- Utility Menu

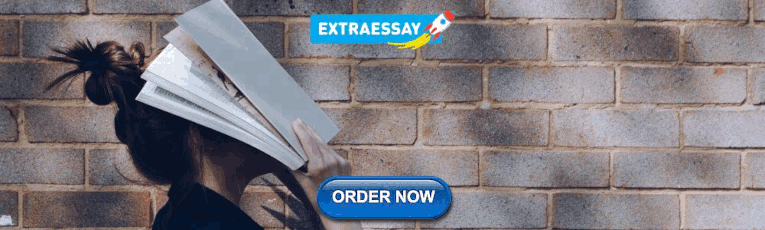
Masahiko Haraguchi
Postdoctoral fellow, harvard kennedy school, harvard university.

Critical Infrastructure Systems: A Case Study of the Interconnectedness of Risks Posed by Hurricane Sandy for New York City
Purpose This study aims to investigate the impact of Hurricane Sandy from the perspective of interdependence among different sectors of critical infrastructure in New York City and to assess the interconnected nature of risks posed by such a hurricane.
Design/methodology/approach This study uses indirect damages of each sector to estimate the degree of functional interdependence among the sectors. The study examines the impact of the hurricane on different critical infrastructures by combining hazard maps of actual inundation areas with maps of critical infrastructure. The direct damages of each sector are calculated from the inundation areas in the flood map. The indirect damages are estimated by considering the areas that were not inundated but affected by Sandy through the interconnected infrastructure.
Findings The electricity sector was the key sector to propagate risks to other sectors. The examination of new initiatives to increase the resilience of critical infrastructures in New York City after Sandy reveals that these initiatives focus primarily on building hard infrastructures to decrease direct damages. They understate the importance of interdependent risk across sectors. Future disaster risk reduction strategies must address interdependent infrastructures to reduce indirect damages.
Originality/value This paper focuses on estimating the direct and indirect damages caused by Hurricane Sandy in each critical infrastructure sector, using GIS mapping techniques. It also introduces a Bayesian network as a tool to analyze critical infrastructure interdependence.
Recent Publications
- Analysis of industrial water–energy–labor nexus zones for economic and resource-based impact assessment
- How Can a Municipal Government Continue Operations During Mega-Disasters? An Analysis of Preparedness Using Complex Adaptive Systems
- Urban waste to energy recovery assessment simulations for developing countries
- Concepts, Frameworks, and Policy Tools for Disaster Risk Management: Linking with Climate Change and Sustainable Development
- Disaster Preparedness and Complex Adaptive Systems: a Government Continuity Plan for a Self-organizing Community
- Leveraging Global and Local Data Sources for Flood Hazard Assessment and Mitigation: An Application of Machine Learning to Manila.
Open Access is an initiative that aims to make scientific research freely available to all. To date our community has made over 100 million downloads. It’s based on principles of collaboration, unobstructed discovery, and, most importantly, scientific progression. As PhD students, we found it difficult to access the research we needed, so we decided to create a new Open Access publisher that levels the playing field for scientists across the world. How? By making research easy to access, and puts the academic needs of the researchers before the business interests of publishers.
We are a community of more than 103,000 authors and editors from 3,291 institutions spanning 160 countries, including Nobel Prize winners and some of the world’s most-cited researchers. Publishing on IntechOpen allows authors to earn citations and find new collaborators, meaning more people see your work not only from your own field of study, but from other related fields too.
Brief introduction to this section that descibes Open Access especially from an IntechOpen perspective
Want to get in touch? Contact our London head office or media team here
Our team is growing all the time, so we’re always on the lookout for smart people who want to help us reshape the world of scientific publishing.
Home > Books > System of System Failures
Failures in a Critical Infrastructure System
Submitted: 11 April 2017 Reviewed: 20 July 2017 Published: 20 December 2017
DOI: 10.5772/intechopen.70446
Cite this chapter
There are two ways to cite this chapter:
From the Edited Volume
System of System Failures
Edited by Takafumi Nakamura
To purchase hard copies of this book, please contact the representative in India: CBS Publishers & Distributors Pvt. Ltd. www.cbspd.com | [email protected]
Chapter metrics overview
1,946 Chapter Downloads
Impact of this chapter
Total Chapter Downloads on intechopen.com

Total Chapter Views on intechopen.com
The purpose of this chapter is to provide a comprehensive overview of a critical infrastructure system, of failures and impacts that occur within it and of the resilience, which effectively reduces the risk of these impacts spreading on to dependent subsystems. The chapter presents a basic description of a critical infrastructure system and of the hierarchic arrangement of its subsystems and linkages between them. Critical infrastructure system failures, including their causes and impacts on dependent subsystems and on society as a whole, are presented in the following section. Particular focus is given to the propagation of impacts in a critical infrastructure system and the current approaches to their modeling. The chapter concludes by expounding on the resilience of critical infrastructure subsystems and its impact on the minimization of failures in critical infrastructure subsystems in circumstances involving emergencies.
- critical infrastructure
Author Information
David rehak.
- Faculty of Safety Engineering, VŠB - Technical University of Ostrava, Czech Republic
Martin Hromada *
- Faculty of Applied Informatics, Tomas Bata University in Zlín, Czech Republic
*Address all correspondence to: [email protected]
1. Introduction
Society has traditionally depended on a broad variety of services as much as on the infrastructures providing them. Over time, some of these infrastructures, or rather their elements considered to be of vital importance to society, began to be regarded as critical. At present, these infrastructures constitute the critical infrastructure system [ 1 ], which consists of individual subsystems, i.e., sectors, subsectors, and elements. There are dependencies between critical infrastructure subsystems which can, due to a disruption in the functionality of one subsystem, spread to dependent subsystems, and thereby escalate the impacts from emergencies on society.
2. Critical infrastructure system description
The issue of critical infrastructure protection began to be addressed in the United States in response to a terrorist bombing on a federal building in Oklahoma City in 1995 [ 2 ]. Over the following years, other countries also started tackling these problems, e.g., from 1998 in Canada and from 1999 in the United Kingdom, Germany, Sweden, and Switzerland. Following the September 11, 2001 attacks, the majority of European countries proceeded to define “Critical Infrastructure” and began to take actions aimed at its protection [ 3 ].
The US Department of Homeland Security (DHS) currently defines a critical infrastructure as “ systems and assets, whether physical or virtual, so vital to the United States that the incapacity or destruction of such systems and assets would have a debilitating impact on security, national economic security, national public health or safety, or any combination of those matters ” [ 4 ]. A critical infrastructure at the European Union level is specified in a Council Directive [ 1 ], defining a critical infrastructure as “ an asset, system or part thereof located in Member States which is essential for the maintenance of vital societal functions, health, safety, security, economic or social well-being of people, and the disruption or destruction of which would have a significant impact in a Member State as a result of the failure to maintain those functions .”
The hierarchic arrangement of a critical infrastructure system has three levels that constitute a vertical classification [ 3 ]: system level, sector level, and element level (see Figure 1 ). The system level is the basic classification of a critical infrastructure according to its functions. This level comprises two areas, namely the technical infrastructure and the socioeconomic infrastructure. The technical infrastructure includes sectors producing and providing specific commodities (e.g., energy and water supply) or sectors providing technical services (e.g., transport or ICT systems). The socioeconomic infrastructure is composed of sectors that provide social or economic services (e.g., health care, financial and currency markets, emergency services, and public administration). There are significant dependencies between two types of critical infrastructure [ 5 ]. For instance, all of the socioeconomic sectors require the unrestricted availability of commodities produced by the technical infrastructure sectors, whereas the technical infrastructure, by contrast, fully depends on the socioeconomic sectors, especially in crisis situations.

Figure 1.
Hierarchic arrangement in a critical infrastructure system.
The sector level is composed of the individual sectors and subsectors of a critical infrastructure. This level represents the classification of specific sectors and their mutual linkages. The transportation sector, for example, is made up of five subsectors, namely road transport, rail transport, air transport, inland waterways transport, and ocean and short-sea shipping and ports [ 1 ]. The individual elements that form the element level are the basic building blocks of the critical infrastructure system. These elements reach different degrees of relevance within the system, depending on the extent of the impact that their disruption or failure can potentially produce.
It is imperative that a critical infrastructure system be viewed in a comprehensive manner, taking into account its networked arrangement where individual subsystems are interlinked via various types of linkages. The basic structure of these linkages arises from their character and includes one-way linkages, which represent an influence or dependency, and two-way linkages involving interdependency. Rinaldi et al. [ 6 ] have classified interdependencies in more detail as physical, cybernetic, geographic, and logical in nature and noted that interdependencies increase the risk of failures or disruptions in multiple infrastructures. Pederson et al. [ 5 ] have subsequently further classified these linkages for lower levels of detail.
3. Impacts of critical infrastructure system failures on dependent subsystems and society
Like any other complex system, a critical infrastructure system includes a multitude of elements with different levels of importance, categorized into several levels and interconnected by linkages of various types and intensity. Such a structural arrangement leads to a broad correlation between individual subsystems, which determines the manner and intensity of propagation of impacts from critical infrastructure system failures on dependent subsystems and society.
3.1. Critical infrastructure system failures
climatological threats (including natural disasters such as floods, tornadoes, heavy snowfall, or extensive fires);
geological threats (e.g., earthquakes, volcanic activity, landslides);
biological threats (e.g., pandemics);
technological threats (including technological emergencies such as radiation emergencies, hazardous chemical spills, flooding caused by damage to hydraulic structures, widespread disruptions to engineering networks, public water supply emergencies or major road, rail, or air traffic accidents); and
criminal threats (e.g., terrorism, criminal activity, armed conflicts).
The effects produced by these threats on a critical infrastructure system or its subsystems can cause adverse events, which can in turn lead to disruptions or in extreme cases, failures of different subsystems. This involves, in particular, disruptions to functional parameters causing a decline in the performance of specific elements (see Figure 2 ) where the decline is directly proportional to the intensity of the emergency and the degree of resilience of the respective critical infrastructure element.

Figure 2.
Disruption to an element in a critical infrastructure system.
A cascading failure occurs when a disruption in one infrastructure causes the failure of element in a second infrastructure, which subsequently causes a disruption in the second infrastructure (e.g., electric power failure could create disruption in other infrastructures).
An escalating failure occurs when an existing disruption in one infrastructure exacerbates an independent disruption of a second infrastructure, generally in the form of increasing the severity or the time for recovery of the second failure (e.g., disruption in ICT network may escalate to disruption in a road transport network).
