b) Modeling of an air quality monitoring network with high space-time resolution. Comput Aided Chem Eng 48:193–198
Sripada PP, Xu T, Kibria MA, Bhattacharya S (2017) Comparison of entrained flow gasification behaviour of Victorian Brown coal and biomass. Fuel 203:942–953
Stabile L, Jayaratne ER, Buonanno G, Morawska L (2014) Charged particles and cluster ions produced during cooking activities. Sci Total Environ 497(498):516–526
Stabile L, Buonanno G, Avino P, Frattolillo A, Guerriero E (2018) Indoor exposure to particles emitted by biomass-burning heating systems and evaluation of dose and lung cancer risk received by population. Environ Pollut 235:65–73
Tong Z, Baldauf RW, Isakov V, Deshmukh P, Max Zhang K (2016) Roadside vegetation barrier designs to mitigate near-road air pollution impacts. Sci Total Environ 541:920–927
Velthof GL, van Bruggen C, Groenestein CM, de Haan BJ, Hoogeveen MW, Huijsmans JFM (2012) A model for inventory of ammonia emissions from agriculture in the Netherlands. Atmos Environ 46:248–255
Venkataraman C, Sagar AD, Habib G, Lam N, Smith KR (2010) The Indian National Initiative for advanced biomass cookstoves: the benefits of clean combustion. Energy Sustain Dev 14(2):63–72
Vicente B, Rafael S, Rodrigues V, Relvas H, Vilaça M, Teixeira J, Bandeira J, Coelho M, Borrego C (2018) Influence of different complexity levels of road traffic models on air quality modelling at street scale. Air Qual Atmos and Health 11:1217–1232
Vlachokostas C, Achillas C, Moussiopoulos N, Banias G (2011) Multicriteria methodological approach to manage urban air pollution. Atmos Environ 45(25):4160–4169
Vranckx S, Lefebvre W, van Poppel M, Beckx C, Theunis J (2015) Air quality impact of intelligent transportation system actions used in a decision support system for adaptive traffic management. Int J Environ Pollut 57(3/4):133–145
Werner M, Kryza M, Dore AJ (2015) Mean annual population exposure to atmospheric particulate matter in Poland. Int J Environ Pollut 58(1–2):89–99
Woodcock J, Edwards P, Tonne C, Armstrong BG, Ashiru O, Banister D, Beevers S, Chalabi Z, Chowdhury Z, Cohen A, Franco OH, Haines A, Hickman R, Lindsay G, Mittal I, Mohan D, Tiwari G, Woodward A, Roberts I (2009) Public health benefits of strategies to reduce greenhouse-gas emissions: urban land transport. Lancet 374(9705):1930–1943
Wu Y, Zhang S, Hao J, Liu H, Wu X, Hu J, Walsh MP, Wallington TJ, Zhang KM, Stevanovic S (2017) On-road vehicle emissions and their control in China: a review and outlook. Sci Total Environ 574:332–349
Xia T, Nitschke M, Zhang Y, Shah P, Crabb S, Hansen A (2015) Traffic-related air pollution and health co-benefits of alternative transport in Adelaide, South Australia. Environ Int 74:281–290
Yang C, Zhao W, Deng K, Zhou V, Zhou X (2017) The association between air pollutants and autism spectrum disorders. Environ Sci Pollut Res 24:15949–15958
Younger M, Morrow-Almeida HR, Vindigni SM, Dannenberg AL (2008) The built environment, climate change, and health. Opportunities for co-benefits. Am J Prev Med 35(5):517–526
Zanobetti A, Schwartz J (2005) The effect of particulate air pollution on emergency admissions for myocardial infarction: a multicity Case-crossover analysis. Environ Health Perspect 113(8):978–982
Zhao ZQ, Bai ZH, Winiwarter W, Kiesewetter G, Heyes C, Ma L (2017) Mitigating ammonia emission from agriculture reduces PM2.5 pollution in the Hai River basin in China. Sci Total Environ 609:1152–1160
Download references
The authors would like to thank the Regione Campania with grant no. B52C18000150007 for their financial support.
Author information
Authors and affiliations.
Sense Square srl, Piazza Vittorio Emanuele 11, 84084, Fisciano, SA, Italy
Daniele Sofia, Filomena Gioiella, Nicoletta Lotrecchiano & Aristide Giuliano
University of Salerno, Via Giovanni Paolo II 132, 84084, Fisciano, SA, Italy
Daniele Sofia & Nicoletta Lotrecchiano
ENEA, Italian National Agency for New Technologies, Energy and Sustainable Economic Development, S.S. 106 Ionica, Rotondella, MT, Italy
Aristide Giuliano
You can also search for this author in PubMed Google Scholar
Corresponding author
Correspondence to Aristide Giuliano .
Additional information
Editorial Responsibility: Philippe Garrigues
Publisher’s note
Springer Nature remains neutral with regard to jurisdictional claims in published maps and institutional affiliations.
Rights and permissions
Reprints and permissions
About this article
Sofia, D., Gioiella, F., Lotrecchiano, N. et al. Mitigation strategies for reducing air pollution. Environ Sci Pollut Res 27 , 19226–19235 (2020). https://doi.org/10.1007/s11356-020-08647-x
Download citation
Received : 06 December 2019
Accepted : 27 March 2020
Published : 11 April 2020
Issue Date : June 2020
DOI : https://doi.org/10.1007/s11356-020-08647-x
Share this article
Anyone you share the following link with will be able to read this content:
Sorry, a shareable link is not currently available for this article.
Provided by the Springer Nature SharedIt content-sharing initiative
- Air pollution
- Mitigation strategies
- Human health
- Environmental impact
- Air quality
- Find a journal
- Publish with us
- Track your research
Information
- Author Services
Initiatives
You are accessing a machine-readable page. In order to be human-readable, please install an RSS reader.
All articles published by MDPI are made immediately available worldwide under an open access license. No special permission is required to reuse all or part of the article published by MDPI, including figures and tables. For articles published under an open access Creative Common CC BY license, any part of the article may be reused without permission provided that the original article is clearly cited. For more information, please refer to https://www.mdpi.com/openaccess .
Feature papers represent the most advanced research with significant potential for high impact in the field. A Feature Paper should be a substantial original Article that involves several techniques or approaches, provides an outlook for future research directions and describes possible research applications.
Feature papers are submitted upon individual invitation or recommendation by the scientific editors and must receive positive feedback from the reviewers.
Editor’s Choice articles are based on recommendations by the scientific editors of MDPI journals from around the world. Editors select a small number of articles recently published in the journal that they believe will be particularly interesting to readers, or important in the respective research area. The aim is to provide a snapshot of some of the most exciting work published in the various research areas of the journal.
Original Submission Date Received: .
- Active Journals
- Find a Journal
- Proceedings Series
- For Authors
- For Reviewers
- For Editors
- For Librarians
- For Publishers
- For Societies
- For Conference Organizers
- Open Access Policy
- Institutional Open Access Program
- Special Issues Guidelines
- Editorial Process
- Research and Publication Ethics
- Article Processing Charges
- Testimonials
- Preprints.org
- SciProfiles
- Encyclopedia
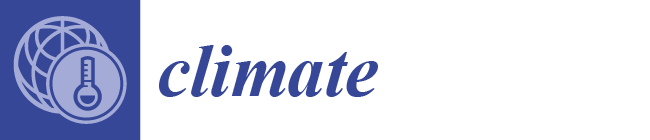
Article Menu
- Subscribe SciFeed
- Recommended Articles
- Google Scholar
- on Google Scholar
- Table of Contents
Find support for a specific problem in the support section of our website.
Please let us know what you think of our products and services.
Visit our dedicated information section to learn more about MDPI.
JSmol Viewer
Air pollution and human health in kolkata, india: a case study.
1. Introduction
2. study area, 2.1 sources of air pollution in kolkata, 3. database and methodology, 3.1. monitoring stations and criteria pollutants, 3.2. air quality assessment.
- Critical pollution (C): when EF is more than 1.5;
- High pollution (H): when the EF is between 1.0–1.5;
- Moderate pollution (M): when the EF between 0.5–1.0; and
- Low pollution (L): when the EF is less than 0.5.
3.3. Health Assessment
3.4. data analysis, 4. results and discussion, 4.1. concentration and trends of ambient air quality, 4.2. interpreting health outcomes of surveyed dispensaries in kolkata, 4.3. outdoor pollution-averting activities, 4.4. diseases analysis, 5. conclusions, acknowledgments, author contributions, conflicts of interest.
- Ghosh, S.; Maji, T. An environmental assessment of urban drainage, sewage and solid waste management in Bardhhaman Municipality, West Bengal. Int. J. Environ. Sci. 2011 , 2 , 92–105. [ Google Scholar ]
- Kumar, B.; Singh, R.B. Urban Development and Anthropogenic Climate Change: Experience in Indian Metropolitan Cities ; Manak Publication Pvt. Ltd.: New Delhi, India, 2003. [ Google Scholar ]
- Sudhir, K.S.; Kumar, S. India’s urban environment: Air/water pollution and pollution abatement. EPW 2013 , 48 , 22–25. [ Google Scholar ]
- Gupta, R.C. Environmental and infrastructural sustainability: Major challenges facing Indian metropolitan cities. In Sustainable Urban Development ; Singh, R.B., Ed.; Concept Publishing Company: New Delhi, India, 2006; pp. 3–11. [ Google Scholar ]
- Singh, R.B.; Mishra, D.K. Slums, environment and development in metropolitan cities of India. In Sustainable Urban Development ; Singh, R.B., Ed.; Concept Publishing Company: New Delhi, India, 2006; pp. 261–271. [ Google Scholar ]
- De, J. Development, environment and urban health in India. Geography 2007 , 92 , 158–160. [ Google Scholar ]
- Sharma, A.R.; Kharol, S.K.; Badrinath, K.V.S. Influence of vehicular traffic on urban air quality: A case study of Hyderabad, India. Trans. Res. 2010 , 15 , 154–159. [ Google Scholar ] [ CrossRef ]
- Singh, R.B.; Haque, S.; Grover, A. Drinking water, sanitation and health in Kolkata metropolitan city: Contribution towards urban sustainability. Geogr. Environ. Sustain. 2015 , 8 , 64–81. [ Google Scholar ] [ CrossRef ]
- UNEP. Environmental Threats to Children: Children in the New Millennium. United Nations Environmental Programme ; UNICEF; WHO: Geneva, Switzerland, 2002. [ Google Scholar ]
- Bates, D.V. Respiratory Function in Diseases ; WB Saunders: Philadelphia, PA, USA, 1992. [ Google Scholar ]
- Dockery, D.K.; Arden, P. Acute respiratory effects of particulate air pollution. Annu. Rev. Public Health 1994 , 15 , 107–113. [ Google Scholar ] [ CrossRef ] [ PubMed ]
- WHO. The World Health Report 2002—Reducing Risks, Promoting Healthy Life ; World Health Organization: Geneva, Switzerland, 2002. [ Google Scholar ]
- Bendahmane, D.B. Air Pollution and Child Health: Priorities for Action ; U.S. Agency for International Development: Washington, DC, USA, 1997.
- Intergovernmental Panel on Climate Change (IPCC). Climate Change 2007: Impacts Adaptation and Vulnerability ; Cambridge University Press: Cambridge, UK, 2007. [ Google Scholar ]
- Albuquerque, P.C.; Gomes, J.F.; Bordado, J.C. Assessment of exposure to airborne ultrafine particles in the urban environment of Lisbon, Portugal. J. Air Waste Manag. Assoc. 2012 , 64 , 373–380. [ Google Scholar ] [ CrossRef ]
- Gurjar, B.R.; Butler, T.M.; Lawrence, M.G.; Leliveld, J. Evaluation of emissions and air quality in megacities. Atmos. Environ. 2007 , 42 , 1593–1606. [ Google Scholar ] [ CrossRef ]
- Faiz, A.; Sturm, P.J. New dimensions: Air pollution and road traffic in developing countries. Atmos. Environ. 2000 , 34 , 4745–4746. [ Google Scholar ]
- Brashier, B.; Londhe, J.; Madas, S.; Vincent, V.; Salvi, S. Prevalence of self-reported respiratory symptoms, asthma and chronic bronchitis in slum area of a rapidly developing Indian city. Sci. Res. 2012 , 2 , 73–81. [ Google Scholar ]
- WHO. World Health Statistic ; World Health Organization: Geneva, Switzerland, 2008. [ Google Scholar ]
- Dincer, I. Renewable energy and sustainable development: A critical review. Renew. Sustain. Energy Rev. 2000 , 4 , 157–175. [ Google Scholar ] [ CrossRef ]
- Hasselblad, V.; Kotchmar, D.J.; Eddy, D.M. Synthesis of environmental evidence: Nitrogen dioxide epidemiological studies. J. Air Waste Manag. Assoc. 1992 , 42 , 662–671. [ Google Scholar ] [ CrossRef ]
- Saldiva, P.H.N.; Lichtenfels, A.J.R.C.; Paiva, P.S. Association between air pollution and mortality due to respiratory diseases in children in Sao Paulo, Brazil: A preliminary report. Environ. Res. 1994 , 65 , 218–225. [ Google Scholar ] [ CrossRef ]
- WHO. Health Aspects of Air Pollution with Particulate Matter, Ozone and Nitrogen Dioxide, Report on a WHO Working Group ; WHO Regional Office for Europe: Copenhagen, Denmark, 2003. [ Google Scholar ]
- Smith, K.R. How much global ill health is attributable to environmental factors? Epidemiology 1999 , 5 , 573–584. [ Google Scholar ] [ CrossRef ]
- Martin, M.C.H.; Fatigati, F.L.; Vespoli, T.C.; Martins, L.C.; Pereira, L.A.A.; Martins, M.A.; Saldiva, P.H.N.; Braga, A.L.F. Influence of socioeconomic conditions on air pollution: Adverse health effects in elderly people: An analysis of six regions. J. Epidemiol. Community Health 2004 , 58 , 41–46. [ Google Scholar ] [ CrossRef ]
- Gouveia, N.; Fletcher, T. Time series analysis of air pollution and mortality: Effects by cause, age and socioeconomic status. J. Epidemiol. Community Health 2000 , 54 , 750–755. [ Google Scholar ] [ CrossRef ] [ PubMed ]
- Stern, R.E. Hong Kong haze: Air pollution as a social class issue. Asian Surv. 2003 , 43 , 780. [ Google Scholar ] [ CrossRef ]
- Suri, S.N.; Birch, E. India and the sustainable cities goal. EPW 2014 , 49 , 26–28. [ Google Scholar ]
- Kumar, K.S.K. Economics of sustainable development. EPW 2016 , 51 , 34–36. [ Google Scholar ]
- Singh, R.B. Urban sustainability, health and wellbeing and disaster risk reduction. Professor R.N. Dubey memorial lectures-2015. Presented at Professor R.N. Dubey Foundation, Allahabad, India, 15 September 2016. [ Google Scholar ]
- UN-Habitat and UN-ESCAP. The State of Asian and Pacific Cities 2015 ; UN-Habitat: Yangon, Myanmar, 2016. [ Google Scholar ]
- Registrar General & Census Commissioner. Census of India. Provisional Population Totals ; Registrar General & Census Commissioner: Delhi, India, 2011. [ Google Scholar ]
- Ghose, M.K.; Paul, R.; Banerjee, S.K. Assessment of the impact on human health of exposure to urban air pollutants: An Indian case study. Int. J. Environ. Stud. 2007 , 62 , 201–214. [ Google Scholar ] [ CrossRef ]
- Anon. Report of the Committee Constituted by the order of the Honourable High Court, Calcutta for Recommending measures to check the pollution in the city of Calcutta. In Health Effects of Air Pollution: A study of Kolkata ; Dept. of Environment, Govt. of West Bengal and West Bengal Pollution Control Board: Kolkata, India, 2002. [ Google Scholar ]
- Singh, R.B.; Haque, S. Urban ambient air quality and respiratory health in Kolkata: A dispensary level analysis. J. Urban. Reg. Stud. 2016 , 2 , 7–21. [ Google Scholar ]
- Bhaumik, S. Air Pollution Suffocates Calcutta. BBC News. Available online: http://news.bbc.co.uk/2/hi/south_asia/6614561.stm (accessed on 3 May 2007).