A common cause occurs when two or more infrastructure networks are disrupted at the same time: elements within each network fail because of some common cause (e.g., action of natural disaster to all local infrastructures).
Over the following years, numerous scholarly papers and studies attempting to elaborate on and tackle the issue of failure propagation within a critical infrastructure system from different viewpoints were published based on the work of Rinaldi et al. [ 6 ]. These include Visualization of Critical Infrastructure Failure [ 8 ], Cascading Effects of Common-Cause Failures in Critical Infrastructures [ 9 ], Analyzing Critical Infrastructure Failure with a Resilience Inoperability Input–Output Model [ 10 ], or Time-based critical infrastructure dependency analysis for large-scale and cross-sectoral failures [ 11 ] to name a few.
3.2. Impacts of critical infrastructure system failures
Critical infrastructure system failures subsequently produce negative impacts. These impacts can propagate further not only within the critical infrastructure system (between dependent subsystems), but also outside the system where they can specifically affect society, including national interests such as state security, the economy, and basic human needs [ 1 ].
The intensity and propagation of the impacts from critical infrastructure system failures is affected by several external and internal factors of the system concerned. While the external factors include, in particular, resilience of society and the character, and the scope and duration of an emergency; the principal internal factors include the type and scope of the failure inside the system [ 6 ], subsystem linkages, and subsystem resilience. The nature of the impacts is characterized by the scope, structure, intensity, duration, and effect of the emergency (see Figure 3 ) [ 3 ].

Figure 3.
Aspects that create the character of impacts in a critical infrastructure system [ 3 ].
In the event of a disruption to a critical infrastructure system, the impacts spread into two basic areas. The first instance involves impacts within the system where the failure of one critical infrastructure subsystem causes a failure of another subsystem in what is known as a cascading effect [ 6 ]. In the second instance, the impacts exert influence outside the system, specifically, on society, producing negative effects on national interests such as security, the economy, and basic human needs [ 3 ].
In both of the above-mentioned cases, the impacts may be classified as direct or indirect from a structural point of view. The immediate effect of a disrupted subsystem on another subsystem or directly on society is considered to be a direct or primary action. In contrast, indirect effects of impacts occur vicariously through any critical infrastructure subsystem, regardless of whether or not they affect another subsystem or society as a result. Indirect effects of impacts may be secondary (through one subsystem) or multi-structural (through several subsystems) in character [ 3 ].
Other important factors determining the character of impacts are their intensity and duration. The impact intensity depends on the extent of a failure in a subsystem, that in turn affects another critical infrastructure subsystem, as well as on the level of their linkage. If the linkage is weak, the impact intensity is low and the subsequent impact on the affected subsystem is limited. However, if this linkage is strong, the impact intensity is high and the impact on the affected subsystem can be devastating or absolute. The impact duration, which may be short-term, medium-term, or long-term, represents an important variable with respect to the impact intensity. Ouyang et al. [ 12 ] present the typical time progression of a critical infrastructure disruption, dividing it into prevention, propagation, damage, assessment, and recovery periods [ 3 ].
Another key factor determining the character of impacts is the effect of their action. If the impacts of a disrupted subsystems act on another subsystem or society in one way only, the impact effect can be regarded as a single impact. However, if the impact effects are multi-way (e.g., through the combination of direct and indirect impacts) and occur concurrently in real-time, then the effects are considered to be synergistic [ 3 ].
3.3. Propagation of impacts in a critical infrastructure system
The above-mentioned aspects, shaping the character of impacts, also significantly contribute to the propagation of these impacts in a critical infrastructure system. At the core of their propagation lie critical infrastructure system failures caused by the negative effects of security risks (i.e., causes of disruptions or failures of a critical infrastructure), which can be either external or internal in nature. Such impacts can then exert a direct influence on society (i.e., direct impacts), spread further across the critical infrastructure, and cause other failures, which lead to additional impacts (i.e., cascading impacts) or they can, due to a cascading effect, act jointly on a single target (i.e., synergistic impacts). See Figure 4 for a graphical representation of all the potential ways in which impacts can propagate within a critical infrastructure system.

Figure 4.
Ways of impact propagation in a critical infrastructure system.
Direct impacts are impacts caused by the disruption or failure of a critical infrastructure subsystem, which act directly on society. The effects of a security threat (e.g., a terror attack) to a component of a critical road infrastructure of international importance (e.g., a major freeway bridge) can be used as an example. These negative effects result in the disruption to the functional parameters of the freeway, which has a direct impact on society (in this instance on passengers and freeway network operators).
Cascading impacts are impacts caused by the disruption or failure of a critical infrastructure subsystem, which spread further across the critical infrastructure, resulting in failures in dependent subsystems that in turn lead to an escalation in other impacts. The effects of a security threat (e.g., a gale) to a component of a critical electric energy infrastructure of national importance (e.g., 110 kV distribution system) can be used as an example. These negative effects result in the disruption to functional parameters of the distribution system, which cascades into dependent subsystems (e.g., a railroad signaling system). The disruption to a distribution system then results in a cascading impact on society due to nonfunctioning railroad transport.
Synergistic impacts are impacts caused by the disruption or failure of two or more critical infrastructure subsystems which occur concurrently, thereby exacerbating their impacts on society [ 3 , 9 ]. The effects of a security threat (e.g., a technological accident) to element of a critical electric energy infrastructure of international importance (e.g., a nuclear power plant) can be used as an example. These negative effects result not only in direct impacts on society (i.e., large-scale power outages), but also in the impacts cascading to dependent subsystems (e.g., heat production and distribution), the disruption of which produces additional impacts on society. This situation brings about a synergistic effect, consisting of the added effect of joint impacts on society, and increasing their mere sum [ 3 ].
3.4. Modeling of impacts of critical infrastructure system failures
early indication of impacts using a bottom-up approach;
harmonization and transformation of cross-cutting criteria at the regional level;
European critical infrastructure risk and safety/security management; and
implementation of a preferential critical infrastructure risk assessment.
An early indication of impacts through the application of a bottom-up approach should be based on the determination of resilience disruption indicators in interconnected critical infrastructure subsystems. It is a holistic approach to assess the resilience of a critical infrastructure based on a comprehensive perception of specific political, economic, social, technological, legislative, and ecological environments. The essence of this approach is a systematic approach consisting of a cross-sectoral evaluation based on a research into the mutual linkages between individual critical infrastructure subsystems. It factors in the propagation of cascading impacts and synergistic effects in a critical infrastructure system. The referenced system solution should be applied using a progressive bottom-up approach, which is based on a critical infrastructure evaluation from the lowest level (city, region) upwards and has already been implemented in a number of developed countries (e.g., Switzerland and the Netherlands). This approach can be viewed as the logical continuation of the ongoing research into critical infrastructure security in terms of integrating the research results, via identifiers describing the critical infrastructure status, into a composite resilience indicator (see Figure 5 ) [ 13 ].

Figure 5.
Development of the approach to a critical infrastructure research [ 14 ].
health impacts—the number of victims with a threshold value of more than 25 fatalities or more than 250 individuals hospitalized for a period exceeding 24 hours per 1 million inhabitants within the region under review;
economic impacts with an economic loss threshold value of over 0.5% of gross domestic product; and
impacts on the public with a threshold value of more than 12,500 individuals per 1 million inhabitants within the region under review affected by extensive restrictions in the provision of essential services or by other major disruptions to everyday life.
A provisional transformation of national criteria could form the basis for the setting of cross-cutting criteria values at the regional level (note: however, this method of setting regional values is not ideal in terms of applying the bottom-up approach as it is more akin to the top-down approach due to the transformation of national criteria). The transformation involves the dynamic conversion of threshold values for national cross-cutting criteria to regional criteria. This ratio is mainly applied as a proportion of the population of a given state to the population of the region concerned, and of the threshold values of national cross-cutting criteria to those of regional cross-cutting criteria. In principal, static threshold values are converted to dynamic values not only due to the varying population sizes in different regions, but also due to the different levels of gross domestic product generated in these regions [ 17 ].
Methodology for selected CIs system resilience element evaluation [ 18 ]; and
Methodology for ensuring the protection of CIs in the production, transmission, and distribution of electricity [ 19 ].
Implementation of a preferential critical infrastructure risk assessment provides another important basis for the modeling of impacts produced by critical infrastructure failures [ 20 ]. This allows the assessor to introduce subjective conditions into an otherwise objective process of risk assessment, providing the assessor with an option to partially influence the assessment process by preferring certain factors over others. The significance of this phase of the assessment process lies in the fact that different entities perceive certain risks from different points of view, which creates a conducive environment for discussion of all stakeholders, ensuring the most appropriate safety/security actions are taken. Moreover, a preferential critical infrastructure risk assessment also provides an important basis for the modeling of impacts of critical infrastructure failures as its results determine vulnerabilities enabling the propagation of impacts throughout the critical infrastructure system [ 13 ].
4. Resilience of critical infrastructure subsystems
The purpose of each critical infrastructure subsystem is to deliver services to recipients. It is therefore essential to ensure that each subsystem is fully functional and that appropriate steps are taken to minimize its failures and curtail the propagation of any potential impacts on society or any other dependent critical infrastructure subsystems. According to existing scientific knowledge, the best and most effective way of minimizing the impacts of critical infrastructure system failures is to reach the highest possible level of resilience with respect to all of its subsystems.
4.1. Definition of resilience
The term resilience was first defined in connection with the resistance and stability of ecological systems where two types of system behavior were identified [ 21 ]. The first type, stability, is the ability of a system to return to an equilibrium state after a temporary disturbance and the more rapidly it returns, the more stable it is. The second type of system behavior, known as resilience, is a measure of the ability of a system to absorb impacts without significant changes to the system status. Over time, this perspective was expanded to include the sphere of sociology, which then led to resilience being explored in socio-ecological systems. Based on the achieved results, the research into resilience gradually spread to other disciplines such as psychology, economy, and engineering.
In 2001, Holling shed light on understanding the complexity of economic, ecological, and social systems with the publication of a definition based on two fundamental components of each system, namely hierarchy and adaptive cycles [ 22 ]. Together they form panarchy according to Holling. Panarchy can be defined as a structure in which systems of nature and humans are interlinked in never-ending adaptive cycles of growth, accumulation, restructuring, and renewal.
The research into the resilience of socio-ecological systems also sparked an interest in research focused on resilience in society. The resilience of a society is dependent on its ability to respond to a stress factor and can be defined as “ The ability of a system, community or society exposed to hazards to resist, absorb, accommodate, adapt to, transform and recover from the effects of a hazard in a timely and efficient manner, including through the preservation and restoration of its essential basic structures and functions through risk management” [ 23 ].
Resilience gradually began to be defined in general terms for any system, including engineering. Resilience was first described in connection with a critical infrastructure in a document entitled Critical Infrastructure Resilience Final Report and Recommendations [ 24 ], where it is defined as the ability to absorb, adapt to, and/or rapidly recover from a potentially disruptive event. By contrast, the critical infrastructure resilience strategy [ 25 ] defines critical infrastructure resilience as the ability to reduce the magnitude and/or duration of a disruptive event. These definitions clearly show what constitutes resilience, or rather what characteristics enhance the resilience of a system. For example, Chandra [ 26 ], based on his study of socio-ecological systems, includes the following attributes in engineering systems resilience: redundancy, adaptability, flexibility, interoperability, and diversity.
Ehlen et al. [ 27 ]—absorption, adaptation, and recovery.
Keeping the country running [ 28 ]—the ability to anticipate, absorb, adapt, and/or rapidly recover. For the system to function as a whole, it must incorporate four assets or elements: resistance, reliability, redundancy, response, and recovery.
Carlson et al. [ 29 ]—the form of linkages between six aspects (anticipation, resistance, absorption, ability to respond, adaptability, and recovery), which according to the author define resilience, and four parameters (preparedness, mitigation, response, and recovery), which characterize the process of enhancing the resilience capacity of a system.
Béné et al. [ 30 ]—three basic aspects: absorptive capacity (the ability to cope with the impacts of adverse changes and shocks), adaptive capacity (the ability of a system to adapt to changes), and transformative capacity (the ability to create a fundamentally new system).
Presidential Policy Directive—Critical Infrastructure Security and Resilience [ 31 ]—the ability to prepare, resist, and rapidly recover.
Hromada et al. [ 32 ]—preparedness and adaptability as the basis for the fulfillment of the resilience function. Key indicators: robustness, preparedness, ability to respond, recoverability.
Eid et al. [ 33 ]—the ability to anticipate, resist, absorb, respond, adapt, and rapidly recover from a disruption.
Ortiz De La Torre et al. [ 34 ]—prepare, prevent, and protect (before the disruption), mitigate, absorb and adapt (during the disruption), and respond, recover and learn (after the disruption).
Bologna et al. [ 35 ]—the overall activities of modeling, and analysis of critical infrastructure system aimed to evaluate the ability to prevent, absorb, adapt, and recover from a disruptive event, either natural or man-made.
Nan and Sansavini [ 36 ]—ability of the system to withstand a change or a disruptive event by reducing the initial negative impacts (absorptive capability), by adapting itself to them (adaptive capability), and by recovering from them (restorative capability).
Some experts consider critical infrastructure resilience to be the primary national policy framework and a vital criterion for the future sustainability of cities or infrastructures as such, and argue that, from a broader perspective, resilience is indispensable in terms of population protection and crisis management [ 33 , 37 ].
4.2. Concept of critical infrastructure resilience
Based on the accepted definitions, resilience can be said to represent the level of internal preparedness of critical infrastructure subsystems for emergencies or the ability of these subsystems to perform and maintain their functions when negatively affected by internal and/or external factors. Strengthening resilience (e.g., Action Plan for Critical Infrastructure [ 38 ] or Labaka et al. [ 39 ]) minimizes the vulnerability of subsystems, which in turn curtails the occurrence, intensity, and propagation of failures and their impacts in a critical infrastructure system and society.
Understanding and clear definition of resilience represent the cornerstone of resilience assessment and strengthen with respect to critical infrastructure subsystems. In fact, critical infrastructure system resilience must be understood as a cyclic process based on continual strengthening of resilience of individual subsystems (see Figure 6 ). The crucial phases of this process are prevention, absorption, recovery, and adaptation.

Figure 6.
Cycle of critical infrastructure resilience.
The first phase of the critical infrastructure resilience cycle is prevention. In individual critical infrastructure subsystems, this is determined by permanent preparedness and protection of each subsystem. Prevention is provided on a continuous basis until a subsystem disruption occurs, at which time it is suspended, and for the duration of the emergency, replaced by absorption.
Absorption, the second phase of the resilience cycle, is initiated if a subsystem is disrupted due to an emergency and is determined by the critical infrastructure subsystem robustness. Accordingly, robustness is determined by the ability of a critical infrastructure element to absorb the effects of an emergency. In a critical infrastructure system, two types of robustness are recognized, namely structural and security robustness. Structural robustness is determined by the progress in the decline of a function and the level of redundancy, while security robustness is based on the level of protective measures, detection, and ability to respond.
The recovery phase starts after the effects of an emergency have worn off. This phase is characterized by recoverability, which is the capacity of a subsystem to recover its function to the required level of performance after the effects of an emergency no longer exist. The success of recovery is determined by the available resources and the time required to complete the recovery process.
The final phase of the critical infrastructure resilience cycle is adaptation, which is essentially the ability of an organization to adapt a subsystem to subsequent effects of an emergency. It represents the dynamic long-acting ability of an organization to adapt to changes in circumstances. Adaptation is determined by the internal processes of an organization focused on the strengthening of resilience, i.e., risk management and innovation/education processes. However, strengthening of the resilience of a subsystem already occurs during the recovery phase of its performance.
4.3. Resilience assessment in a critical infrastructure system
The Resilience Assessment and Evaluation of Computing Systems compilation monograph [ 40 ] was the first comprehensive overview study exploring critical infrastructure resilience assessment in the field of information and communication technology. Another important monograph, Critical Infrastructure System Security and Resiliency [ 41 ], introducing a practical methodology for the development of an efficient system of critical infrastructure protection, was published a year later. This methodology focuses both on the prevention of emergencies and the mitigation of its consequences. The same year saw the publication of the Resilience Measurement Index: An Indicator of Critical Infrastructure Resilience study [ 42 ], whose main objective is to measure the ability of a critical infrastructure to reduce the magnitude and/or duration of impacts from disruptive events.
In 2013, the European Commission published a working document on a new approach to the European Programme for Critical Infrastructure Protection: Making European Critical Infrastructures more secure [ 43 ]. This document clearly emphasized the importance of resilience and interdependencies in a critical infrastructure as well as the need to develop tools and methods for their assessment.
In addition, the issue of measuring critical infrastructure resilience has long been explored by the Swiss Federal Institute of Technology in Zurich (Eidgenössische Technische Hochschule Zürich). The institute presents the results of its risk and resilience research in the form of scientific reports, with the issue of resilience measurement addressed in detail in the SKI Focus Report 8: Measuring Resilience [ 44 ] and the SKI Focus Report 9: Measuring Critical Infrastructure Resilience [ 45 ].
There are also several major international projects dealing with critical infrastructure resilience assessment at present, including SMART RESILIENCE: Smart Resilience Indicators for Smart Critical Infrastructures, IMPROVER: Improved Risk Evaluation and Implementation of Resilience Concepts to Critical Infrastructure, RESILIENS: Realizing European Resilience for Critical Infrastructure, or RESILIENCE 2015: Dynamic Resilience Evaluation of Interrelated Critical Infrastructure Subsystems.
In 2016, a comprehensive approach based on the results of leading research projects was published in the Guidelines for Critical Infrastructures Resilience Evaluation document [ 35 ]. This approach has its basis in the evaluation of individual indicators constituting resilience, the resulting composite indicator being a function of indicators in technical (i.e., prevention, absorption, adaptation, and recovery), personal, organizational, and cooperative dimensions.
Additional significant approaches to evaluate resilience have been presented, for example, in an interim report of the project RESILIENS D2.2: Qualitative, Semi-Quantitative and Quantitative Methods and Measures for Resilience Assessment and Enhancement [ 34 ]. The second part of the document presents a critical infrastructure resilience assessment tool (CI-RAT), which has been developed as part of this project and is based on a semi-quantitative methodology for CI resilience assessment, and on a CI resilience management concept.
5. Conclusion
The chapter entitled “Failures in a Critical Infrastructure System” presents a comprehensive overview of a critical infrastructure system, which may be regarded as the basis for ensuring the functional continuity of society from both the economic and social perspectives. The introductory part of the chapter is designed as a historical framework, defining critical infrastructures in relation to legislative, normative, and institutional processes involved in addressing the issues concerned. The described framework formulates the basis, approaches, and logic of a hierarchical system arrangement in connection to interdependencies and linkages between elementary elements. Infrastructure failures have been classified in terms of their sources and causes because the potential impacts of failures in selected dependent systems can have profound effects on the functioning of society as a whole. It was argued that the impacts of failures in dependent systems increase the occurrence of cascading and synergistic effects, which fundamentally affect the resilience of individual elements and the general function of the system. This led to establishing the relationship between system resilience and failures with respect to critical infrastructure network elements.
Based on these facts, the impacts of failures and their propagation were described in the context of the necessity to model such impacts. In this regard, the significance and applicability of top-down and bottom-up approaches in relation to the exploration of mutual linkages was further compared as one of the identifiers describing the critical infrastructure status. The significance of identifying and labeling critical infrastructure elements is, therefore, also viewed from the perspective of the need for a more objective setting of cross-cutting criteria values, equally applicable at the regional level. As already mentioned, element resilience exerts a substantial effect on the overall impacts of potential failures. That is why a resilience framework for critical infrastructure subsystems was established with a view to defining resilience, formulating a resilience concept, and setting up a resilience evaluation process in a critical infrastructure system. The presented facts are based on the Ministry of the Interior of the Czech Republic Security Research project—RESILIENCE 2015: Dynamic Resilience Evaluation of Interrelated Critical Infrastructure Subsystems and form a resilience knowledge base as the ability of a system, community, or society exposed to adverse events to resist, absorb, accommodate, adapt to, transform, and recover from the effects of a hazard in a timely and efficient manner, including through the preservation and recovery of its essential basic structures and functions through risk management.
Acknowledgments
The chapter has been elaborated within the project of the Ministry of the Interior of the Czech Republic filed under VI20152019049 and entitled “Dynamic Resilience Evaluation of Interrelated Critical Infrastructure Subsystems.”