- Mukhopadhyay, K. Air Pollution in India and Its Impact on the Health of Different Income Groups ; Nova Science Publishers, Inc.: New York, NY, USA, 2009. [ Google Scholar ]
- Ghose, M.K. Controlling of motor vehicle emissions for a sustainable city. TIDEE 2002 , 2 , 273–282. [ Google Scholar ]
- Schwela, W.; Haq, G.; Huizenga, C.; Han, W.; Fabian, H.; Ajero, M. Urban Air Pollution in Asian Cities Status, Challenges and Management ; Earthscan Publishers: London, UK, 2006. [ Google Scholar ]
- Lahiri, T.; Roy, S.; Ganguly, S.; Ray, M.R.; Lahiri, P. Air pollution in Calcutta elicits adverse pulmonary reaction in children. Ind. J. Med. Res. 2000 , 112 , 21–26. [ Google Scholar ]
- Roy, S.; Ray, M.R.; Basu, C.; Lahiri, P.; Lahiri, T. Abundance of siderophages in sputum: Indicator of an adverse lung reaction to air pollution. Actayologica 2006 , 45 , 958–964. [ Google Scholar ] [ CrossRef ]
- West Bengal Pollution Control Board. A Quinqueenniel Report, April 1998 to March 2003 ; West Bengal Pollution Control Board: Kolkata, India, 2003. [ Google Scholar ]
- Mondol, R.; Sen, G.K.; Chatterjee, M.; Sen, B.K.; Sen, S. Ground-level concentration of nitrogen oxides (NOx) at some traffic intersection points in Calcutta. In Air Pollution in Kolkata: An Analysis of Current Status and Interrelation between Different Factors ; SEEU Review: Tetovo, Macedonia, 2013; Volume 8, pp. 181–214. [ Google Scholar ]
- Ghose, K.M.; Paul, R.; Banerjee, S.K. Assessment of the impacts of vehicular emissions on urban air quality and its management in Indian context: The case of Kolkata (Calcutta). Environ. Sci. Policy 2004 , 7 , 345–351. [ Google Scholar ] [ CrossRef ]
- Mukherjee, A.; Mukherjee, G. Occupational exposure of the traffic personnel of Calcutta of lead and carbon monoxide. In Air Pollution in Kolkata: An Analysis of Current Status and Interrelation between Different Factors ; SEEU Review: Tetovo, Macedonia, 2013; Volume 8, pp. 181–214. [ Google Scholar ]
- Kazimuddin, A.; Banerjee, L. Fighting for Air. Available online: http://www.downtoearth.org.in/coverage/fighting-for-air-18428 (accessed on 31 July 2000).
- Ghose, M.K. Air pollution in the city of Kolkata: Health effects due to chronic exposure. In Air Pollution in Kolkata: An Analysis of Current Status and Interrelation between Different Factors ; SEEU Review: Tetovo, Macedonia, 2013; Volume 8, pp. 181–214. [ Google Scholar ]
- West Bengal Pollution Control Board. Air Quality Management: Final Report. WBPCB in Collaboration with Asian Development Bank ; Intercontinental Consultant and Technocrats Pvt. Ltd.: New Delhi, India, 2005. [ Google Scholar ]
- West Bengal Pollution Control Board. Annual Report 2008–2010 ; Government of West Bengal: Kolkata, India, 2010. [ Google Scholar ]
- Government of West Bengal. “We Care for You”, Annual Report, Kolkata Traffic Police ; Government of West Bengal: Kolkata, India, 2012.
- Bhaduri, S. Vehicular growth and air quality at major traffic intersection points in Kolkata: An efficient intervention strategies. Stand. Int. J. 2013 , 1 , 19–25. [ Google Scholar ]
- Government of West Bengal. “We Care for You”, Annual Report, Kolkata Traffic Police ; Government of West Bengal: Kolkata, India, 2013.
- Central Pollution Control Board. Guidelines for Ambient Air Quality Monitoring. National Ambient Air Quality Monitoring Series ; CPCB, Ministry of Environment and Forest, Government of India: Delhi, India, 2003.
- West Bengal Pollution Control Board. Annual Report 2010–2011 ; Government of West Bengal: Kolkata, India, 2011.
Source Types | Emissions (Tonnes/Year) | Totals | % RPM | % NOx | % SO | % Total | ||
---|---|---|---|---|---|---|---|---|
RPM | NOx | SO | ||||||
Motor Vehicles | 16,115 | 95,452 | 0 | 7.4 | 44.0 | 0 | ||
Industry | 6571 | 34,208 | 12,378 | 3.0 | 15.8 | 5.7 | ||
Road Dust | 45,881 | 0 | 0 | 21.1 | 0 | 0 | ||
Area Sources | 6573 | 0 | 0 | 3.0 | 0 | 0 | ||
Sl. No. | Months | Monthly Average Concentration (μg/m ) | |||
---|---|---|---|---|---|
SO | NO | RPM | SPM | ||
1 | 10 April | 7.6 | 50.2 | 45 | 117 |
2 | 10 May | 5.4 | 42.3 | 35 | 96 |
3 | 10 June | 5.0 | 43.8 | 34 | 90 |
4 | 10 July | 4.4 | 39 | 28 | 77 |
5 | 10 August | 4.2 | 38.3 | 28 | 75 |
6 | 10 September | 4.4 | 37.1 | 34 | 88 |
7 | 10 October | 6.1 | 49.3 | 63 | 155 |
8 | 10 November | 7.9 | 65.8 | 127 | 265 |
9 | 10 December | 9.9 | 78.9 | 179 | 342 |
10 | 11 January | 9.2 | 94 | 211 | - |
11 | 11 February | 8.2 | 79.7 | 172 | - |
12 | 11 March | 5.5 | 59.7 | 96 | - |
Sl. No. | Monitoring Stations | Air Pollutants, Annual Concentration and Pollution Level | ||||||||
---|---|---|---|---|---|---|---|---|---|---|
SO | NO | RPM | ||||||||
Annual Average (μg/m ) | Value of E.F * | Air Quality ** | Annual Average (μg/m ) | Value of E.F * | Air Quality ** | Annual Average (μg/m ) | Value of E.F * | Air Quality ** | ||
1 | Dunlop Station | 7.9 | 0.1 | L | 67.2 | 1.6 | C | 108 | 1.8 | C |
2 | Picnic Garden | 5.6 | 0.1 | L | 48.9 | 1.2 | H | 73 | 1.2 | H |
3 | Tollygunge | 6.7 | 0.1 | L | 57.2 | 1.4 | H | 81 | 1.3 | H |
4 | Hyde Road | 6.5 | 0.1 | L | 58.1 | 1.4 | H | 92 | 1.5 | H |
5 | Behala Chowrasta | 7.8 | 0.1 | L | 68.0 | 1.7 | C | 97 | 1.6 | C |
6 | Beliaghata | 5.8 | 0.1 | L | 54.0 | 1.3 | H | 80 | 1.3 | H |
7 | Salt Lake | 6.5 | 0.1 | L | 57.8 | 1.4 | H | 87 | 1.4 | H |
8 | Topsia | 5.6 | 0.1 | L | 51.4 | 1.2 | H | 74 | 1.2 | H |
9 | Baishanabghata | 5.6 | 0.1 | L | 51.0 | 1.2 | H | 86 | 1.4 | H |
10 | Ultadanga | 7.1 | 0.1 | L | 62.1 | 1.6 | C | 92 | 1.5 | H |
11 | Mominpore | 6.0 | 0.1 | L | 53.8 | 1.3 | H | 85 | 1.4 | H |
12 | Moulali | 8.2 | 0.1 | L | 70.7 | 1.7 | C | 107 | 1.7 | C |
13 | Shyambazar | 7.4 | 0.1 | L | 60.8 | 1.5 | C | 90 | 1.5 | H |
14 | Gariahat | 5.9 | 0.1 | L | 51.0 | 1.2 | H | 78 | 1.3 | H |
15 | Minto Park | 6.8 | 0.1 | L | 58.0 | 1.4 | H | 70 | 1.2 | H |
16 | Rajarhat | 5.5 | 0.1 | L | 47.5 | 1.1 | H | 79 | 1.3 | H |
17 | Paribesh Bhawan | 5.4 | 0.1 | L | 43.1 | 1.0 | H | 113 | 1.9 | C |
Name of the Dispensaries | Ward Number | Respondents | % Slum and Non-Slum | |
---|---|---|---|---|
Slum | Non-Slum | |||
Ultadanga Dispensary | 14 | 28 | 82.1 | 17.9 |
Tangra Dispensary | 57 | 43 | 86.2 | 13.8 |
Behala Dispensary | 121 | 29 | 79.1 | 20.9 |
Average | 82.5 | 17.5 | ||
Total | 100 | 100 |
Name of the Dispensary | % Cooking Inside the Living Room | % Cooking Outside the Living Room | Total | ||||||
---|---|---|---|---|---|---|---|---|---|
Firewood | Coal | Kerosene | LPG | Firewood | Coal | Kerosene | LPG | ||
Ultadanga Dispensary | - | - | 28.0 | 40.0 | 15.7 | 3.1 | 6.3 | 6.3 | 100 |
Behala Dispensary | - | 2.6 | 46.1 | 20.5 | 25.6 | - | - | 5.1 | 100 |
Tangra Dispensary | 2.0 | 4.1 | 38.8 | 30.6 | 14.3 | - | 4.1 | 6.1 | 100 |
Average | 0.6 | 2.2 | 37.6 | 30.3 | 18.5 | 1 | 3.5 | 5.8 | 100 |
Total | 71 | 29 | 100 |
Outdoor Pollution Averting Activities | % Share of the Respondents at Dispensaries | |||||
---|---|---|---|---|---|---|
Ultadanga Dispensary | Tangra Dispensary | Behala Dispensary | ||||
Yes | No | Yes | No | Yes | No | |
Prefer to Stay Indoor | 3.60 | 96.4 | 2.00 | 98.0 | 00.0 | 100 |
Using Mask While Walking on the Road | 10.7 | 89.3 | 28.0 | 72.0 | 28.0 | 72.0 |
Avoiding Busy Road and Busy Timing | 25.0 | 75.0 | 28.0 | 72.0 | 48.0 | 52.0 |
Avoiding Landfill/Garbage Disposal Site | 71.4 | 28.6 | 67.0 | 33.0 | 90.0 | 10.0 |
Outdoor Pollution has Affected Health | 39.3 | 60.7 | 44.0 | 56.0 | 38.0 | 62.0 |
Name of the Dispensary | % Respiratory Diseases | Total | % Waterborne Diseases | Total | |||||
---|---|---|---|---|---|---|---|---|---|
ARI | COPD | Influenza | UTRI | AFB | Diarrhoea | Ringworm | |||
Ultadanga Dispensary | 21.4 | 10.7 | 35.7 | 3.6 | - | 71.4 | 25.0 | 3.6 | 28.6 |
Behala Dispensary | 72.4 | 10.3 | - | - | 10.3 | 93.1 | 6.9 | - | 6.9 |
Tangra Dispensary | 86.1 | 2.3 | 2.3 | - | - | 90.9 | 9.3 | - | 9.1 |
Average | 60.0 | 7.8 | 12.7 | 1.2 | 3.4 | 85.1 | 13.7 | 1.2 | 14.9 |
85.1 | 14.9 | ||||||||
Total | 100 |
Share and Cite
Haque, M.S.; Singh, R.B. Air Pollution and Human Health in Kolkata, India: A Case Study. Climate 2017 , 5 , 77. https://doi.org/10.3390/cli5040077
Haque MS, Singh RB. Air Pollution and Human Health in Kolkata, India: A Case Study. Climate . 2017; 5(4):77. https://doi.org/10.3390/cli5040077
Haque, Md. Senaul, and R. B. Singh. 2017. "Air Pollution and Human Health in Kolkata, India: A Case Study" Climate 5, no. 4: 77. https://doi.org/10.3390/cli5040077
Article Metrics
Article access statistics, further information, mdpi initiatives, follow mdpi.