- 1. European Union. Council Directive 2008/114/EC of 8 December 2008 on the Identification and Designation of European Critical Infrastructures and the Assessment of the Need to Improve their Protection
- 2. Pesch-Cronin KA, Marion NE. Critical Infrastructure Protection, Risk Management, and Resilience: A Policy Perspective. London, United Kingdom: Taylor & Francis Group; 2017. p. 366
- 3. Rehak D, Markuci J, Hromada M, Barcova, K. Quantitative evaluation of the synergistic effects of failures in a critical infrastructure system. International Journal of Critical Infrastructure Protection. 2016; 14 :3-17. DOI: 10.1016/j.ijcip.2016.06.002
- 4. The Department of Homeland Security. The National Infrastructure Protection Plan 2013: Partnering for Critical Infrastructure Security and Resilience. Washington, DC, USA: U.S. Department of Homeland Security; 2013. p. 50
- 5. Pederson P, Dudenhoeffer D, Hartley S, Permann M. Critical Infrastructure Interdependency Modeling: A Survey of U.S. and International Research. Idaho Falls, ID, USA: Idaho National Laboratory; 2006. p. 116
- 6. Rinaldi SM, Peerenboom JP, Kelly TK. Identifying, understanding and analyzing critical infrastructure interdependencies. IEEE Control Systems Magazine. 2001; 21 (6):11-25. DOI: 10.1109/37.969131
- 7. Rehak D, Martinek B, Ruzickova P. Population Protection in the Context of Current Security Threats. Ostrava, Czech Republic: SPBI; 2015. p. 131
- 8. Wilde WD, Warren MJ. Visualisation of critical infrastructure failure. In: Australian Information Warfare and Security Conference. Perth, Western Australia: Edith Cowan University; 2008. pp. 48-63. DOI: 10.4225/75/57a82a75aa0de
- 9. Kotzanikolaou P, Theoharidou M, Gritzalis D. Cascading effects of common-cause failures in critical infrastructures. In: Butts J, Shenoi S, editors. Critical Infrastructure Protection VII. Berlin, Germany: Springer; 2013. pp. 171-182. DOI: 10.1007/978-3-642-45330-4_12
- 10. Jonkeren O, Giannopoulos G. Analysing critical infrastructure failure with a resilience inoperability input–output model. Economic Systems Research. 2014; 26 (1):39-59. DOI: 10.1080/09535314.2013.872604
- 11. Stergiopoulos G, Kotzanikolaou P, Theocharidou M, Lykou G, Gritzalis D. Time-based critical infrastructure dependency analysis for large-scale and cross-sectoral failures. International Journal of Critical Infrastructure Protection. 2016; 12 :46-60. DOI: 10.1016/j.ijcip.2015.12.002
- 12. Ouyang M, Dueñas-Osorio L, Min X. A tree-stage resilience analysis framework for urban infrastructure systems. Structural Safety. 2012; 36-37 :23-31. DOI: 10.1016/j.strusafe.2011.12.004
- 13. Rehak D, Novotny P. Bases for modelling the impacts of the critical infrastructure failure. Chemical Engineering Transactions. 2016; 53 :91-96. DOI: 10.3303/CET1653016
- 14. Rehak D, Hromada M, Ristvej J. Indication of critical infrastructure resilience failure. In: Čepin M, Briš R, editors. Safety and Reliability—Theory and Application (ESREL). Florida, London, United Kingdom: CRC Press; 2017. pp. 963-970
- 15. RAIN Project [Internet]. 2015. Available from: http://rain-project.eu/about/the-scope-of-the-project/ [Accessed: 2017-07-01]
- 16. Government Decree 432/2010 of 22 December 2010 on Criteria for Determination of the Critical Infrastructure Element
- 17. Novotny P, Markuci J, Rehak D. Determination of the critical infrastructure elements at regional level. Spektrum. 2014; 14 (1):54-59
- 18. Hromada M. Information support system development in relation to critical infrastructure element resilience evaluation. In: International Conference on Emerging Security Information, System and Technologies (SECURWARE); 24-28 July 2016; Nice, France. Wilmington, DE, USA: IARIA; 2016. pp. 174-184
- 19. Methodology to Ensure of Critical Infrastructure Protection in the Area of Electricity Generation, Transmission and Distribution. Prague, Czech Republic: Deloitte Advisory; 2012. p. 55
- 20. Rehak D, Senovsky P. Preference risk assessment of electric power critical infrastructure. Chemical Engineering Transactions. 2014; 36 :469-474. DOI: 10.3303/CET1436079
- 21. Holling CS. Resilience and stability of ecological systems. Annual Review of Ecology and Systematics. 1973; 4 :1-23. DOI: 10.1146/annurev.es.04.110173.000245
- 22. Holling CS. Understanding the complexity of economic, ecological, and social systems. Ecosystems. 2001; 4 (5):390-405. DOI: 10.1007/s10021-001-0101-5
- 23. United Nations Office for Disaster Risk Reduction. Terminology on Disaster Risk Reduction [Internet]. 2007. Available from: https://www.unisdr.org/we/inform/terminology [Accessed: 2017-07-15]
- 24. Critical Infrastructure Resilience Final Report and Recommendations. Washington, DC, USA: National Infrastructure Advisory Council; 2009. p. 54
- 25. Critical Infrastructure Resilience Strategy. Sydney, Australia: Commonwealth of Australia; 2010. p. 34
- 26. Chandra A. Synergy between biology and systems resilience [thesis]. Rolla, MO, USA: Missouri University of Science and Technology; 2010. p. 134. Available from: http://scholarsmine.mst.edu/cgi/viewcontent.cgi?article=7727&context=masters_theses
- 27. Ehlen MA, Vugrin ED, Warren DE. Overcoming challenges in critical infrastructure resilience analysis: A new framework for resilience assessments. In: Workshop on Grand Challenges in Modeling, Simulation, and Analysis for Homeland Security (MSAHS-2010); 17-18 March 2010; Washington, DC, USA: U.S. Department of Homeland Security; 2010
- 28. Cabinet Office. Keeping the Country Running: Natural Hazards and Infrastructure. London, United Kingdom: Cabinet Office; 2011. p. 98
- 29. Carlson L, Bassett G, Buehring W, Collins M, Folga S, Haffenden B, Petit F, Phillips J, Verner D, Whitfield R. Resilience: Theory and Application. Argonne, IL, USA: Argonne National Laboratory; 2012. p. 60. DOI: 10.2172/1044521
- 30. Béné C, Wood RG, Newsham A, Davies M. Resilience: New Utopia or New Tyranny? Reflection about the potentials and limits of the concept of resilience in relation to vulnerability reduction programmes. IDS Working Papers. 2012; 2012 (405):1-61. DOI: 10.1111/j.2040-0209.2012.00405.x
- 31. The White House. Presidential Policy Directive 21 (PPD-21): Critical Infrastructure Security and Resilience. Washington, DC, USA: The White House; 2013
- 32. Hromada M, Lukas L, Matejdes M, Valouch J, Necesal L, Richter R, Kovarik F. The System and Approach to Critical Infrastructure Resilience Evaluation. Ostrava, Czech Republic: SPBI; 2014. p. 177
- 33. Eid M, Serafin D, Barbarin Y, Kuligowska E, Soszyńska-Budny J, Kolowrocki K. A resilience model based on Stochastic Poison Process. In: Summer Safety and Reliability Seminars (SSARS); 21-27 June 2015; Gdansk, Poland. 2015. DOI: 10.13140/RG.2.1.2418.3766
- 34. Ortiz De La Torre P, et al. Qualitative, Semi-Quantitative and Quantitative Methods and Measures for Resilience Assessment and Enhancement (Project Report). Dublin, Ireland: Future Analytics; 2016. p. 138
- 35. Bologna S, Carducci G, Bertocchi G, Oliva G, Traballesi A, Carrozzi L, Cavallini S, Lazari A. Guidelines for Critical Infrastructures Resilience Evaluation. Roma, Italy: Italian Association of Critical Infrastructures’ Experts; 2016. p. 101
- 36. Nan C, Sansavini G. A quantitative method for assessing resilience of interdependent infrastructures. Reliability Engineering & System Safety. 2017; 157 :35-53. DOI: 10.1016/j.ress.2016.08.013
- 37. Gross B, Weichselgartner J. Modernes Risikomanagement: Zwischen Robustheit und Resilienz. Bevölkerungsschutzmagazin. 2015; 1 :12-17
- 38. Public Safety Canada. Action Plan for Critical Infrastructure (2014-2017). Ottawa, Canada: Public Safety Canada; 2014. p. 14
- 39. Labaka L, Hernantes J, Sarriegi JM. A framework to improve the resilience of critical infrastructures. International Journal of Disaster Resilience in the Built Environment. 2015; 6 (4):409-423. DOI: 10.1108/IJDRBE-07-2014-0048
- 40. Wolter K, Avritzer A, Vieira M, van Moorsel A, editors. Resilience Assessment and Evaluation of Computing Systems. Berlin, Germany: Springer Heidelberg; 2012. p. 490. DOI: 10.1007/978-3-642-29032-9
- 41. Biringer B, Vugrin E, Warren D. Critical Infrastructure System Security and Resiliency. London, United Kingdom: CRC Press; 2013. p. 229. DOI: 10.1201/b14566
- 42. Petit F, Bassett G, Black R, Buehring W, Collins M, Dickinson D, Fisher R, Haffenden R, Huttenga A, Klett M, Phillips J, Thomas M, Veselka S, Wallace K, Whitfield R, Peerenboom J. Resilience Measurement Index: An Indicator of Critical Infrastructure Resilience. Argonne, IL, USA: Argonne National Laboratory; 2013. p. 56
- 43. European Union. Commission Staff Working Document on a new approach to the European Programme for Critical Infrastructure Protection Making European Critical Infrastructures more secure. Brussels, Belgium: European Commission; 2013. p. 17
- 44. Prior T, Hagmann J. SKI Focus Report 8: Measuring Resilience. Zurich, Switzerland: Eidgenössische Technische Hochschule Zürich; 2012. p. 25
- 45. Prior T. SKI Focus Report 9: Measuring Critical Infrastructure Resilience. Zurich, Switzerland: Eidgenössische Technische Hochschule Zürich; 2015. p. 13
© 2017 The Author(s). Licensee IntechOpen. This chapter is distributed under the terms of the Creative Commons Attribution 3.0 License , which permits unrestricted use, distribution, and reproduction in any medium, provided the original work is properly cited.
Continue reading from the same book
Published: 09 May 2018
By Datu Buyung Agusdinata
1262 downloads
By Takafumi Nakamura
955 downloads
By Steven H. Voldman
1570 downloads
- Tools and Resources
- Customer Services
- Contentious Politics and Political Violence
- Governance/Political Change
- Groups and Identities
- History and Politics
- International Political Economy
- Policy, Administration, and Bureaucracy
- Political Anthropology
- Political Behavior
- Political Communication
- Political Economy
- Political Institutions
- Political Philosophy
- Political Psychology
- Political Sociology
- Political Values, Beliefs, and Ideologies
- Politics, Law, Judiciary
- Post Modern/Critical Politics
- Public Opinion
- Qualitative Political Methodology
- Quantitative Political Methodology
- World Politics
- Back to results
- Share This Facebook LinkedIn Twitter
Article contents
Critical infrastructure disruption and crisis management.
- Eric Stern Eric Stern College of Emergency Preparedness, Homeland Security, and Cybersecurity, University at Albany
- and Brian Nussbaum Brian Nussbaum College of Emergency Preparedness, Homeland Security and Cybersecurity, University at Albany, State University of New York
- https://doi.org/10.1093/acrefore/9780190228637.013.1603
- Published online: 20 June 2022
Explicitly considering major critical infrastructure disruptions from the perspective of crisis/crisis management enables policymakers, analysts, and researchers to draw inspiration from an extensive multidisciplinary literature. Furthermore, this approach takes infrastructure failures or disruptions, and provides crucial institutional, economic and social context that is too often ignored when such challenges are treated as exclusively technical problems. The added value from this approach enables analysts and decision makers to understand the complexity of such failures and consider the many levers—technical, economic and social—that might be used to respond to them. Attempts to understand infrastructure failures as crises are not new, but the literature—like the field of practice—is to some extent underdeveloped and continuously evolving (e.g., with regard to the challenges associated with cybersecurity), generating a need for a more comprehensive approach to understanding the leadership tasks associated with the management of such crisis events in dynamic and complex organizational environments.
- critical infrastructure
- crisis analysis
- crisis management
- cybersecurity
- crisis leadership
Critical Infrastructure Disruption as Crisis
Among the most developed countries, there is an increasing awareness of growing dependencies on and vulnerabilities to disruptions of a number of key, interconnected infrastructure systems and their associated supply chains. Such disruptions tend to create chains of cascading consequences with profound effects on vital societal functions ( Brown et al., 2004 ; Leavitt & Kiefer, 2006 ; Rinaldi et al., 2001 ). Whereas there are many potentially fruitful ways of approaching the challenge of identifying risks to such critical infrastructures and improving the resilience of critical infrastructure systems, one potentially fruitful avenue is to view disruptions of critical infrastructure systems as strategic and operational crises involving diverse sets of actors and stakeholders including both governmental and nongovernmental actors ( Boin & McConnell, 2007 ; Newlove et al., 2003 ; cf. Organisation for Economic Cooperation and Development [OECD], 2015 ).
Viewing infrastructure disruptions, failures, and attacks as crises to be managed offers some important and valuable new perspectives complementing more established approaches to infrastructure risk management. Risk management approaches are a widely adopted tack to conceptualizing potential infrastructure fragility. For example, the U.S. Department of Homeland Security (DHS), through its National Infrastructure Protection Plan (NIPP), has adopted the commonly used risk model that frames risk as a function of “threat,” “vulnerability,” and “consequence.” The first of five goals listed in the 2013 NIPP update is to “assess and analyze threats to, vulnerabilities of, and consequences to critical infrastructure to inform risk management activities” ( DHS, 2013 ). This tripartite model of risk is actually drawn from work done by Sandia National Laboratory on adversarial infrastructure risk as well as work by Mary Garcia on physical security risks ( Baker et al., 2002 ), and it is an expansion and variation on the Kaplan and Garrick model of risk as a function of “likelihood” and “consequence” ( Kaplan & Garrick, 1981 ). The reader should note that by incorporating more explicitly the ideas of politics, policy-making processes, and nontechnical drivers of decision-making such as public opinion and cultural values, the crisis management perspective imparts additional information about both constraints on decision makers and the opportunities they face during and after an infrastructure disruption.
Whereas models of risk management of this kind are very valuable for thinking about the management of infrastructure risks, they have limitations. The Sandia and DHS versions, at least are really tailored for thinking about adversarial risks rather than naturally or accidentally occurring ones. In addition, all three models are very useful for thinking about risk before something bad happens, but none of them puts thinking about response and reaction to infrastructure failure (or even really distinguishing between efforts before and after such events) center stage. It is not the case that these different models of risk are not compatible; in fact they are potentially complementary.
A long and growing list of major events—adding to the growing body of cases documented in the literature—clearly demonstrates the severe disruptions as well as the domestic/international policy and organizational challenges associated with critical infrastructure crises. All three of the major U.S. hurricanes of the fall 2017 season (Harvey, Irma, and Maria) involved profound and in some instances extremely prolonged disruptions of critical infrastructure systems, including electrical power and fuel distribution, mobile communications and Internet, food and water distribution systems, healthcare, and transportation. 1 These cases also provide vivid examples of cascading effects producing potentially devastating and even life-threatening downstream effects of critical infrastructure disruption. For example, storm-related disruption of the power grid and both primary and secondary cooling systems at the Arkema chemical plant in Texas occurred. This created a secondary spin-off crisis when it became impossible to maintain safe temperatures for volatile chemical compounds stored at the facility, resulting in a series of uncontrolled explosions at the plant and the release of noxious and potentially toxic fumes. 2 Parallel, although perhaps more subtle, deadly risks emerged in the healthcare system, as power and supply chain failures affected hospital and nursing home functionality, critical care systems (e.g., dialysis for patients with kidney failure), pharmaceutical supply chains, and so on. Many of these patterns of disruption were prominent across the myriad major disasters of 2020 and 2021 and not least in Hurricane Ida, which took lives and wrought havoc with critical infrastructure systems from the Gulf Coast to New York and New Jersey.
Furthermore, the crisis perspective is promising from a policy and preparedness enhancement perspectives for a number of reasons. Disruption of critical infrastructure systems can be considered not only a direct cause of crises. They are also a common consequence of the occurrence of many other types of natural and man-made hazards and threats such as extreme terrestrial and space weather events, terrorism, major industrial or construction accidents, cyberattacks on healthcare data systems. 3 Disruption also takes place as a result of unintentional human causes such as neglect, deferred maintenance, underfunding, and workforce challenges. In addition, this perspective offers additional hitherto unidentified origins of and pathways to disruptions; they may arise from deliberate civil and system protection measures (e.g., protective shutdowns in advance of extreme weather or to minimize wildfire risk) and public reactions (crisis-related citizen usage surges that disrupt telecommunications systems, hoarding behaviors that disrupt supply chains, spontaneous or poorly planned evacuations that dangerously disrupt road transportation) as well as from the hazards themselves. Furthermore, major societal crises can be initially triggered due to processes and factors internal to critical infrastructure systems that cause them to fail, triggering a variety of secondary failures and disruptions of systems and societal functions linked to them. In fact, not only can disruption of critical infrastructure systems be either cause or consequence of a crisis, but they can also serve as crisis intensifiers that can spread a crisis to additional domains; generate additional complex problem sets characterized by tensions among core societal values; proliferate uncertainty; and increase time pressures experienced by decision makers in the public, private, and nonprofit sectors. The reader should note that these intensifiers coincide with the components of a widely used and practically relevant definition of crisis.
Explicitly considering major critical infrastructure disruptions from the perspective of crisis/crisis management enables policymakers and analysts to draw inspiration from an extensive multidisciplinary literature. Similarly, it takes infrastructure failures or disruptions and provides the institutional and social context that is too often ignored when such challenges are treated as merely technical problems, rather than situated in the sociotechnical system literature that recognizes that “infrastructures are not merely large systems, but sociotechnical institutions” ( Edwards, 2003 ).
Thus it is important and valuable to frame critical infrastructure failure or disruption as both a cause and consequence of crises, examine how such interruptions can exacerbate the elements of a crisis and make the related decisions more complex, and finally to situate such events in the crisis studies literature. This is not the first paper to do this (cf. Boin & McConnell, 2007 ; Michel‐Kerjan, 2003 ; Roe, 2016 ); however, it intends to do so in a novel way, by (a) incorporating additional insights about the profound implications of cybersecurity concerns and (b) having a particular focus on leadership tasks associated with the management of such crisis events. First, the evolving nexus between the literatures on critical infrastructure disruption and crisis will be discussed. Second, the notion of viewing critical infrastructure disruptions as crises will be presented in depth and illustrated with a variety of examples. Third, five key tasks of crisis management will be explained and discussed in terms of their application to the specific realm of critical infrastructure crises (cf. Baubion, 2013 ; Boin et al., 2017 ). The concluding section of the paper summarizes the results from the literature with regard to challenges and good practices for building resilience and preparing for critical infrastructure crisis management.
The Evolving Nexus of the Literatures on Critical Infrastructure and Crisis
Examination of the literature at the intersection of critical infrastructure and crisis (or crisis management) reveals both continuities with the past and some emerging areas of focus. The continuities include an emphasis on place and context, organizational factors, sector and industry, and hazard-specific attempts to assess or manage crises. That is, geographic place, industrial sector, and hazard type remain important through lines in the study of infrastructure crises, although some of these areas are also changing and have emerging areas of activity. On the other hand, there are also areas where the literature has expanded rapidly in recent years—areas such as recognition of the importance of social media (cf. Stern, 2017b ), attempts to operationalize and measure previously theoretical concepts, increasing prominence of cybersecurity, and emerging threats—that seem to be changing the nature and are the focus of much of this literature.
Place has always been key to studying infrastructure and crisis management—whether the level of analysis be the city, state, province, nation, or region. Case studies of infrastructure policy, regulation, and management (both in crises and beyond) have been a core part of this literature. Recent writing on infrastructure related crises maintains this history, with many pieces focused on incidents, responses, and management of such crises at the municipal ( Millington, 2018 ), subnational ( Zhang et al., 2020 ), national ( Grosse & Olausson, 2019 ), and cross-national or regional level ( Petersen et al., 2017a , 2017b ; Pursiainen, 2018 ). In addition to place, industrial sector has also long been an organizing factor for thinking about infrastructure, and that certainly has not changed. Recent literature on infrastructure crises covers such events in sectors such as water ( Breen & Markey, 2019 ), energy ( Monaghan & Walby, 2017 ), transportation ( Fuggini & Bolletta, 2020 ), information technology ( Herrera & Maennel, 2019 ), and maritime environments ( Blokus & Dziula, 2019 ). Finally, issues of management, decision-making, sense-making, and risk communication remain in the mainstream of recent infrastructure crisis literature. Topics such as consensus definitions ( Gallais & Filiol, 2017 ), interorganizational coordination ( Grosse & Olausson, 2019 ), public education and engagement ( Kitagawa et al., 2017 ; cf. Petersen et al., 2020 ), and assessing interdependencies ( Chou & Ongkowijoyo, 2019 ) are prominent.