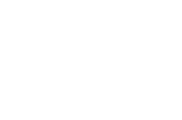
Subscribe to receive issue release notifications and newsletters from MDPI journals

An official website of the United States government
The .gov means it’s official. Federal government websites often end in .gov or .mil. Before sharing sensitive information, make sure you’re on a federal government site.
The site is secure. The https:// ensures that you are connecting to the official website and that any information you provide is encrypted and transmitted securely.
- Publications
- Account settings
- My Bibliography
- Collections
- Citation manager
Save citation to file
Email citation, add to collections.
- Create a new collection
- Add to an existing collection
Add to My Bibliography
Your saved search, create a file for external citation management software, your rss feed.
- Search in PubMed
- Search in NLM Catalog
- Add to Search
Thermal power plants pollution assessment based on deep neural networks, remote sensing, and GIS: A real case study in Iran
Affiliations.
- 1 Department of Geography, Faculty of Economics, Management and Social Science, Shiraz University, Shiraz, Iran.
- 2 College of Mechatronics and Control Engineering, Shenzhen University, Shenzhen 518060, China.
- 3 Department of Electrical Engineering Fundamentals, Faculty of Electrical Engineering, Wroclaw University of Science and Technology, Wroclaw, Poland. Electronic address: [email protected].
- PMID: 37263027
- DOI: 10.1016/j.marpolbul.2023.115069
To investigate the impact of the Bandar Abbas thermal power plant on the waters of the Persian Gulf coast, a combination of satellite images and ground data was utilized to determine the Sea Surface Temperature (SST) as a thermal index, Total Organic Carbon (TOC) and Chemical Oxygen Demand (COD) as biological indices. Additionally, measurements of SO 2 , O 3 , NO 2 , CO 2 , CO, and CH 4 values in the atmosphere were taken to determine the plant's impact on air pollution. Temperature values of the water for different months were predicted using Long Short-Term Memory (LSTM), Support Vector Regression (SVR), and Cascade neural networks. The results indicate that the waters near thermal power plants exhibit the highest temperatures in July and September, with temperatures reaching approximately 50 °C. Furthermore, the SST values were found to be strongly correlated with ecological indices. The Multiple Linear Regression (MLR) analysis revealed a strong correlation between the temperature and TOC, COD, and O 2 in water (R TOC 2 =0.98), [Formula: see text] , R COD 2 =0.87 and O 3 , NO 3 , CO 2 , and CO in the air ( [Formula: see text] ). Finally, the results demonstrate that the LSTM method exhibited high accuracy in predicting the water temperature (R 2 = 0.98).
Keywords: Air pollution; Bandar Abbas thermal power plant; Long Short-Term Memory (LSTM); Oxygen levels; Remote sensing; Thermal pollution.
Copyright © 2023 Elsevier Ltd. All rights reserved.
PubMed Disclaimer
Conflict of interest statement
Declaration of competing interest The authors declare that they have no known competing financial interests or personal relationships that could have appeared to influence the work reported in this paper.
Similar articles
- Using neural networks and remote sensing for spatio-temporal prediction of air pollution during the COVID-19 pandemic. Mokarram M, Taripanah F, Pham TM. Mokarram M, et al. Environ Sci Pollut Res Int. 2023 Dec;30(58):122886-122905. doi: 10.1007/s11356-023-30859-0. Epub 2023 Nov 18. Environ Sci Pollut Res Int. 2023. PMID: 37979107
- Investigating Patterns of Air Pollution in Metropolises Using Remote Sensing and Neural Networks During the COVID-19 Pandemic. Shaygan M, Mokarram M. Shaygan M, et al. Adv Space Res. 2023 Jun 21. doi: 10.1016/j.asr.2023.06.027. Online ahead of print. Adv Space Res. 2023. PMID: 37361684 Free PMC article.
- Long-term remote sensing-based methods for monitoring air pollution and cloud cover in the Balkan countries. Valjarević A. Valjarević A. Environ Sci Pollut Res Int. 2024 Apr;31(18):27155-27171. doi: 10.1007/s11356-024-32982-y. Epub 2024 Mar 20. Environ Sci Pollut Res Int. 2024. PMID: 38509311
- Assessment and statistical modeling of the relationship between remotely sensed aerosol optical depth and PM2.5 in the eastern United States. Paciorek CJ, Liu Y; HEI Health Review Committee. Paciorek CJ, et al. Res Rep Health Eff Inst. 2012 May;(167):5-83; discussion 85-91. Res Rep Health Eff Inst. 2012. PMID: 22838153
- The oxycoal process with cryogenic oxygen supply. Kather A, Scheffknecht G. Kather A, et al. Naturwissenschaften. 2009 Sep;96(9):993-1010. doi: 10.1007/s00114-009-0557-2. Epub 2009 Jun 4. Naturwissenschaften. 2009. PMID: 19495717 Free PMC article. Review.
- Research Progress in Fluid Energy Collection Based on Friction Nanogenerators. Yan J, Sheng Y, Zhang D, Tang Z. Yan J, et al. Micromachines (Basel). 2023 Dec 24;15(1):40. doi: 10.3390/mi15010040. Micromachines (Basel). 2023. PMID: 38258159 Free PMC article. Review.
- Search in MeSH
Related information
- PubChem Compound (MeSH Keyword)
LinkOut - more resources
Full text sources.
- Elsevier Science
- MedlinePlus Health Information
- Citation Manager
NCBI Literature Resources
MeSH PMC Bookshelf Disclaimer
The PubMed wordmark and PubMed logo are registered trademarks of the U.S. Department of Health and Human Services (HHS). Unauthorized use of these marks is strictly prohibited.
Ecological Questions
The impact assessment of thermal pollution on subtidal sessile assemblages: a case study from mediterranean rocky reefs.
- Stanislao Bevilacqua Deptartment of Life Sciences, University of Trieste, 34127 Trieste, Italy CoNiSMa, Piazzale Flaminio, 9, Roma, Italy
- Samsa Clara Deptartment of Life Sciences, University of Trieste, 34127 Trieste, Italy
- Antonio Terlizzi Deptartment of Life Sciences, University of Trieste, 34127 Trieste, Italy CoNiSMa, Piazzale Flaminio, 9, Roma, Italy Stazione Zoologica Anton Dohrn, 80121 Napoli, Italy
Coastal power plants generally use seawater as cooling fluid, discharging heated waters into the sea after the cooling process. The ensuing increase in seawater temperature could affect the marine biota in the nearby areas, causing alterations at different level of biological organization, from individuals to populations and assemblages. In the Mediterranean Sea, few attempts have been made to assess the effects of this point sources of thermal pollution, especially on rocky habitats. Here, we investigated the putative impact of a thermal effluent from one of the largest European coal-fired coastal power plants on sessile assemblages of subtidal rocky reefs. Sessile assemblages on rocky substrates were photographically sampled at one location near the effluent ( I ), and at two control locations ( Cs ) virtually unaffected by thermal discharge. An asymmetrical after-control impact experimental design was employed to test the hypothesis that the thermal discharge significantly modified sessile assemblages at I if compared to Cs . We detected significant differences in assemblages at I versus Cs , indicating a clear effect of the effluent on assemblage structure. Such differences were mostly due to shift in dominance among macroalgae between I and Cs , which likely depended on different tolerance limits of species to increased seawater temperature and other sources of disturbance associated to the effluent, such as increased sedimentation rates and water turbidity. Our findings stressed the need for further investigations of the impact of thermal effluents on marine communities, considering the potential synergistic effects of climate change especially in the Mediterranean Sea.
Airoldi L., 1998, Roles of disturbance, sediment stress, and substratum retention on spatial dominance in algal turf, Ecology 79: 2759–2770.
Anderson M.J., 2001, A new method for non-parametric multivariate analysis of variance, Austral Ecology 26: 32–46.
Anderson M.J., Gorley R.N., Clarke K.R., 2008, PERMANOVA+ for PRIMER: guide to software and statistical methods. PRIMER-E, Plymouth, UK.
Arieli R.N., Almogi-Labin A., Abramovich S., Herut B., 2011, The effect of thermal pollution on benthic foraminiferal assemblages in the Mediterranean shoreface adjacent to Hadera power plant (Israel), Marine Pollution Bulletin 62: 1002–1012.
Baker P., Fajans J.S., Arnold W.S., Ingrao D.A., Marell D.C., Baker S.M., 2007, Range and dispersal of a tropical marine invader, the asian green mussel, Perna viridis, in subtropical waters of the southeastern United States, Journal of Shellifish Research 26: 345–355.
Bamber R.N., 1990, Power station thermal effluents and marine crustaceans, Journal of Thermal Biology 15: 91–96.
Barnett P.R.O., 1972, Effects of Warm Water Effluents from Power Stations on Marine Life, Proceedings of the Royal Society of London. Series B, Biological Sciences 180: 497–509.
Bevilacqua S., Katsanevakis S., Micheli F., Sala E., Rilov G., Sarà G., Malak D.A., Abdulla A., Gerovasileiou V., Gissi E., Mazaris A.D., Pipitone C., Sini M., Stelzenmüller V., Terlizzi A., Todorova V., Fraschetti S., 2020, The status of coastal benthic ecosystems in the Mediterranean Sea: Evidence from ecological indicators. Frontiers in Marine Science 7: 475.
Bevilacqua S., Savonitto G., Lipizer M., Mancuso P., Ciriaco S., Srijemsi M., Falace A., 2019, Climatic anomalies may create a long‐lasting ecological phase shift by altering the reproduction of a foundation species, Ecology 100: e02838.
Bray J.R., Curtis J.T., 1957, An ordination of the upland forest communities of Southern Wisconsin, Ecological Monographs 27: 325–349.
Bush R.M., Welch E.B., Mar B.W., 1974, Potential effects of thermal discharges on aquatic systems, Environmental Science and Technology 6: 561–568.
Choi D.H., Park J.S., Hwang C.Y., Huh S.H., Cho B.C., 2002, Effects of thermal effluents from a power station on bacteria and heterotrophic nanoflagellates in coastal waters, Marine Ecology Progress Series 229: 1–10.
Choi H.-G., 2008, Effects of thermal effluents from Wolseong nuclear power plant on macroalgal composition and community structure, Algae 23: 151–162.
Clarke K.R., 1993, Nonparametric multivariate analyses of changes in community structure, Australian Journal of Ecology 18: 117–143.
Clarke K.R., Gorley R.N., 2015, PRIMER version 7: User manual/tutorial, PRIMER-E, Plymouth, UK.
Crema R., Bonvicini Pagliai A.M., 1980, The structure of benthic communities in an area of thermal discharge from a coastal power station, Marine Pollution Bulletin 11: 221–224.
Deabes E.A.M., 2020, The impact of thermal power stations on coastline and benthic fauna: Case study of El-Burullus power plant in Egypt, Results in Engineering 7: 100128.
Dethier M.N., Graham E.S., Cohen S., Tear L.M., 1993, Visual versus random-point percent cover estimation: ‘objective’ is not always better, Marine Ecology Progress Series 96: 93–100.
Dijkstra J.A., Litterer A., Mello K., O'Brien B.S., Rzhanov Y., 2019, Temperature, phenology, and turf macroalgae drive seascape change: Connections to mid‐trophic level species. Ecosphere 10: e02923.
Fairweather P.G., 1991, Statistical power and design requirements for environmental monitoring, Australian Journal of Marine and Freshwater Research 42: 555–568.
Flagella M.M, Lorenti M., Buia M.C., 2008, Temperature response in a shallow-water Mediterranean population of Caulerpa racemosa var. cylindracea (Caulerpales, Chlorophyta), and a possible strategy of season anticipation, Botanica Marina 51: 278–284.
Gaeta M.G., Samaras A.G., Archetti R., 2020, Numerical investigation of thermal discharge to coastal areas: A case study in South Italy. Environmental Modelling & Software 124: 104596.
Givan O., Edelist D., Sonin O., Belmaker J., 2018, Thermal affinity as the dominant factor changing Mediterranean fish abundances, Global Change Biology 24: 80–89.
Gray J.S., 1990, Statistics and the precautionary principle, Marine Pollution Bulletin 21:174–176.
Guarnieri G., Fraschetti S., Bogi C., Galil B.S., 2017, A hazardous place to live: spatial and temporal patterns of species introduction in a hot spot of biological invasions, Biological Invasion 19: 2277–2290.
Harvey B.P., Gwynn‐Jones D., Moore P.J., 2013, Meta‐analysis reveals complex marine biological responses to the interactive effects of ocean acidification and warming, Ecology and Evolution 3: 1016–1030.
Jiang Z., Zeng J., Chen Q., Huang Y., Xu X., Liao Y., Shou L., Liu J., 2009, Tolerance of copepods to short-term thermal stress caused by coastal power stations, Journal of Thermal Biology 33: 419–423.
Johnson M.D., Comeau S., Lantz C.A., Smith J.E., 2017, Complex and interactive effects of ocean acidification and temperature on epilithic and endolithic coral-reef turf algal assemblages. Coral Reefs 36: 1059–1070.
Katsanevakis S., Coll M., Piroddi C., Steenbeek J., Ben Rais Lasram F., Zenetos A., Cardoso A.C., 2014, Invading the Mediterranean Sea: biodiversity patterns shaped by human activities, Frontiers in Marine Science 1: 1–11.