However, there is some newer literature on or related to infrastructure crises that differs from (or more often, builds upon and extends) early research in significant ways. There are additions to the literature around place and infrastructure concerning cybersecurity and computerization, and there is a growing focus on urban infrastructure. Also, there is a growing literature designed to move from theoretical and conceptual description and assessment into operationalization, measurement, and evaluation using specific frameworks to create metrics, tools, and other systematic implementations. In addition, there is definitely an increased focus on emerging threats to infrastructure from largely naturally occuring hazards such as the COVID-19 pandemic; hybrid natural and anthropogenic hazards, including climate change; and politically and socioeconomically generated hazards, such as the inability to fund and maintain infrastructure projects.
Furthermore, there have been some interesting additions to this that have become more prominent and central in the literature in recent years. The movement of cybersecurity into the mainstream of thinking about hazards, and the increasing informatization of many aspects of life—through the Internet of Things (IoT) ( Simon, 2017 ), cloud computing ( Baker et al., 2019 ), “big data” ( Yang et al., 2017 ), and others—has clearly affected the literature around infrastructure crisis management ( Jennex, 2012 ). In addition, and related, the rapidly expanding literature around urban infrastructure and urban infrastructure crises ( Liu & Song, 2020 ; Monstadt & Schmidt, 2019 ) is partially based on this informatization—in concepts such as “smart cities” ( Soyata et al., 2019 ). It is also based on increased urbanization and recognition of infrastructure failures and threats ( Andrade et al., 2020 ) that are uniquely challenging in denser urban environments ( Fekete et al., 2017 ).
One way in which this literature has clearly changed is while there are still theoretical and conceptual pieces coming out in attempts to hone definitions and create agreed-upon frameworks, there has been a move toward operationalization ( Rød, 2020 ) and implementation of analytical frameworks. Increasingly, there are attempts to measure, assess, and evaluate hazards, crises, and decisions or decision-making using a variety of frameworks and tools. Although there are still attempts to apply new theories from outside the field ( Gharehyakheh et al., 2017 ), there are also many more attempts to distill or refine existing and applied theories into operational tools and modeling frameworks, with acronyms such as CIERA (Critical Infrastructure Element Resilience Assessment, Rehak et al., 2019 ) and CRISIS (Civil Restoration with Interdependent Social Infrastructure Systems, Loggins et al., 2019 ). This move from conceptual to operational, and in some cases from theoretical to empirical, suggests that this field not only continues to grow but is also maturing in some ways.
Finally, the field is engaging with a series of emerging and growing threats, some of which are actually novel, such as COVID-19—the most serious global pandemic in 100 years ( Barabadi et al., 2020 ). Also, some that have played a role in discussions of infrastructure crises for some time but are becoming increasingly stark or pressing, such as climate change ( Shakou et al., 2019 ). There are also unique challenges emerging that are not hazards in the traditional sense but organizational and sociotechnical challenges that parallel aging infrastructure and neglect of maintenance, only with new wrinkles such as austerity programs ( Petrova & Prodromidou, 2019 ) or inability to organize jurisdictional infrastructure spending ( de Gooyert, 2020 ; O’Brien et al., 2019 ).
Critical Infrastructure Disruption as a Crisis Leadership Challenge
If one is to speak meaningfully about disruptions of critical infrastructure as crises, it helpful to apply an explicit crisis definition. The casual and policy usage of the term crisis tends to vary widely across policy and academic contexts (and scholars) and can generate significant misunderstanding.
Definition of Crisis
In light of the dramatic consequences of the various forms of disruption of critical infrastructure noted above, one can safely argue that by definition, such disruption has the potential to spawn crises. 4 However, there may be differences in terms of the specifics of the direct and cascading effects. In fact, the adjective critical and the noun crisis are related; one standard meaning of critical refers to being at or on the verge of crisis. 5
From the perspective of policy decision-making, a crisis may be usefully defined in terms of three subjective criteria perceived by strategic leaders (as well as by those for whom they are responsible): threat, uncertainty , and urgency (cf. Hermann, 1963 ; Rosenthal et al., 1989 ; Stern, 2003 ). 6 These criteria are not only helpful in distinguishing crises from other types of situations but also provide a means for probing and preparing to act during them. The components of crisis will be introduced from a general perspective in the paragraphs below and then applied to the domain of critical infrastructure disruption in the following section.
First, crises are associated with threats to (and often potential opportunities to promote) the core values cherished by decision makers and/or their constituencies. These include, among others, human life, public health and welfare, democracy, civil liberties and rule of law, political autonomy, economic viability, and public confidence in leaders and institutions. Leaders must also be prepared to cope with conflicts among such values ( Farnham, 2000 ). The threat of terrorism, for example, entails potential conflicts between security considerations and civil liberties, as demonstrated by the post-9/11 debates on the Patriot Act and Guantanamo Bay, and, more recently, regarding electronic surveillance. Corresponding tensions can emerge with regard to potential public safety and health measures associated with other contingencies as well, such as quarantine restrictions in epidemics and mandatory evacuation orders in the face of hazards such as storms, wildfires, and toxic spills.
Second, crises are associated with high degrees of uncertainty regarding the nature of the threat (i.e., the known and unknown unknowns), the contours of an appropriate response, and the possible ramifications of various courses of action. For example, the causes and manner of contagion of sudden acute respiratory syndrome (SARS) were not known during the initial outbreak in 2003 ( Kleinman & Watson, 2006 ). It was difficult for Chinese and Canadian authorities to deal with the public health challenges—and the political, social, and economic consequences—of this disease in the absence of such knowledge ( Olsson et al., 2011 ). Some analysts distinguish between “familiar” and “novel” contingencies when it comes to crises. All else being equal, the more familiar the contingency (and the more it conforms to scenarios used prior to the crisis as a basis for planning, training, and exercising), the more likely it is that crisis managers will face moderate levels of uncertainty and be working in the domain of structured problem-solving. The more unexpected and novel the event, the greater the uncertainty and the more ill-structured the domain in which crisis managers must operate. Coping with such novel contingencies and the cascading shocks associated with them makes the already difficult challenges of crisis sense and decision-making even more demanding (cf. Baubion, 2013 ).
Third, crises are associated with a sense of urgency. Events are perceived as moving quickly, and there are fleeting windows of opportunity to influence their course. Effective, proactive intervention can minimize vulnerability (e.g., by getting citizens or mobile assets out of harm’s way before a storm hits) and help to prevent or mitigate the impact of a potential threat (e.g., disrupting a terror plot or isolating carriers of a highly infectious disease). Additional time pressure stems from the relentless pace of the 24-hour news cycle. Strategic decision makers and their organizations must cultivate the capacity to diagnose situations and formulate responses under severe time pressure. Thus, crises force decision makers to make some of the most consequential decisions in public life under extremely trying circumstances.
Applying the Crisis Definition to Critical Infrastructure Disruptions
The infrastructure systems of concern are labeled critical for a reason. These are systems vital to sustaining life, health, welfare, and functionality in highly developed societies, and disruptions to them tend to have a dramatic and cascading impact on core societal values. Furthermore, policy decisions regarding managing the consequences of disruption and priorities as well as resource allocations regarding system restoration involve (often competing) core values for societies and organizations. Political leaders and infrastructure operators face typical dilemmas relating to the protective shutdown of systems. Proactively shutting down systems in the face of threat or hazard warnings can help reduce exposure and avoid damage. For example, authorities shut down the U.S. civilian air transportation system completely in the wake of the 9/11 attacks ( Gordon et al., 2007 ). Similar preventive measures—with dramatic impacts on air transportation—and indirectly ground transportation—initially reduced the risk to air traffic stemming from volcanic dust during the eruption of an Icelandic volcano in 2010 ( Alexander, 2013 ; Brooker, 2010 ). However, it should be noted that such protective measures can cause severe disruption and post-decision controversy in and of themselves—especially if the potential threat triggering the measures does not materialize. Conversely, failure to take protective measures in the event of a major attack or destructive storm can expose government leaders and infrastructure operators to even more devastating liability and criticism. To take another example, for example, failure of the power grid can cause life and health-threatening consequences through impacts on food storage and preparation, hospital and critical care functionality, traffic management, temperature (heating or cooling of indoor spaces), and so on. Failure to maintain or rapidly restore service can negatively affect trust in government and in critical infrastructure operators alike ( Newlove et al., 2003 ). To take another example, recent cyberattacks have caused severe financial and reputational damage to individuals and institutions as indicated by the cyberattacks on the 2016 U.S. election, the Equifax data breach, the NotPetya data destruction attack, and breaches targeting major retailers. Furthermore, as so-called cyberphysical systems become more common and cyberweapons become more sophisticated, cyberattacks are liable to cause material, as well less tangible, forms of damage and pose severe threats to human life as well as information security. 7 The security firm Thales frames this change in the potential consequences of cyberattacks as a move from attacks on business information technology systems that result in mostly “financial and reputational risks” to attacks on industrial control systems that can also result in “safety and operational risks” ( Thales, 2013 ). Finally, it should be noted that many disruptions also raise significant issues of justice, fairness, and liability with regard to the distribution of responsibility for costs or other negative consequences stemming from the direct and indirect impacts of critical infrastructure disruption.