Kim H.-G., Rho H.-S., Oh C.-W., 2013, Seasonal and spatial variations in nematode assemblages affected by thermal influence of nuclear power plant in Korea (East Sea, Pacific Ocean), Marine Biology Research 9: 725–738.
Langford T.E., 1990, Ecological Effects of Thermal Discharges, Elsevier, pp. 468.
Lejeusne C., Chevaldonné P., Pergent-Martini C., Boudouresque C.F., Pérez T., 2010, Climate change effects on a miniature ocean: the highly diverse, highly impacted Mediterranean Sea. Trends in Ecology and Evolution 25: 250–260.
Lin J., Zou X., Huang F., 2017, Effects of the thermal discharge from an offshore power plant on plankton and macrobenthic communities in subtropical China, Marine Pollution Bulletin 131: 106–114.
Lukšienė D., Sandström O., Lounasheimo L., Andersson J., 2000, The effects of thermal effluent exposure on the gametogenesis of female fish, Journal of Fish Biology 56: 37–50.
Mannino A., Balistreri P., Deidun A., 2017, The Marine Biodiversity of the Mediterranean Sea in a Changing Climate: The Impact of Biological Invasions, [in:] Mediterranean Identities - Environment, Society, Culture, F.-B. Borna (ed.), Intech Open, London.
Marano G., De Zio V., Pastorelli A.M., Rizzi E., Rositani L., Ungaro N., 2000, Effects of thermal discharge on marine ecosystems. A case study from a coastal power station in Southern Italy, Oebalia 26: 15–36.
Micheli F., Halpern B.S., Walbridge S., Ciriaco S., Ferretti F., Fraschetti S., Lewison R., Nykjaer L., Rosenberg A.A., 2013, Cumulative human impacts on Mediterranean and Black Sea, marine ecosystems: Assessing current pressures and opportunities. PLoS ONE 8: e79889.
Rilov G., Galil B., 2009, Marine bioinvasions in the Mediterranean Sea–history, distribution and ecology, [in:] Biological Invasions in Marine Ecosystems, G. Rilov & A.C. Crooks (eds), Springer, Berlin: 549–575.
Rivetti I., Fraschetti S., Lionello P., Zambianchi E., Boero F., 2014, Global warming and mass mortalities of benthic invertebrates in the Mediterranean Sea, PLoS ONE 9: e115655.
Roberts D.E., Fitzhenry S.R., Kennelly S.J., 1994, Quantifying subtidal macrobenthic assemblages on hard substrata using a jump camera method, Journal of Experimental Marine Biology and Ecology 177: 157–170.
Sala E., Kizilkaya Z., Yildirim D., Ballesteros E., 2011, Alien marine fishes deplete algal biomass in the eastern Mediterranean. PLoS ONE 6: e17356.
Samperio-Ramos G., Olsen Y.S., Tomas F., Marbà N., 2015, Ecophysiological responses of three Mediterranean invasive seaweeds (Acrothamnion preissii, Lophocladia lallemandii and Caulerpa cylindracea) to experimental warming, Marine Pollution Bulletin 96: 418–423.
Sanford E., 1999, Regulation of keystone predation by small changes in ocean temperature, Science 283: 2095–2097.
Savva I., Bennett S., Roca G., Jordà G., Marbà N., 2018, Thermal tolerance of Mediterranean marine macrophytes: Vulnerability to global warming, Ecology and Evolution 8: 12032–12043.
Strain E.M.A., Thomson R.J., Micheli F., Mancuso F.P., Airoldi L., 2014, Identifying the interacting roles of stressors in driving the global loss of canopy forming to mat-forming algae in marine ecosystems. Global Change Biology 20: 3300–3312.
Sylvester J.R., 1970, Possible effects of thermal effluents on fish: A review, Environmental Pollution 3: 205–215.
Teixeira T.P., Neves L.M., Araújo F.G., 2009, Effects of a nuclear power plant thermal discharge on habitat complexity and fish community structure in Ilha Grande Bay, Brazil, Marine Environmental Research 68: 188–195.
Teixeira T.P., Neves L.M., Araújo F.G., 2012, Thermal impact of a nuclear power plant in a coastal area in Southeastern Brazil: effects of heating and physical structure on benthic cover and fish communities, Hydrobiologia 684: 161–175.
Terlizzi A., Benedetti-Cecchi L., Bevilacqua S., Fraschetti S., Guidetti P., Anderson M.J., 2005, Multivariate and univariate asymmetrical analyses in environmental impact assessment: a case study of Mediterranean subtidal sessile assemblages, Marine Ecology Progress Series 289: 27–42.
Underwood A.J., 1994, On beyond BACI: sampling designs that might reliably detect environmental disturbances, Ecological Applications 4: 3–15.
Underwood A.J., 1997, Experiments in ecology: their logical design and interpretation using analysis of variances. Cambridge University Press, Cambridge.
Walther G.-R., Roques A., Hulme P.E., Sykes M.T., Pyšek P., Kühn I., Zobel M., Bacher S., Botta-Dukát Z., Bugmann H., Czúcz B., Dauber J., Hickler T., Jarošík V., Kenis M., Klotz S., Minchin D., Moora M., Nentwig W., Ott J., Panov V.E., Reineking B., Robinet C., Semenchenko V., Solarz W., Thuiller W., Vilà M., Vohland K., Settele J., 2009, Alien species in a warmer world: risks and opportunities, Trends in Ecology and Evolution 24: 686–693.
White D.P., Wahl D.H., 2020, Growth and physiological responses in largemouth bass populations to environmental warming: Effects of inhabiting chronically heated environments, Journal of Thermal Biology 88: 102467.
Wolf M.A., Sfriso A., Moro I., 2014, Thermal pollution and settlement of new tropical alien species: The case of Grateloupia yinggehaiensis (Rhodophyta) in the Venice Lagoon, Estuarine, Coastal and Shelf Science 147: 11–16.
World Power Plant Database, 2020, https://data.opendatasoft.com . Accessed Septembe, 20, 2020.
Yeruham E., Shpigel M., Abelson A., Rilov G., 2020, Ocean warming and tropical invaders erode the performance of a key herbivore, Ecology 101: e02925.
How to Cite
- Endnote/Zotero/Mendeley (RIS)
Number of views and downloads: 594 Number of citations: 2
- Browse Author Index
- Issue archive
Current Issue
Information.
- For Readers
- For Authors
- For Librarians
Search using one of provided tags:

- Let Me Explain
- Yen Endra Kelvi
- SUBSCRIBER ONLY
- Whats Your Ism?
- Pakka Politics
- NEWSLETTERS
Tamil Nadu’s Ennore is a case study in climate recklessness and environmental casteism
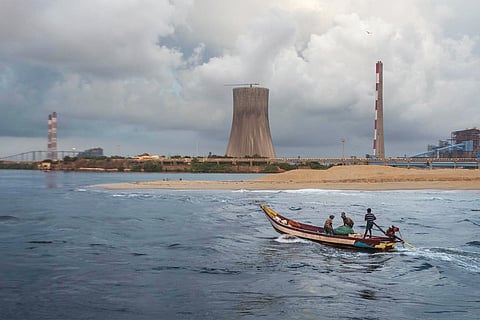
On April 12, the Tamil Nadu Pollution Control Board (TNPCB) is scheduled to conduct a public hearing for state-owned electricity utility TANGEDCO’s proposal to set up the Ennore Thermal Power Station (ETPS), a 660 MW coal-fired thermal power plant in Ennore in north Chennai. Coming up in a state where the government is talking up its commitment to combating climate change and social injustice, its insistence on pushing ETPS in an already over-polluted region exposes the yawning gap that the government needs to span to actually walk its talk on both counts. Here’s why.
Environmental casteism
Even after the racial epithet “Black Town” in reference to north Chennai was dropped post-independence, this predominantly working class region remains a site of structural environmental and social discrimination. The proposed power plant falls within the Ennore-Manali industrial area, a patchwork of localised toxic hotspots within north Chennai’s polluted airshed. Ennore’s cluster of thermal power plants has 3,300 MW of coal-burning capacity; of this, TANGEDCO operates 1,800 MW and NTPC-owned NTECL Vallur operates 1,500 MW.
Two more TANGEDCO projects totalling 2,120 MW are at an advanced state of completion, and two projects of 1,320 MW capacity are in the pre-licensing stage. TANGEDCO’s ETPS (expansion) is one of the latter two; ETPS (replacement) is the other. If all plans materialise despite local opposition, the Ennore cluster will be home to 7,740 MW of coal-burning power plants. Then, Chennai will have the dubious distinction of hosting the largest capacity of carbon-spewing coal power plants among any metropolitan region in the world.
That is not all. North Chennai is home to a 10 million tonne/year oil refinery, 36 large, red category petrochemical and other factories, and the city’s largest garbage dump. Taken together, the Ennore-Manali region contains the densest concentration of fossil-fuel industries in south India.
Demographically, north Chennai is also home to the densest concentration of marginalised communities in the Metropolitan area. According to the Environmental Impact Assessment (EIA) report for the ETPS (expansion) project, more than 37% of the population in the study area is from Scheduled Caste, Scheduled Tribe or Most Backward Communities. It is no coincidence that such a high concentration of dirty industries is located in this region. This is modern untouchability, or as social scientist MSS Pandian puts it “caste by other means”.
Officially over-polluted
Industries in north Chennai operate in a regulatory vacuum. In November 2020, Chennai Climate Action Group, a youth-led initiative, published a report titled ‘Poison in the Air’ that analysed real-time emission data from the smokestacks of six large polluting industries, including TANGEDCO’s North Chennai Thermal Power Station (NCTPS), NTECL, CPCL’s oil refinery, Madras Fertilisers Ltd, Tamil Nadu Petroproducts Ltd and Madras Petrochemical Ltd. Taken together, the six were operating in violation of prescribed air pollution norms for nearly 60% of the year in 2019. The fact that the data was available with and sourced from the TNPCB using Right to Information exposes the agency’s ineptitude as a regulator.
The report triggered suo motu action by the National Green Tribunal, which constituted a Joint Committee comprising senior officials of the Union environment ministry, Central Pollution Control Board (CPCB) and TNPCB, and a scientist from Anna University. Buried in the report are two key findings:
1. Ground-level Particulate Matter (PM) pollution due to emissions from NCTPS’ Stage I power plant alone exceeded the carrying capacity of the area in the vicinity of the plant.
2. Ground-level sulphur dioxide (SO2) and nitrogen dioxide (NO2) pollution from emissions from CPCL’s oil refinery exceeded the carrying capacity of the area in the vicinity of the plant.
The carrying capacity of an airshed for an air pollutant, say PM 10 or SO2, is the maximum emission (or the natural limit) of that air pollutant that can be released from all sources into the airshed such that these emissions do not lead to concentrations of that pollutant in excess of local ambient air quality standards.
The Joint Committee’s report finds that the carrying capacity for PM 10 has been exhausted just from the emissions from NCTPS’s Stage I, and for SO2/NO2 by emissions from CPCL. The cumulative impact of pollution from other industries or the movement of heavy vehicles to and from the port and factories was not even considered.
TNPCB claims to have issued notices demanding “environmental compensation” of more than Rs 8 crore from the six industries. But none has paid up. Even if they do, the compensation will not reach the victims of the pollution. Rather it will be paid to the account of TNPCB that allowed the pollution in the first place.
SO2 and dust or PM pollution are serious health hazards. SO2 is an acidic gas that irritates the lining of the nose, throat and lungs, and can worsen existing respiratory illnesses, and heart disease. PM pollution is linked to various kinds of cancers, reduced lung function, cardiovascular and respiratory disorders, and an overall reduction in life expectancy.
Curiously, TANGEDCO’s EIA for the proposed ETPS plant also confirms the over-polluted state of the local airshed. According to the EIA, PM 10 levels recorded in all seven residential areas – Ennore, Athipattu, Sivagami Nagar, Vallur, Ponniamman Nagar, Ernavur village and Manali New Town, and 2 industrial sites, including within the proposed ETPS site – exceeded safe levels.
Official confirmation
Earlier this month, a report of a Joint Experts Committee (JEC) set up by the National Green Tribunal (SZ) in a case (OA 8 of 2016) filed by an Ennore fisherman appeared on the tribunal’s website. This committee looked into the pollution – particularly coal ash leaks – caused by TANGEDCO’s NCTPS. The high-level committee included Santha Sheela Nair, IAS (retd) as chairperson, senior officials from the CPCB and TNPCB, Dr Balaji Narasimhan, hydrologist, IIT-Madras, Dr Indumathi Nambi, environmental scientist, IIT-Madras, eminent botanist Dr D Narasimhan, restoration ecologist Dr Jayashree Vencatesan, and Dr Deepak Samuel, a marine biologist from the National Centre for Sustainable Coastal Management.
The report’s findings reveal the depth of the pollution and health crisis already underway in north Chennai. Unlike the detached and often substandard reports submitted by committees dominated by regulatory authorities, the JEC’s report relies on and narrates the experiences of members during field visits and interactions with local residents, and draws from detailed investigations by scientific members of the committee.
A sample of extracts from the JEC’s observations from the field visit, public consultation and findings are reproduced below:
From the Site Visit: “The JEC visited the ash-contaminated sites by road on 30.12.2021. A site visit report is annexed. [Annexure 3] However, we also wish to register our observations not merely as unemotional observers, but also at a human level.
“The visit brought home to all committee members the harsh reality of the life of local residents. The committee’s exposure to ash-choked neighbourhood, though brief, was distressful. Visibility was poor due to the dust in the air. Many committee members experienced breathing difficulty after some time there and eye irritation. The sheer physical discomfort in the brief period the committee spent there deeply impacted us as it made us realise that this is a 24/7 phenomenon for people living here.”
From the Public Consultation:
“The committee’s own observations that polluters have polluted and continue to pollute with impunity were repeated by members of the public who were openly cynical of this committee and voiced their fears of this also being just another “eye-wash.”
“Pollution is reported to be the main cause of illness in the region. NCTPS was identified as a major cause of air pollution, and the region is a hotspot of pollution due to the concentration of power plants and other polluting industries. Children and women are particularly affected, and gynaecological problems were particularly reported by respondents. . .They expressed concern that more and more polluting industries are proposed to be located in Ennore unmindful of the prevailing health crisis.”
From the Findings sections (paraphrased):
Unlicensed operation
- TANGEDCO’s NCTPS Stage I and II have operated without a valid Consent/License under Air and Water Acts since 2015 and 2019 respectively.
Illegal emissions
- CPCB/TNPCB found TANGEDCO had tampered with the Online Continuous Emission Monitoring System so that actual results are not transferred to CPCB and TNPCB.
- Despite the tampering, between 01.04.2019 and 07.03.2022 (1071 days), NCTPS Stage 1 emitted particulate matter (including ash) in excess of permissible levels for 481 days.
- During the same period (1091 days), NCTPS Stage II emitted particulate matter (including ash) in excess of permissible levels for 791 days.
Cancer risk
- Adults face a high cancer and non-cancer risk due to cadmium and lead exposure.
- Cancer and non-cancer risk for children is much higher due to exposure to cadmium, lead and copper. Compared to an acceptable cancer risk norm of 1 in a million, cancer risk for children due to exposure to these pollutants is a 1000 times higher.
Wetlands choked, contaminated by coal ash
- More than 56 lakh tonnes of coal ash is spread over the riverbed, with flyash deposits ranging in depth from 1 ft to 8 ft.
- Natural drainage patterns have been considerably altered due to fly ash pond construction and ash contamination. This will have an impact on local hydrology and flooding.
- Surface sediments are heavily contaminated with levels of cadmium, chromium, copper, lead, nickel and zinc well in excess of safe levels as per Canadian sediment quality guideline for protection of aquatic life.
Loss of wetlands
- The Ennore wetlands studied by JEC has shrunk from 855.69 ha in 1996 to 277.92 ha in 2022. Meanwhile,
◦ built-up area increased from 0 ha to 259.87 ha;
◦ area covered by flyash increased from 0 ha to 260.28 ha;
◦ area under mangroves decreased 68.72 to 33.74 ha.
Impact on fisher livelihoods
- Several fish species have either disappeared or diminished. These include: White prawn, black prawn, sand prawn, tiger prawn, green crab (Scylla serrata), Flotosus canius, Mugil cephalus, Silver Biddy (Gerres sp.), sand whiting (Sillago sihama), Terrapon jarbua, sea bass (Lates calcarifer), and other fishes locally called Kalavan, Udupathi, Panna, Oodan.
- Damage to the river and reduction in fish catch has drastically affected fisher livelihoods, including of women fishers from SC/ST community who hand-pick shrimp and crab from the river bed.
- The impacts of pollution on livelihoods and health has eroded the economic status of affected people due to lost income, lost work days and increased health care expenditure.
A climate reckless act
On April 4, the United Nations Intergovernmental Panel on Climate Change’s (IPCC) third Working Group released a report that unequivocally declared that no new coal plants can be built anywhere in the world if we are to have any hope of keeping global temperature rise below 1.5 degree Celsius. This is the threshold beyond which the costs of adapting to climate change will become prohibitively expensive and yield diminishing returns. The window of opportunity to keep climate disasters within manageable limits is closing fast, and will have closed completely by 2030 – just 8 years away.
IPCC’s reports – this one on mitigation and the earlier one on adaptation – spotlight the increased risk exposure of marginalised communities and the poor. Both between nations and within countries, it is the poorer nations and people – those that contributed the least to the climate crisis – who will be worst affected by the effects of climate change. The report’s prescriptions for Climate Resilient Development situate equity and social justice concerns at the centre of all interventions, and warn that measures taken either in the name of development or climate adaptation that aggravate inequity and injustice will defeat global efforts to contain runaway climate change and meet the UN Sustainable Development Goals.
Climate change manifests itself in the form of rising sea levels, increased heat stress, intense rain events and droughts, salinity intrusion and ocean desertification due to warming seas. Coastal wetlands like the Ennore estuary and Kosasthalai’s backwaters are natural shock absorbers that mitigate flooding during heavy rains or tidal surges, check salinity intrusion and keep the oceans healthy by restocking it with fish. ETPS will aggravate the already degraded state of the Ennore wetlands and nearshore seas.
- The ETPS project envisages a coal ash dump inside the river, and a coal conveyor corridor cutting through the already degraded estuary and mangrove-fringed islands.
- Each day, more than 170 million (17 crore) litres of seawater will be drawn using giant pumps that will suck in marine life along with water. What life does not get killed by getting trapped by the filters fitted at the pipe-heads will be killed by dousing with biocides in the intake water storage tanks.
- More than 120 million litres of hot wastewater will be discharged daily into the ocean. Marine organisms are hypersensitive to temperature changes. Such discharge in an already warming ocean will create localised marine deserts in the nearshore waters, and further hurt the artisanal fisher economy.
For all the controversy it has generated, ETPS does not even address an electricity shortage. In 2019-20, all state-run thermal power plants operated at a plant load factor of 56%, according to the government’s submission to the state Assembly. That means the existing plants remained idle for the equivalent of 160 out of 365 days*.
The ETPS project is a reckless proposal because of its global climate implications and because of what it does to local communities. Just in Ennore and between the two power plants under construction and the two that are in pre-licensing stage, the state government is investing Rs 30,000 crore in climate-changing, inequity-aggravating activities. Meanwhile, for all of Tamil Nadu, the government has announced a Rs 500 crore climate mission and a Rs 100 crore wetland mission. For Tamil Nadu to stay true to its declared commitment to social justice and combating climate change, this imbalance has to be rapidly set right. Abandoning the ETPS proposal and investing in improving local health, health care and the health of the wetlands would be a step in that direction.
* Source: Energy Policy Note, Government of Tamil Nadu, 2020-2021.
Nityanand is a Chennai-based writer and social activist. Views expressed are the author’s own.
Related Stories
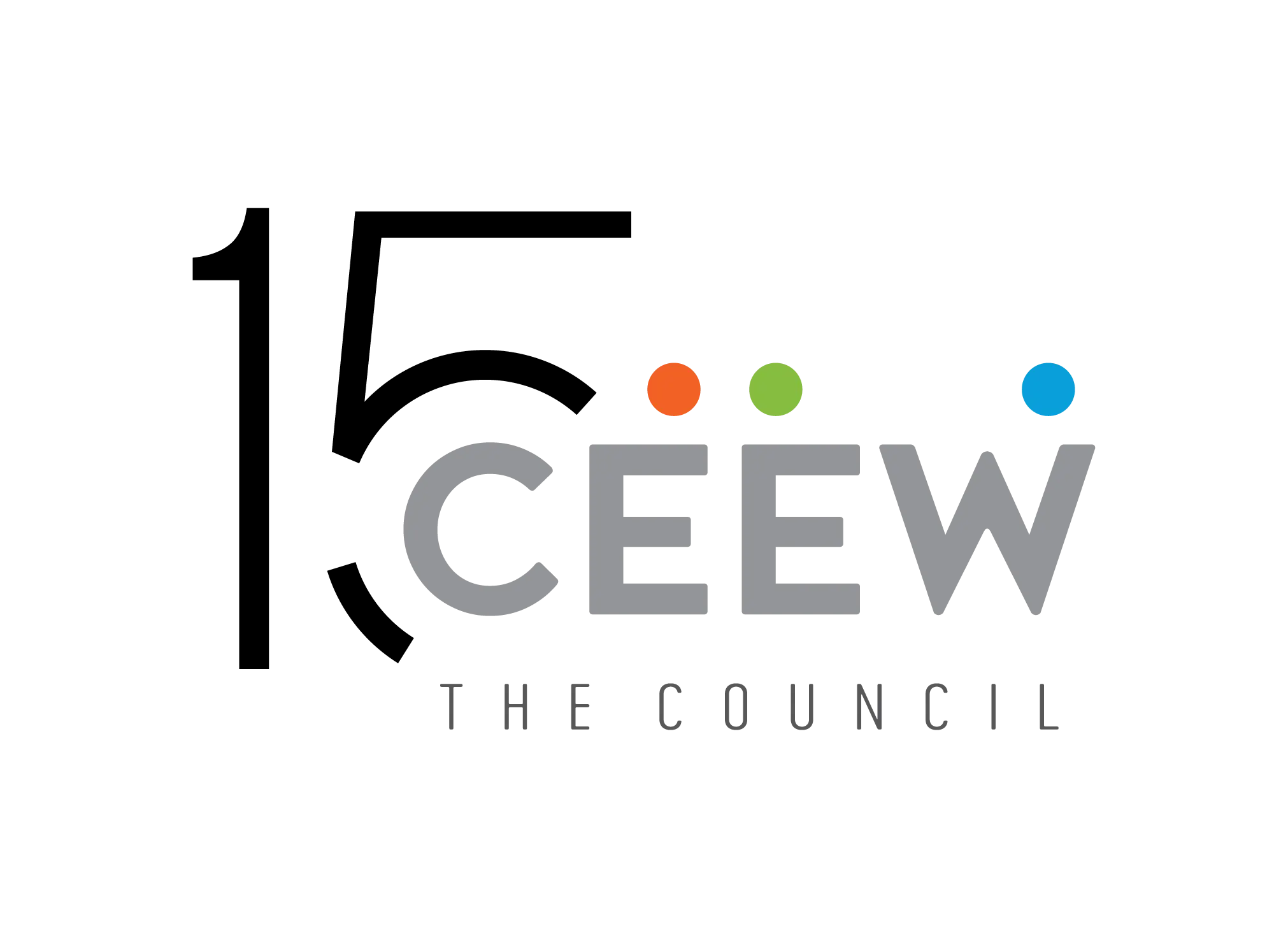
Suggested citation: Khan, Adeel, Uday Suryanarayanan, Tanushree Ganguly, and Karthik Ganesan. Improving Air Quality Management through Forecasts: A Case Study of Delhi’s Air Pollution of Winter 2021. New Delhi: Council on Energy, Environment and Water.
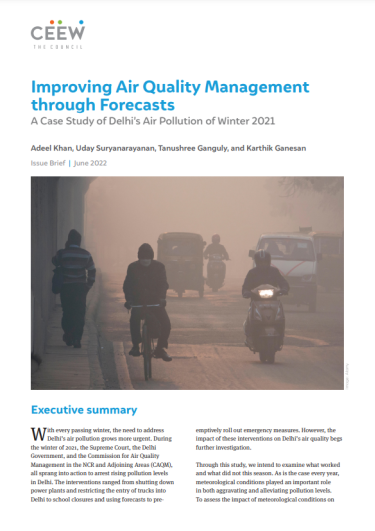
This study assesses Delhi’s air pollution scenario in the winter of 2021 and the actions to tackle it. Winter 2021 was unlike previous winters as the control measures mandated by the Commission of Air Quality Management (CAQM) in Delhi National Capital Region and adjoining areas were rolled out. These measures included the Graded Response Action Plan (GRAP) and additional emergency responses instituted on the basis of air quality and meteorological forecasts. Given that the forecasts play a major role in emergency response measures, the study assesses the reliability of different forecasts. Further, it gauges the impact of the emergency measures on Delhi’s air quality levels. It also discusses the primary driver of air pollution in winter 2021.
Key Findings
- While air quality forecasts picked up the pollution trends, they are not yet very accurate in predicting high pollution episodes ('very poor' and 'severe' air quality days)
- When the restrictions were in place like ban on entry of trucks, construction & demolition activities and others, air quality did not descend into the ‘severe +’ category. Moreover, air quality improved from ‘severe’ to ‘poor’ when all the restrictions were in place simultaneously, aided by better meteorology.
- However, when the restrictions were finally lifted, the air quality spiralled back into the ‘severe’ category resulting in the longest six days ‘severe’ air quality spell of the season.
- There has been no significant improvement in Delhi's winter air quality since 2019. In winter 2021, air quality was in the ‘very poor’ to ‘severe’ category on about 75 per cent of days.
- In the winter of 2021, transport(∼ 12 per cent), dust (∼ 7 per cent) and domestic biomass burning (∼ 6 per cent) were the largest local contributors.
- About 64 per cent of Delhi’s winter pollution load comes from outside of Delhi’s boundaries.
HAVE A QUERY?
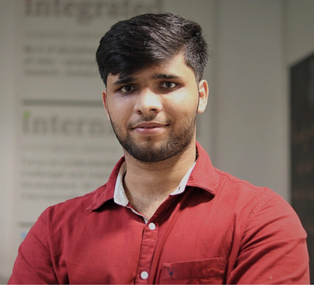
Executive Summary
With every passing winter, the need to address Delhi’s air pollution grows more urgent. During the winter of 2021, the Supreme Court, the Delhi Government, and the Commission for Air Quality Management in the NCR and Adjoining Areas (CAQM), all sprang into action to arrest rising pollution levels in Delhi. The interventions ranged from shutting down power plants and restricting the entry of trucks into Delhi to school closures and using forecasts to pre-emptively roll out emergency measures. However, the impact of these interventions on Delhi’s air quality begs further investigation.
Through this study, we intend to examine what worked and what did not this season. As is the case every year, meteorological conditions played an important role in both aggravating and alleviating pollution levels. To assess the impact of meteorological conditions on pollution levels, we analysed pollution levels during the months of October to January vis-a-vis meteorological parameters. To understand the driving causes of pollution in the winter of 2021, we tracked the changes in relative contribution of various polluting sources as the season progressed.
While pre-emptive actions based on forecasts was a step in the right direction, an assessment of forecast performance is a prerequisite to integrating them in decision-making. We also assessed the performance of forecasts by comparing them with the measured onground concentrations. We also studied the timing and effectiveness of emergency directions issued in response to forecasts.