Uncertainty
As in many other realms of crisis, uncertainties abound when it comes to critical infrastructure crises. These uncertainties typically stem from a number of sources. These include uncertainty about the impacts and trajectories of acute natural hazards or potential threats originating from human adversaries. For example, despite advances in hurricane modeling and healthy competition between European and North American modelers, it remains difficult to predict hurricane tracks with sufficient timeliness and accuracy to enable efficient evacuation—as the experience of Florida with Hurricane Irma clearly demonstrated. In that case, areas thought to be in danger were proactively evacuated; however, the storm did not behave as predicted, and some of the evacuated persons ended up leaving areas less affected by Irma and going to areas hit harder by the hurricane. 8
Other forms of uncertainty stem from inadequate understanding of system interdependencies and vulnerabilities. These can result in unanticipated pathways to failure and consequences of disruption ( Roe & Schulman, 2016 ). Even where pathways are reasonably well understood, interdependence and linkages can cause vulnerabilities that are understood in general terms, but very difficult to mitigate in ways that are economic or feasible. In this case, the general problem is understood, but the uncertainty is largely focused on the type and scale of the initiating event’s impact on these systems. As Paul Edwards argues:
The effects of such failures can be magnified by interdependencies among infrastructures. For example, natural cataclysms can cripple one infrastructure—such as the emergency services system—by taking out others, such as the telephone system and the roadway network. Indeed, we depend so heavily on these infrastructures that the category of “natural disaster” really refers primarily to this relationship between natural events and infrastructures. ( Edwards, 2003 , pp. 193–194)
Further uncertainties stem from difficulties in predicting and coordinating responses of citizens and various nongovernmental actors to warnings, safety recommendations, and protective orders. Will the issuance of an evacuation order lead to an orderly exodus from an urban area or prolonged gridlock that will cause an additional disruption of the transportation system and increase citizen exposure to a threat or hazard? For example, Mayor Sylvester Turner of Houston chose not to emphasize proactive evacuation as a civil protection strategy for Hurricane Harvey due to concerns over these types of uncertainties. 9
Similarly, another type of potential uncertainty has to do with how much cooperation and solidarity to expect from citizens, neighbors, or partners (cf. Almklov et al., 2012 ; cf. Grönvall, 2001 )? Will a recommendation to households to make sure to have essential supplies lead to sensible preparations or exaggerated hoarding behaviors that disrupt supply chains? Will citizens respond to calls to conserve water or energy to an extent sufficient to mitigate an escalating crisis? How will neighboring jurisdictions (municipalities, provinces or states, countries) respond to calls for assistance? Will they live up to obligations to provide mutual aid, assistance, and potentially scarce critical resources if they are facing a potential or actual threat—or intense domestic political pressure—as well?
Time Pressure
Time pressure manifests in a number of ways in critical infrastructure crisis management, and it poses particular challenges with regard to warning and response. First of all, there is often a “window of opportunity” for proactive protection measures such as a grid or transportation system shutdown of the kind discussed previously. As a threat or hazard is becoming more imminent, it may be possible to reduce exposure in various ways. For example, shutting down or reducing load on a power grid or satellite-based communications or navigation systems can reduce vulnerability to damage caused by extreme solar storms (cf. Odenwald & Green, 2008 ). Similarly, heightening security or shutting down air or rail traffic in response in response to an acute terrorist threat (9/11 or the London Bombings of 2005 ) or hazard (hurricane force winds or volcanic ash) can help protect assets and/or users of the infrastructure systems in question. However, such opportunities tend to be time-sensitive and may be fleeting. Other systems (such as some pharmaceuticals production and supply chains, nuclear power, and some chemical storage sites [e.g., Arkema plant; see above]) may require continuous access to refrigeration, power, fuel, or cooling water to maintain safe operations, creating intense time pressure to restore functionality should disruptions occur. Should deviations from normal functionality take place, there may be limited time to intervene in order to prevent accidents or other forms of cascading disruptions from occurring. Similarly, there may be limited time to attempt to influence public behaviors in ways that will protect systems or prevent disruption to critical infrastructures and supply chains (cf. Holeguin-Veras et al., 2012 ). Efforts to get the public to conserve water (e.g., during the California droughts) or power to protect vulnerable power transmission systems (e.g., Auckland, Outer Banks) are likely to involve considerable urgency. Finally, inquiries, criticism, and pressure from media (traditional and social), advocacy groups, the opposition, and competitor groups can create additional forms of time pressure and add additional time-sensitive tasks to the burdens carried by crisis managers.
Clearly, contingencies involving critical infrastructure disruptions—whether as a triggering event or (potential) consequence of natural hazards, large-scale accidents, or deliberate attacks—meet the crisis criteria proposed previously. Readers should note that the following criteria can be easily turned into diagnostic questions that can help crisis managers make sense of events involving potential or actual critical infrastructure disruption (cf. OECD, 2015 ; Stern, 2009 ):
What values are at stake in this situation?
What are the critical uncertainties and what can be done to reduce them?
How much time is available for deliberation and decision-making?
Crisis Management Tasks and Application to Critical Infrastructure Crisis
Core crisis management tasks.
Several decades of intensive empirical research on crisis management shows that leaders face recurring challenges when confronted with (the prospect of) community (or organizational/national/international) crises ( Boin et al., 2017 ). 10 These are:
sense-making
decision-making
meaning-making
ending and accounting
learning and changing
These tasks are germane to leaders and other crisis managers across sectors and are central not only to effective crisis leadership in a particular incident but also to creating better preconditions for future incidents and resilient adaptation to changing environmental conditions over the longer term. Hannah et al. (2009) , like Leonard and Howitt (2012) , suggest that different forms of leadership may be needed in different phases of a disaster or crisis. Helsloot et al. (2012) , with their focus on “mega-crises,” describe leadership challenges across crises of differing size and scope. The following conceptualization identifies crisis leadership tasks likely to arise during a variety of extreme events.
Sense-making in crisis refers to the challenging task of developing an adequate interpretation of what are often complex, dynamic, and ambiguous situations (cf. Stern, 2015 ; Weick, 1988 , 1993 ). This entails developing not only a picture of what is happening but also an understanding of the implications of the situation from one’s own vantage point and that of other salient stakeholders. As Alberts and Hayes (2003) put it: “Sense-making is much more than sharing information and identifying patterns. It goes beyond what is happening and what may happen to what can be done about it” (p. 102).
Making sense of critical infrastructure crises is a challenging task. As this discussion has shown, uncertainties, ambiguities regarding threats, hazards, latent vulnerabilities as well as complex interdependencies within and among critical infrastructure systems make sense-making extremely difficult. Even when warnings are forthcoming—for example, with regard to natural disasters such as hurricanes and flooding where monitoring and detection systems have improved significantly in recent years in many parts of the world—it is often very difficult to predict with confidence how particular critical infrastructures will be affected or what the cascading effects of disruptions might be. This task is even more difficult with relation to novel, relatively unanticipated crises in which information and data-sharing networks and expert communities of practice may be underdeveloped and much of the sense-making work must take place on a relatively improvised basis. This was the case, for example, to a considerable extent during the volcanic ash cloud crisis sparked by volcanic activity in Iceland in 2010 bringing commercial air traffic to a halt over much of Europe ( Parker, 2014 ). In such cases, difficult scientific and technical assessments with regard to “exotic” issues must be produced and communicated to governmental and other key decision-makers under crisis conditions. In many countries this still occurs largely on an ad hoc basis, although others such as the United Kingdom have developed (or are in the process of developing) Scientific Advisory Groups for Emergencies [SAGE]. 11
A first step in making sense of a critical infrastructure crises is to identify key public, private, and nonprofit actors and stakeholders and gather information via various forms of (social and technical) networks associated with or connecting across critical infrastructure sectors. Many highly developed countries have or are in the process of developing strategies and collaborative fora to improve critical infrastructure protection and resilience. Such efforts, hopefully undertaken well before the onset of a crisis can improve the flow of information, contribute to improved situational awareness, and facilitate “heedful interrelating” and other forms of crisis management cooperation among diverse sets of actors involved in governing, operating, or using critical infrastructures. 12 Making sense of critical infrastructure crises is, of course, facilitated by deeper and more dynamic understanding of systems and cross-system interdependencies. As a result, efforts to improve technologies for mapping, modeling, simulation, and visualization of such systems can contribute not only to system design improvements to improve resilience and reliability but also to capacity for crisis management and rapid restoration. 13 This issue will be discussed further in the final section of this paper, Preparing for Critical Infrastructure Crisis Management.
Another promising development—to the extent that communications and mobile data networks remain available—for improving situational awareness in critical infrastructure crisis is citizen crowdsourcing. Armed with smartphones and web and geographic information system (GIS)-based platforms for aggregating, analyzing, integrating and transposing data, citizens as well as various forms of organized official or unofficial responders can act as sensors ( Akhgar et al., 2017 ; Stern, 2017b ).
Decision-making refers to the fact that crises tend to be experienced by leaders (and those who follow them) as a series of “what do we do now” problems triggered by the flow of events. These decision occasions emerge simultaneously or in succession over the course of the crisis ( Newlove et al., 2003 ; Stern, 1999 ; Stern et al., 2014 ). Coping with critical infrastructure disruptions and managing the restoration process tends to require an interdependent series of crucial decisions and to be taken in a timely fashion under very difficult conditions by a variety of public, private, and nonprofit sector actors and stakeholders. Of note, governance arrangements may vary significantly among highly developed countries regarding the extent of public control, regulation, and ownership of critical infrastructures.
It is important to keep in mind that decisions in critical infrastructure crises will, by definition, be taken under highly stressful conditions of considerably uncertainty, time pressure, and threat to core societal and organizational values, as noted above. Furthermore, conflicts among values are to be expected, contributing further to the decisional stress load facing decision-makers (cf. Lebow & Stein, 2019 ).
It may be helpful to consider typical examples of the kinds of decisions that are likely to arise in the context of a critical infrastructure crisis. Taking a threat/disruption to electric power infrastructure as an example, the following are just some of the very difficult crisis decisions that may be required ( Newlove et al., 2003 ; Nye, 2010 ; Parker et al., 2009 ):
Decisions to issue warnings regarding threats, hazards, and possible power grid disruptions and/or their consequences
Decisions to order protective shutdowns to protect system components (e.g., power generation or transmission) or prevent unsafe or unduly risky operations under conditions of acute threat or mounting natural hazards
Decisions to request or order mandatory power conservation to prevent further degradation of the power system
Decisions regarding how to allocate drastically reduced supplies of power and scarce repair capabilities and resources
Decisions regarding requesting, providing or accepting mutual aid (e.g., in the form of components or repair crews from other regions of the country and/or from other countries)
Decisions regarding provision of financial resources for response and recovery, and/or accepting financial or moral responsibility for the direct and indirect costs and consequences associated with outages and restoration
Decisions regarding public health and safety measures (e.g., food safety and pharmaceuticals interventions for compromised refrigeration, decisions regarding hospital continuity of service) necessitated by power outages
Decisions regarding civil protection measures—such as evacuation orders or instruction to shelter-in-place, curfews—for populations potentially endangered by consequences of power outages and supply chain disruptions and/or the hazards and threats that triggered them
Decisions regarding measures for restoration and recovery, some of which may conflict with normal rules, practices and procedures for building planning and permission, environmental protection, and so on
Thus, decision-making in critical infrastructure crises, as in other types of crisis events, is extremely complex, difficult and demanding.