We sourced data on pollution levels from Central Pollution Control Board’s (CPCB) real-time air quality data portal and meteorological information from ECMWF Reanalysis v5 (ERA5). For information on modelled concentration and source contributions, we used data from publicly available air quality forecasts, including Delhi’s Air Quality Early Warning System (AQ-EWS) (3-day and 10-day), the Decision Support System for Air Quality Management in Delhi (DSS), and UrbanEmissions.Info (UE).
Figure ES1 In Delhi, 75% of Winter 2021 saw 'very poor' to 'severe' air quality
Source: Authors’ analysis, data from Central Pollution Control Board (CPCB). Note: Air quality index (AQI) for the day is calculated using the PM2.5 concentration at the same stations with a minimum of 75 per cent of the data being available.
A. 75 per cent of days were in ‘Very poor’ to ‘Severe’ air quality during winter 2021
The number of ‘Severe’ plus ‘Very poor’ air quality days during the winter has not decreased in the last three years (Figure ES1). During the winter of 2021 (15 October 2021 - 15 January 2022), about 75 per cent of the days, air quality were in the ‘Very poor’ to ‘Severe’ category. Interestingly, despite more farm fire incidents in Punjab, Haryana, and Uttar Pradesh in 2021 compared to 2020, Delhi’s PM2.5 concentration during the stubble burning phase (i.e., 15 October to 15 November) was lesser in 2021. This was primarily due to better meteorological conditions like higher wind speed and more number of rainy days during this period.
B. Regional influence predominant; Transport, dust, and domestic biomass burning are the largest local contributors to air pollution
We find that about 64 per cent of Delhi’s winter pollution load comes from outside Delhi’s boundaries (Figure ES2(a). Biomass burning of agricultural waste during the stubble burning phase and burning for heating and cooking needs during peak winter are estimated to be the major sources of air pollution from outside the city according to UE (Figure ES2(b). Locally, transport (12 per cent), dust (7 per cent), and domestic biomass burning (6 per cent) contribute the most to the PM2.5 pollution load of the city. While transport and dust are perennial sources of pollution in the city, the residential space heating component is a seasonal source. However, this seasonal contribution is so significant that as the use of biomass as a heat source in and around Delhi starts going up as winter progresses, the residential sector becomes the single-largest contributor by 15 December (Figure ES2(b)). This indicates the need to ramp up programmes to encourage households to shift to cleaner fuels for cooking and space heating.
Figure ES 2(a) Transport, dust, and domestic biomass burning are the largest local contributors to the PM2.5 pollution load in Delhi
Source: Authors’ analysis, source contribution data from DSS and UE. Note: Modelled estimates of relative source contributions retrieved from UE and DSS.
Figure ES 2(b) Both local and regional sources need to be targeted for reducing Delhi’s pollution
Source: Authors’ analysis, source contribution data from UE. Note: Source contribution data retrieved from UE district products which have larger geographical cover and lower resolution.
C. Forecasts picked up the pollution trend but could not predict high pollution episodes
The availability of multiple forecasts provides decisionmakers with a range of options to choose from. At the same time, this is an obstacle to effective onground action. To streamline the flow of relevant information from forecasters to decision-makers, it is important to analyse the forecasts and assess their reliability. We found that all the forecasts identified pollution trends accurately (Figure ES3) but their accuracy in predicting pollution episodes (‘Severe’ and ‘Very Poor’ air quality days) decreases with future time horizon.
D. Though forecasts were used to impose restrictions, the lifting of the curbs was ill-timed
In November–December 2021, apart from the Graded Response Action Plan (GRAP) coming into effect in DelhiNCR, the CAQM introduced several emergency response measures through a series of directions and orders. The Supreme Court also stepped in from time to time to direct the authorities to act on air pollution.
As a first, the CAQM used air quality and meteorological forecasts to time and tailor emergency response actions. The first set of restrictions was put in place on 16 November 2021, and all were lifted by 20 December 2021, save the one on industrial operations.
Figure ES3 All the forecasts can predict the trend accurately
Source: Authors’ analysis, data from Central Pollution Control Board (CPCB), AQ-EWS, and UE. Note: r represents correlation.
During this period, all the forecasts except AQ-EWS (3-day) underpredicted PM2.5 levels. Therefore, by looking at the difference between forecasted and measured concentrations, it is not possible to gauge the effectiveness of the restrictions conclusively. Hence, multiple models or different modelling experiments are needed to estimate the impact of the intervention.
It should be noted that during the restriction period, air quality did not descend into the ‘Severe +’ category. Further, when all the restrictions were in place along with better meteorology, air quality did improve from ‘Severe’ to ‘Poor’. The first prolonged ‘Severe’ air quality period in December was witnessed between 21 December and 26 December. While the forecasts sounded an alarm for high pollution levels during this period, all restrictions barring those on industrial activities were lifted. Subsequently, PM2.5 levels remained above 250 µgm -3 for six straight days resulting in the longest ‘Severe’ air quality spell of the season. (Figure ES4).
Figure ES4 The lifting of the restrictions was ill-timed with high pollution levels forecasted in the following days
Source: Authors’ analysis, data from Central Pollution Control Board (CPCB). Note: C&D stands for construction and demolition activities. Work from home (WFH) stands for the 50% cap on employee attendance in the office. Industrial restrictions stand for compulsory switching over to Piped Natural Gas (PNG) or other cleaner fuels within industries and non-compliant industries being allowed to operate restrictively.
The discussion above highlights that despite the emergency measures taken in winter 2021, the air quality conditions were far from satisfactory. Calibrating emergency responses to forecasted source contributions may result in a greater impact on air quality. Our study recommends the following to help the Government of National Capital Territory of Delhi ( GNCTD) and CAQM plan and execute emergency responses better:
- GRAP implementation must be based strictly on modelled source contributions obtained from forecasts and timed accordingly. This will eliminate the need for ad-hoc emergency directions to restrict various activities. For instance, restrictions on private vehicles can be brought in when the air quality is forecasted to be ‘Very poor’ as transport is a significant contributor.
- Surveys or assessments are required in the residential areas across NCR to explore the prevalence of biomass usage for heating and cooking purposes. Based on this, a targeted support mechanism is required to allow households and others to use clean fuels for cooking and heating. There is also a need to assess and promote alternatives for space heating.
- Air quality forecasts should be relayed to the public via social media platforms to encourage them to take preventive measures such as avoiding unnecessary travel and wearing masks when stepping out. This will help reduce individual exposure and activity levels in the city.
- Ground level data and insights need to be incorporated in forecasting models. Data from sources like social media posts (text and photos), camera feeds from public places, and pollution related grievance portals like SAMEER, Green Delhi, and SDMC 311 can provide near-real time information on pollution sources. Then aggregated representation of polluting activities based on recent days or weeks can be used as an input in models. Ultimately, a crowd-sourced emissions inventory for NCT/NCR will benefit modellers and policymakers alike while also making pollution curtailment efforts transparent.
- Combining the available air quality forecasts through an ensemble approach can help improve the accuracy of the forecasts and prompt better coordination within the modelling community.
Sign up for the latest on our pioneering research
Explore related publications.
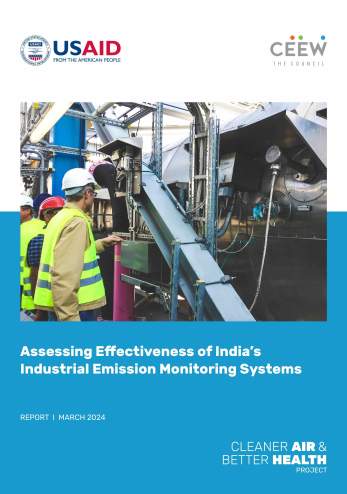
Assessing Effectiveness of India’s Industrial Emission Monitoring Systems
Hemant Mallya, Sankalp Kumar, Sabarish Elango
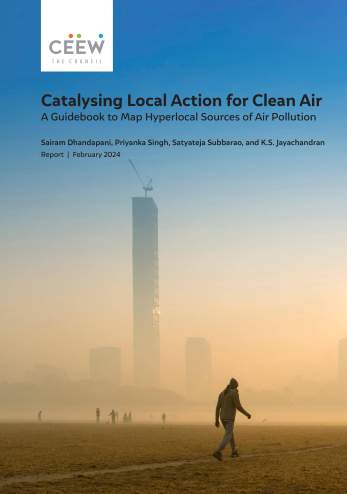
Catalysing Local Action for Clean Air
Sairam D, Priyanka Singh, Satyateja Subbarao, KS Jayachandran
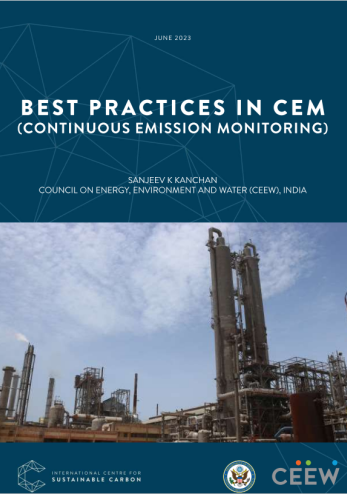
Best Practices in CEM (Continuous Emission Monitoring)
Sanjeev K Kanchan
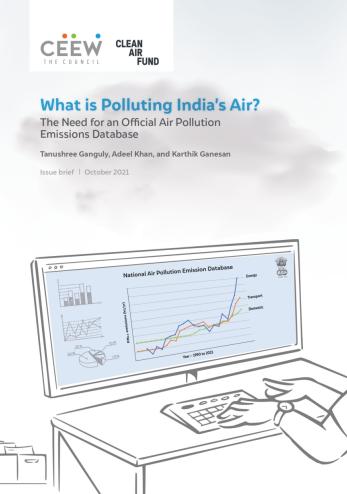
What is Polluting India’s Air? The Need for an Official Air Pollution Emissions Database
Tanushree Ganguly, Adeel Khan and Karthik Ganesan
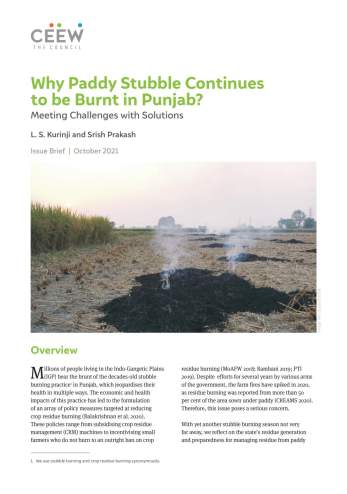
Why Paddy Stubble Continues to be Burnt in Punjab?
L. S. Kurinji, Srish Prakash
- Research and Innovation
- The Abstract
- Audio Abstract Podcast
- Centennial Campus
- Campus Life
- Faculty and Staff
- Awards and Honors
- HR and Finance
- Resilient Pack
- We Are the Wolfpack
- Service and Community
- Red Chair Chats
- News Releases
- In the News
- NC State Experts on 2022 Elections
- NC State Experts Available on Climate
- NC State Experts on Roe v. Wade
- NC State Supply Chain Experts
- Experts on COVID-19
- Hurricane Experts
- About NC State News
- Faculty Support
- Training Program
Climate Change Will Make Air Pollution Worse. Here’s How.
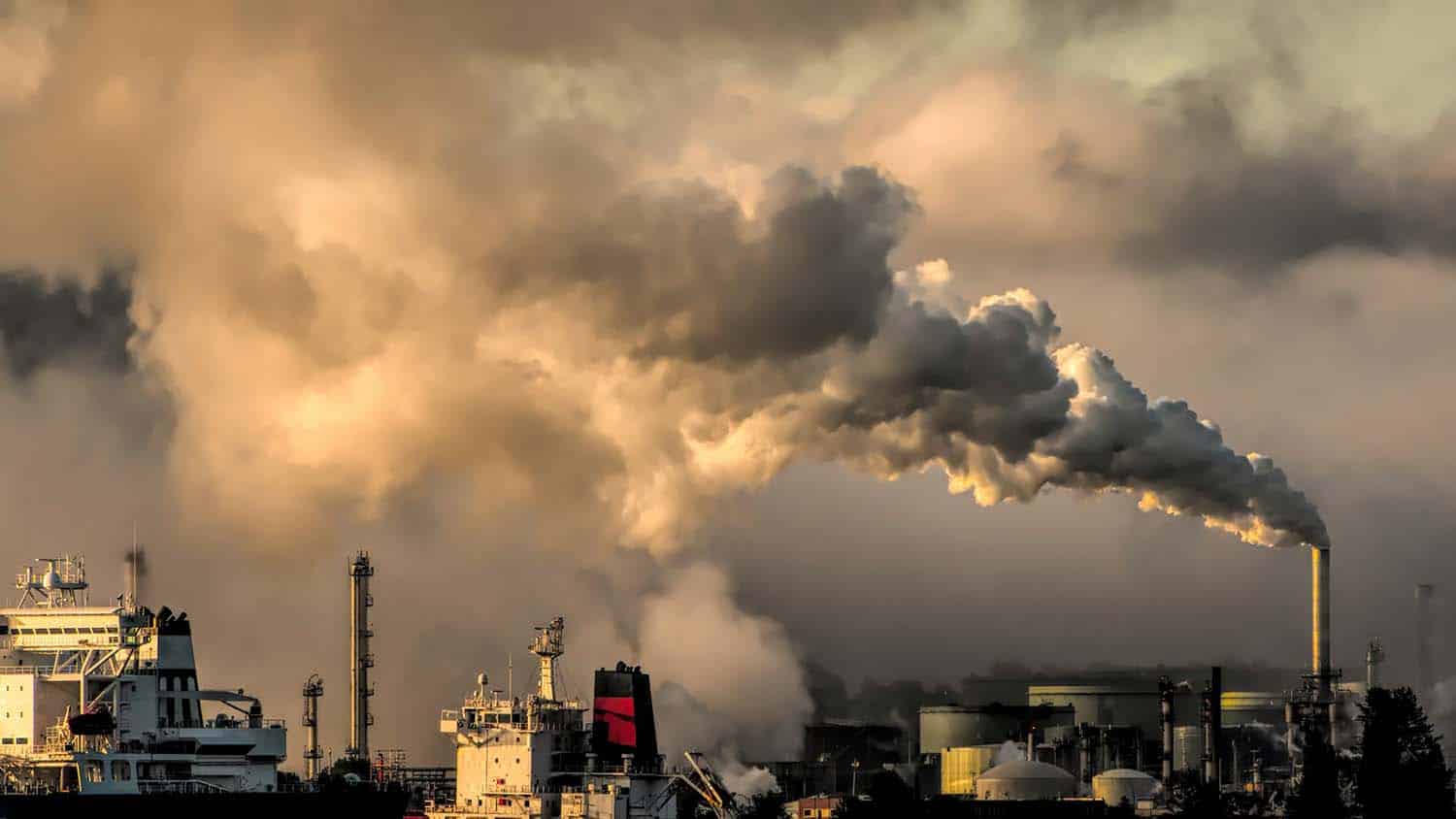
For Immediate Release
A new study finds climate change is likely to make upward spikes of ozone at ground level worse by 2050, which could result in many parts of the United States falling out of compliance with air quality standards and increasing risks to public health.
Ozone is a reactive gas that consists of three oxygen atoms. And while it can be naturally occurring, at ground level it is often formed by the interaction of volatile organic compounds (VOCs) and nitrogen oxides (NOx) – which are both air pollutants.
“Climate change affects ozone formation through a complex set of factors, but warmer temperatures are correlated with increases to ozone in polluted areas,” says James East, first author of a paper on the study and a former Ph.D. student at North Carolina State University who is now a researcher at Harvard University.
“That means areas that already have higher levels of air pollution of VOCs and NOx will likely see increases in ozone as average temperatures go up. What’s more, climate change is expected to increase naturally occurring VOC emissions in some parts of the U.S., such as the Southeast, exacerbating the challenge.”
Increases in ozone are important because ground-level ozone can cause a wide array of health problems , including decreased lung function and inflammation of airways, contributing to hundreds of thousands of deaths each year .
“It’s pretty well-established that climate change will increase ozone pollution , but there has been a tremendous amount of uncertainty regarding what that increase might look like,” says Fernando Garcia Menendez, corresponding author of the work and an associate professor of environmental engineering at NC State. “Our goal with this work was to quantify the range of outcomes and get a much clearer picture of how climate change will affect ozone pollution events in the U.S.”
“Atmospheric chemistry is complex, and climate change affects the rate of chemical reactions, the amount of ozone precursors present, and how long the ozone will linger in the environment,” Garcia Menendez says. “We built on a variety of existing models and incorporated statistical tools that allow us to account for this wide array of variables to look at ozone pollution in the years ahead.”
Specifically, the researchers looked at how often ozone levels would exceed air quality standards designed to protect public health, how far ozone levels would overshoot the standards, and how that may change by 2050.
The study’s findings are presented as a range, because climate scientists still have some uncertainty about how sensitive the climate is to changes in the concentration of greenhouse gases.
The best case scenario is that the climate has a relatively low sensitivity to carbon dioxide. If that is the case, the new study finds that the high end of ozone measurements would – on average – go up by less than 0.3 parts per billion (ppb). Those high measurements could still be below the existing air quality standards for many locations.
“However, even in this best case scenario, we found that more variability in ozone levels is projected for 2050 – meaning that we’d still expect to see an increase in the number of days where there is an exceptionally high increase in ozone, violating the air quality standard,” says East.
The worst case scenario is that the climate is highly sensitive to carbon dioxide. In that case the study says the high end of ozone measurements would – on average – go up by more than 2.3 ppb. Coupled with the increase in variability, this would mean that many parts of the country would see a significant increase in the number of days when ozone levels exceed air quality standards.
“In practical terms, our study finds that between 5 million and 13 million additional people will be exposed to dangerously high levels of ozone in 2050,” East says.
“Right now, state and federal governments are trying to manage ozone levels by reducing emissions of air pollutants,” says Garcia Menendez. “This work suggests that the current emissions reductions efforts may be less effective for helping meet ozone standards for many parts of the country, particularly those that are already struggling to meet air quality standards.”
“This work is important for two reasons,” East says. “First, it contributes to our understanding of how climate change will affect ground-level air quality and, by extension, human health. Among other things, this contributes to the way we estimate cost/benefit analyses of climate regulations and related technologies.
“Second, by clarifying the range of climate impacts on ozone, we’re providing critical information that can inform policy decisions – such as EPA’s ongoing review of the air quality standard for ozone .”
The paper, “ Projecting Changes in the Frequency and Magnitude of Ozone Pollution Events Under Uncertain Climate Sensitivity ,” is published in the open access journal Earth’s Future . The paper was co-authored by Erwan Monier of the University of California Davis; and by Rebecca Saari of the University of Waterloo.
The work was done with support from the Natural Sciences and Engineering Research Council of Canada, under grant number RGPIN-2023-03807.
Note to Editors: The study abstract follows.
“Projecting Changes in the Frequency and Magnitude of Ozone Pollution Events Under Uncertain Climate Sensitivity”
Authors : James D. East, North Carolina State University and Harvard University; Erwan Monier, University of California Davis; Rebecca K. Saari, University of Waterloo; and Fernando Garcia-Menendez, North Carolina State University
Published : June 2, Earth’s Future
DOI : 10.1029/2023EF003941
Abstract: Climate change is projected to worsen ozone pollution over many populated regions, with larger impacts at higher concentrations. More intense and frequent ozone episodes risk setbacks to human health and environmental policy achievements. However, assessing these changes is complicated by uncertain climate sensitivity, closely related to climate model response, and internal variability in simulations projecting climate’s influence on air quality. Here, leveraging a global modeling framework that one-way couples a human activity model, an Earth system model of intermediate complexity, and an atmospheric chemistry model, we investigate the role of climate sensitivity in climate-induced changes to high ozone pollution episodes in the United States using multiple greenhouse gas emissions scenarios, representations of climate sensitivity, and initial condition members. We bias correct and evaluate historical model simulations, identifying modeled and observed O 3 episodes using extreme value theory, and extend the approach to projections of mid- and end-century climate impacts. Results show that the influence of climate sensitivity can be as significant as that of greenhouse gas emissions scenario absent precursor emissions changes. Climate change is projected to increase the magnitude of the highest annually occurring O 3 concentrations by over 2.3 ppb on average across the U.S. at mid-century under a high climate sensitivity and moderate emissions scenario, but the increase is limited to less than 0.3 ppb under lower climate sensitivity. Further, we show that areas in the U.S. currently meeting air quality standards risk being pushed into non-compliance due to a climate-induced increase in frequency of high ozone days.
- Designing Healthy and Resilient Societies
- Driving Food, Water and Energy Solutions
- Harnessing Data for Decision-Making
- climate change
- college of engineering
- Department of Civil Construction and Environmental Engineering
- environmental engineering
- health and well-being
- research news
More From NC State News
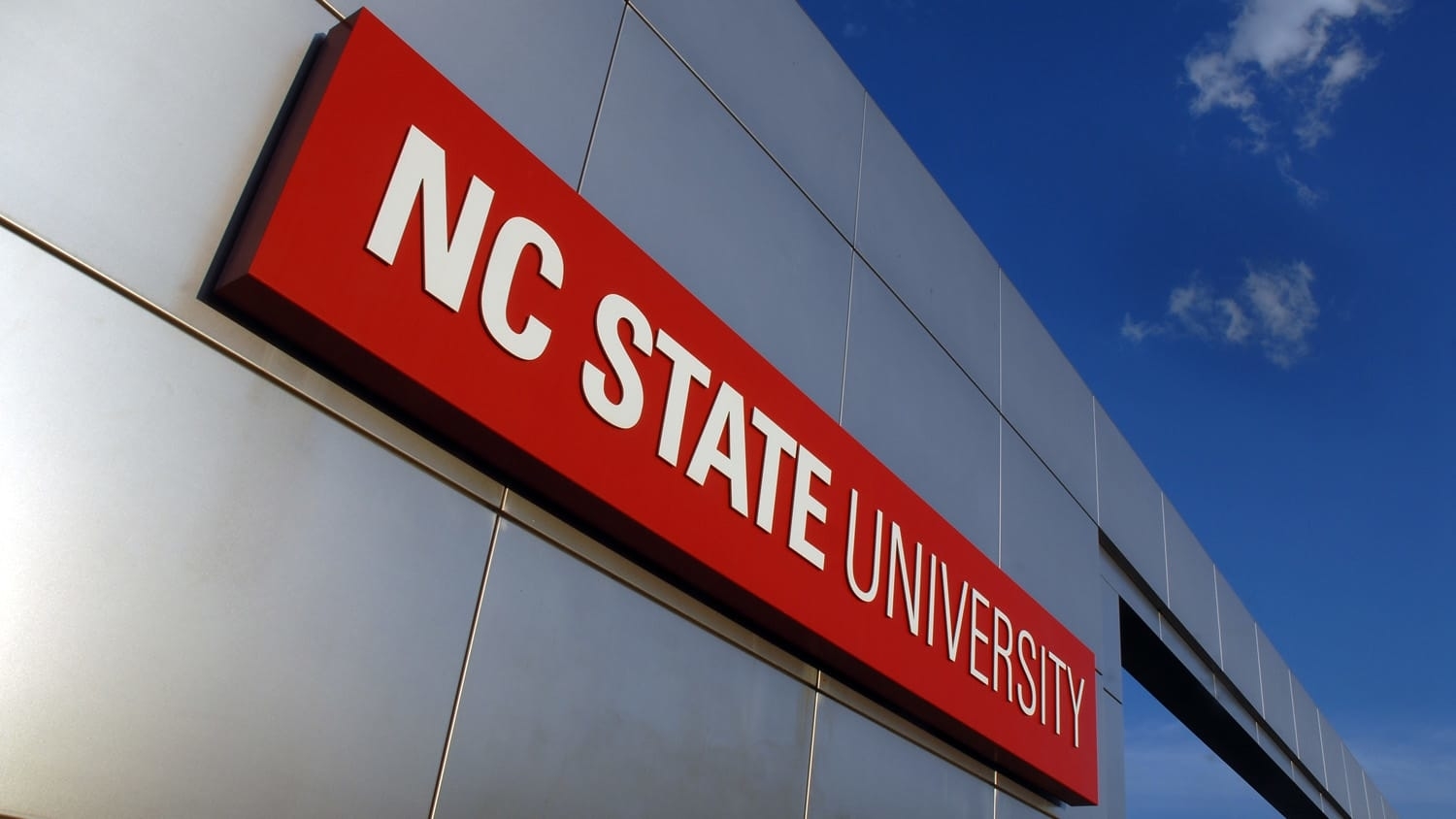
Bartonella DNA Found in Blood of Patients With Psychosis
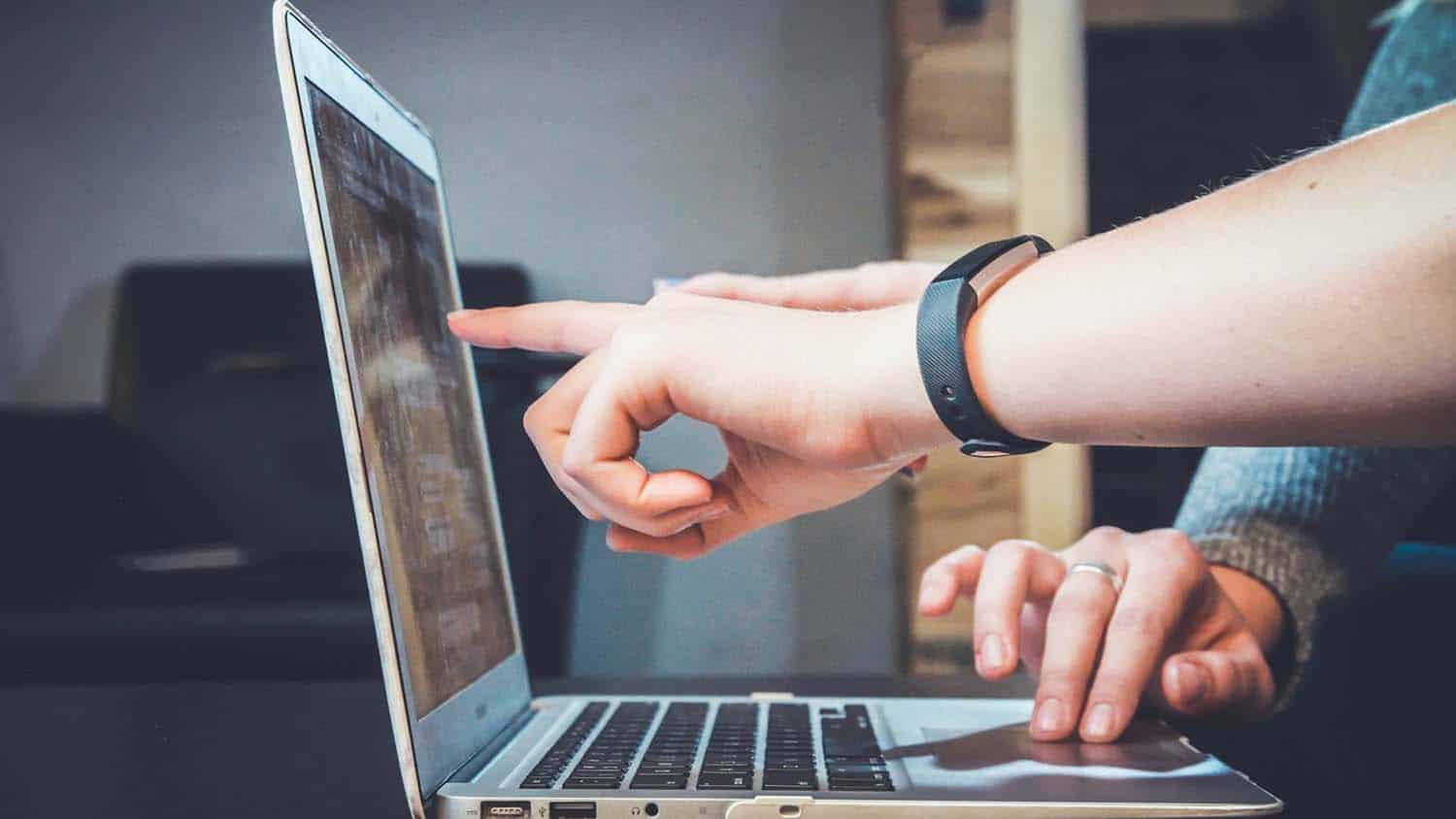
How to Build a Team of ‘Appropriately Skeptical’ Financial Statement Auditors
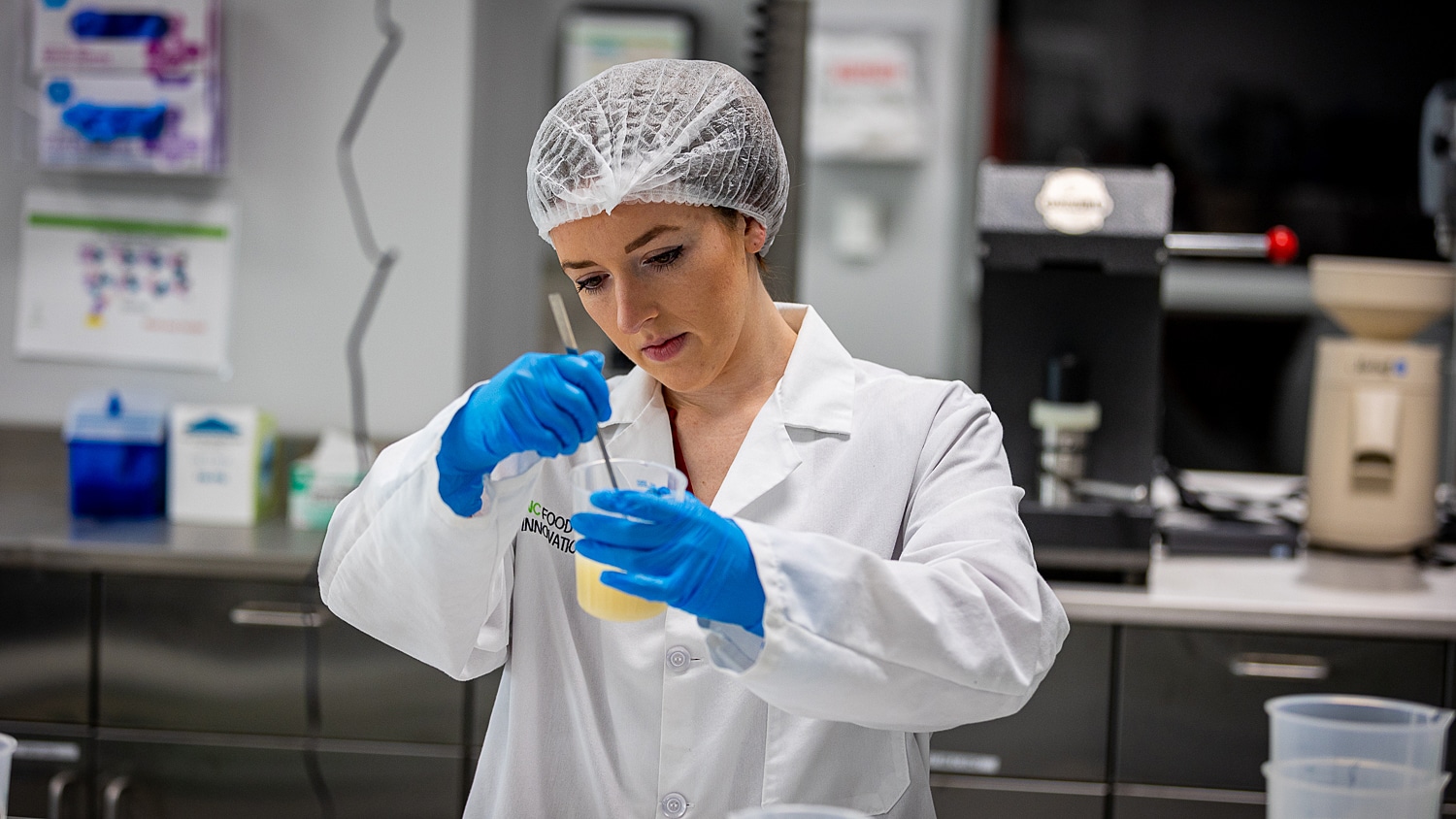
Bezos Earth Fund Grant Creates Sustainable Protein Research Hub at NC State
A case study of using artificial neural networks to predict heavy metal pollution in Lake Iznik
- Mert, Berna Kırıl
- Kasapoǧulları, Deniz
Artificial neural networks offer a viable route in assessing and understanding the presence and concentration of heavy metals that can cause dangerous complications in the wider context of water quality prediction for the sustainability of the ecosystem. In order to estimate the heavy metal concentrations in Iznik Lake, which is an important water source for the surrounding communities, characterization data were taken from five different water sources flowing into the lake between 2015 and 2021. These characterization results were evaluated with IBM SPSS Statistics 23 software, with the addition of the lake water quality system. For this purpose, seven distinct physicochemical parameters were measured and monitored in Karasu, Kırandere, Olukdere and Sölöz water sources flowing into the lake, to serve as input data. Concentration levels of 15 distinct heavy metals in Karsak Stream originating from the lake were as the output. Specifically, Sn for Karasu (0.999), Sb for Kırandere (1.000), Cr for Olukdere (1.000) and Pb and Se for Sölöz (0.995) indicate parameter estimation R 2 coefficients close to 1.000. Sn stands out as the common heavy metal parameter with best estimation prospects. Given the importance of the independent variable in estimating heavy metal pollution, conductivity, COD, COD and temperature stood out as the most effective parameters for Karasu, Olukdere, Kırandere and Sölöz, respectively. The ANN model emerges as a good prediction tool that can be used effectively in determining the heavy metal pollution in the lake as part of the efforts to protect the water budget of Lake Iznik and to eliminate the existing pollution.
- Water quality;
- Heavy metal parameter;
- Artificial neural networks;
- Prediction;
- Conservation
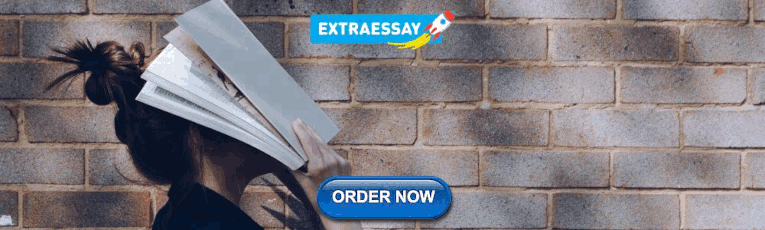
IMAGES
VIDEO
COMMENTS
Power plants with once-through (OT) cooling technologies account for 64% of water withdrawals for electricity generation in the US and cause thermal pollution, which degrades riverine ecosystems [1-7].Between 1970-2015, the US thermoelectric generation sector almost tripled its productivity in the context of water withdrawals from 110 to 39 m 3 per MWh [8-10].
Mitigation. Thermal pollution is a rapid change in temperature in a natural body of water. This pollution is most often caused by heated discharge from an industrial facility or another human ...
The presented numerical investigation is the first authors' step towards the better understanding of thermal discharge dynamics in coastal areas, by means of numerical modelling of thermal pollution. Furthermore, the study is among the few in relevant literature that focuses directly on thermal discharge dynamics rather than studying it ...
The combined study on global warming and thermal pollution may give an understanding that how aquatic organisms deal with higher temperatures. ... the temperature of Lake Victoria has been increased more than 1°C since the 1970s because of climatic change and thermal pollution problem. Another case of lake warming due to nuclear power plant is ...
Over the past thirty years the United States has developed a very strong opposition to environmental pollution. One of the first signs of this growing movement was the 1970 addition of cooling towers to the Tennessee Valley Authority's Paradise Power Plant. By focusing on thermal pollution, this paper traces the evolution of one type of environmental regulation and its impact on the Paradise ...
Respondents were told of the possible health impacts due to the pollution from the thermal power plant. They next were asked to put a monetary value under two scenarios, 11 one the thermal power plant with no pollution controls in place and two, the thermal power plant with pollution controls. Only air pollution was considered.
Singh et al. (2020) conducted a case study at National thermal power corporation (NTPC) Badarpur thermal power plant, Delhi. The concentration of Ni and Zn was reported to be high in the groundwater. ... Atmospheric emissions and pollution from the coal-fired thermal power plants in India. Atmos. Environ., 92 (2014), pp. 449-460.
In addition, we use spatial analysis to determine watersheds where more focused studies of thermal pollution may be appropriate. A case study of one of these basins, the Upper Catawba River, illustrates some of the issues with regulatory management of power plant thermal effluent. ... Langford T E 2001 Thermal discharges and pollution ...
less case studies investigated the consequences of thermal pollution on hard bottoms and, however, they were focused on single taxa (e.g., Arieli et al., 2011).
Reliable and safe operation of a coal-fired power plant is strongly linked to freshwater resources, and environmental problems related to water sources and wastewater discharge are challenges for power station operation. In this study, an evaluation on the basis of a wastewater thermal pollution vector is reported for the environmental impact of residual water generated and discharged in the ...
Technical and environmental efficiency of some coal-fired thermal power plants in India is estimated using a methodology that accounts for firm's efforts to increase the production of good output and reduce pollution with the given resources and technology. The methodology used is directional output distance function. Estimates of firm-specific shadow prices of pollutants (bad outputs), and ...
A concentration of 0.12 ppm of SO 2 for 60 days leads to fewer yields of. crops, 0.1 ppm of SO 2 for 36 days leads to leaf damage, and 0.3 ppm of SO2 for 4 hours. leads to depression of pollen ...
Alters the water properties. Thermal pollution changes the properties of water. The mixture of hazardous chemicals from various sources decreases the density and viscosity of water. It also increases the solubility of multiple gases in the water. As a result, the food supply of water creatures gets disrupted.
Liu X, Wen Z (2012) Best available techniques and pollution control: a case study on China's thermal power industry. J Clean Prod 23:113-121. Google Scholar Maheswaran R (2016) Air pollution and stroke - an overview of the evidence base. Spatial Spatio-temp Epidemiol 18:74-81. Google Scholar
Case Studies in Thermal Engineering. Volume 25, June 2021, 100901. Thermal pollution zones on the aquatic environment from the coastal power plant: Numerical study ... In this work the results of a numerical study of thermal pollution from the power plant operation using the Irtysh River as a natural water cooling system have been presented. In ...
An analysis of different sources of air pollution in Kolkata has revealed that motor vehicles are the leading contributor to air pollution (51.4%) which is followed by industry (24.5%) and dust particles (21.1%), respectively ( Table 1) [ 48 ]. Table 1. Sources of air pollution emissions in Kolkata.
Thermal power plants pollution assessment based on deep neural networks, remote sensing, and GIS: A real case study in Iran Mar Pollut Bull. 2023 Jul;192:115069. doi: 10.1016/j.marpolbul.2023.115069. Epub 2023 May 30. Authors Marzieh Mokarram 1 ...
Deabes E.A.M., 2020, The impact of thermal power stations on coastline and benthic fauna: Case study of El-Burullus power plant in Egypt, Results in Engineering 7: 100128. Dethier M.N., Graham E.S., Cohen S., Tear L.M., 1993, Visual versus random-point percent cover estimation: 'objective' is not always better, Marine Ecology Progress ...
The study finds that the initial surface temperature, wind speed, rainfall intensity, thermal conductivity, and the underlying surface's water permeability can explain 95.4% and 91.9% of the observed differences in surface runoff heating and temperature increase rate, respectively.
On April 12, the Tamil Nadu Pollution Control Board (TNPCB) is scheduled to conduct a public hearing for state-owned electricity utility TANGEDCO's proposal to set up the Ennore Thermal Power ...
Among the major innovations of this research is the use of satellite images to study pollution caused by thermal power plants on the water beches in south of Iran as well as the effect of temperature on ... A Case Study of Thermal Power Generation in India* (2006) Google Scholar. Mustafa et al., 2020. E.K. Mustafa, Y. Co, G. Liu, M.R. Kaloop, A ...
Overview. This study assesses Delhi's air pollution scenario in the winter of 2021 and the actions to tackle it. Winter 2021 was unlike previous winters as the control measures mandated by the Commission of Air Quality Management (CAQM) in Delhi National Capital Region and adjoining areas were rolled out. These measures included the Graded Response Action Plan (GRAP) and additional emergency ...
July 18, 2022 — Air pollution, climate change, and public health are closely linked, as a new study shows. The report details on a town-by-town basis the deaths and illnesses caused by air ...
The best case scenario is that the climate has a relatively low sensitivity to carbon dioxide. If that is the case, the new study finds that the high end of ozone measurements would - on average - go up by less than 0.3 parts per billion (ppb). Those high measurements could still be below the existing air quality standards for many locations.
A case study was carried out on the thermal power industry, China's energy consumption and pollutant emissions leader. This paper is organized as follows: Section 2 describes the technology structure details of the thermal power industry and defines technical efficiency as evaluation indicators, which are then used along with technological ...
Artificial neural networks offer a viable route in assessing and understanding the presence and concentration of heavy metals that can cause dangerous complications in the wider context of water quality prediction for the sustainability of the ecosystem. In order to estimate the heavy metal concentrations in Iznik Lake, which is an important water source for the surrounding communities ...