Meaning-making (and crisis communication) refers to the fact that leaders—from public, private, and nonprofit sectors alike—must attend not only to the operational crisis communications challenges associated with a contingency, but also to the ways in which various stakeholders and constituencies perceive and understand them. Because of the emotional charge associated with disruptive events, followers look to leaders to help them to understand the meaning of what has happened and place it a broader perspective. By their words and deeds, leaders can convey images of competence, control, stability, sincerity, decisiveness, and vision—or their polar opposites.
Critical infrastructure crises can pose unique challenges with regard to crisis communication in general and meaning-making in particular. First of all, information and communications technology—and critical infrastructures which support them—may be directly affected by the impact of accidents, natural hazards or attacks associated with the crisis. Extreme earth (windstorms, floods) and space weather (e.g., solar storms) events as well as physical and cyberattacks can disrupt the capacity to communicate via both wired and wireless communications networks for shorter or longer periods. In addition, many types of events (again including both deliberate attacks, major accidents, and natural hazard–based events) may be associated with increased citizen and responder communications traffic to the extent that vulnerable communications networks may fail or have severe degradations regarding access, reliability, or quality of communications. As a result, crisis managers may have difficulty in sending and receiving messages and sharing data when ability to do so matters most.
For example, during the prolonged summertime power outage in Auckland, New Zealand in 1998 , the normal press briefing facilities (among other key functions and coordination centers for city government) were unuseable because of high temperatures and lack of functioning elevators. As a result, Mayor Les Mills ended up conducting much of his business and media interviews from his car and mobile phone from various locations around the city, which complicated the coordination of operations and messaging. The power company, Mercury Energy, which was responsible for the outage, was kind enough to offer the mayor the use of their press briefing facilities, which had functioning power and communications. Unfortunately for the mayor, this also entailed giving his briefings from a podium boldly displaying the logo of what was at the time a very unpopular critical infrastructure operator. This served to symbolically associate the mayor with the power company at a time when a degree of critical distance would have served him better politically. It should be noted that he lost the next election, in part due to what some regarded as a subpar performance in critical infrastructure crisis management ( Newlove et al., 2003 ).
Hurricane Maria in Puerto Rico in the fall of 2017 is another excellent example. Vulnerable communications and electrical power networks, already battered by Hurricane Irma, were devastated by Hurricane Maria. As a result, much of the response and early recovery effort took place under particularly challenging conditions and large portions of the stricken island remained without power and electricity as not only days but weeks and months passed. Modular cellular technologies (so-called cells on wheels, or COWS) were helpful in restoring communications in certain urban or other particularly sensitive areas but were insufficient to restore communications capacity for the bulk of the island. 14 Degraded communications capacity can limit the ability of communities to self-organize and coordinate citizen-based efforts to respond to and recover from disasters using social media and smart communications technologies—an increasingly important dimension of resilience in large-scale events ( Akhgar et al., 2017 ; Stern, 2017b ; Stern, 2017a ).
Hurricane Maria also contains good examples of the ways that leaders at various levels of government engage in competitive attempts to demonstrate empathy and engagement as well as endeavoring to shape the narratives and public perceptions of the event. While President Trump and acting Secretary of Homeland Security Elaine Dukes suggested that the response to Maria in Puerto Rico was effective and a “good news story,” Carmen Yulin Cruz, the Mayor of San Juan, appeared on CNN wearing a black T-shirt with the text “We are dying,” creating vivid images that went viral via social and traditional media far beyond the island. Similarly, the tone set by President Trump’s combative approach to crisis communication—which included sharp criticism via Twitter of the leadership of Mayor Cruz and allegations that the Puerto Rican locals were not doing their part with regard to response and recovery—proved highly controversial. 15
Ending and Accounting refers to the nontrivial task of finding the appropriate timing and means to end the crisis, manage accountability processes, and return to normalcy. Furthermore, attempting to end a crisis prematurely can endanger or alienate constituencies who may still be in harm’s way, traumatized, or otherwise continue to be politically or emotionally invested in the crisis. Crises may be particularly difficult to terminate if the operational challenges lead to a so-called crisis after the crisis in which serious recriminations—resulting in losses of trust and legitimacy—are launched against those who failed to prevent, respond to, or recover effectively from a negative event.
Critical infrastructure crises, like other forms of crisis, may have different trajectories and different combinations of operational and legitimacy dimensions. Some are “sudden onset” in which a dramatic event such as an earthquake or hurricane causes dramatic damage. Others may demonstrate a “creeping” quality whereby a threat slowly manifests or the functionality of a critical infrastructure slowly degrades for a prolonged period before attracting notice. The water crises that have affected many cities around the world—from places as diverse as Mexico City; Flint, Michigan; and Hoosick Falls (New York)—are good examples of vulnerabilities mounting as a result of environmental change; rapid population growth and development; industrial pollution; or unsafe properties of aging infrastructure installations (use of lead pipe in water distribution systems); and so on. 16
Similarly, some critical infrastructure crises demonstrate rapid and effective restoration of the pre-crisis status quo, whereas others may exhibit prolonged disruptions of functionality. This issue of differing time frames and trajectories is even more troubling when combined with the fact that infrastructure risk experts claim that “Many security risk decisions do not account for the life cycle of a system or an infrastructure” Assante, 2009 :4), That is, there is a disconnect between short-term decision making—and the incentives surrounding it—and the very long terms for which many infrastructures are expected to remain operational. Logically, this could both make crises more likely but also suggests that crisis decision-making might be likely to be short-sighted. Even in shorter, more clear-cut cases of infrastructure crisis, public evaluation of leadership and authorities can be mixed or contradictory ( Helsloot & Beerens, 2009 ), in long-term and complex situations such as these, the effect is likely to be similar.
Tied to the trajectories, some disruptions and their responses may morph into legitimacy crises after the initial infrastructure crisis (cf. Boin et al., 2017 ; Fischbacher-Smith, 2006 ). When it comes to critical infrastructure crises, such legitimacy crises may focus on questions such as:
Why were vulnerable and/or potentially dangerous critical infrastructure systems or components allowed to be used or to remain in service?
Why were safety margins and measures not more robust?
Why were safer and/or more reliable alternatives to the failed or vulnerable technologies not used?
Why were “known” risks and vulnerabilities not addressed more proactively and promptly before and/or during the crisis?
Why were leaders not more focused on the crisis issue and why did they not provide stronger, more empathetic, and/or more effective leadership?
Why was the response not better coordinated, better resourced, more proactive, more effective, more fair, and so on?
Why was the restoration and early recovery not faster, cheaper, more effective, and more complete?
Why were some groups in society advantaged or disadvantaged (prioritized or neglected) in terms of response and recovery efforts?
The reader should note that these issues are likely to arise in multiple accountability fora: parliamentary bodies, professional or industry associations or governance bodies, regulatory agencies, courts, and the media. Furthermore, crisis experiences and their associated accountability processes may profoundly challenge and in some instances destabilize established practices and patterns of governance (including regulation and divisions of authority and responsibility across sectors and levels of government) with regard to managing critical infrastructure sectors. When critical infrastructure operators and industries are perceived to have been—or determined in a court of law to have been—negligent, strong pressures for change and significant legal and financial liability for operators and other private sector actors may emerge. This can take the form of proposals for increased regulation, incentivizing safety and resilience, heightened operator responsibility for damages, or even withdrawal of the “permissive consensus” that allows potentially hazardous activities such as nuclear power or process industries involving potentially dangerous chemicals to continue. The Fukushima nuclear accident stemming from the great Tohuko earthquake and tsunami is a good example, with profound repercussions for the nuclear power industry even in countries on the other side of the world such as Germany ( Bernardi et al., 2018 ).
The highly complex systems of critical infrastructure governance entailing various forms and degrees of regulation and legislation, diverse patterns of ownership, different varieties of public-private partnership in place in many countries have profound implications for accountability with regard to critical infrastructure disruption/restoration and crisis management (cf. Dunn-Cavelty & Suter, 2009 ). Awareness of and outcomes from previous accountability processes should inform preparedness, response, recovery, and postcrisis efforts toward learning and reform (which will be discussed next).
Learning and changing requires an active, critical and reflective process that recreates, analyzes, and evaluates key processes, tactics, techniques, and procedures in order to enhance performance, safety, capability, and so on. The learning process has just begun when a so-called lessons learned document has been produced. To bring the learning process to fruition, change in management/implementation must take place in a fashion that leaves the organization with improved prospects for future success ( Boin et al., 2017 ; Deverell & Olsson, 2009 ; Stern, 2015 ; Stern et al., 2014 ). Note that political and organizational cultural obstacles and various forms of disincentives often prevent effective learning and change from taking place ( Stern, 1997 ).
Critical infrastructure disruptions—like other forms of crisis—provide unique chances for learning and opportunities for change. Latent or unrecognized vulnerabilities and interdependencies manifest themselves in dramatic fashion, providing opportunities for redesign and reform of technical systems, institutional frameworks, tactics, techniques, procedures, and so on. The abrupt manifestation of urgent and difficult problems can stimulate creative improvisation as well as new forms and constellations of collaboration and innovation. However, taking advantage of these opportunities requires reflection, inquiry, leadership, and resources to identify adaptive lessons (cf. Heifetz & Laurie, 2001 ) and translate them into more resilient, reliable effective and legitimately functioning systems for critical infrastructure and crisis management.
Hurricane Sandy serves as a vivid example. The second-largest Atlantic storm on record, Sandy affected the East Coast of the United States from Florida to Maine, as well as states as far inland as West Virginia, Ohio, and Indiana. The storm made landfall in southern New Jersey on October 29, 2012, battering the densely populated New York and New Jersey region with heavy rains, strong winds, and record storm surges. The storm’s effects were extensive, leaving more than 8.5 million customers without power, causing widespread flooding throughout the region, and contributing to acute fuel shortages in parts of New York and New Jersey. In the United Statesl the storm damaged or destroyed hundreds of thousands of homes, caused tens of billions of dollars in damages, and killed at least 162 people. 17
In addition, Hurricane Sandy caused severe damage and disruption to multiple critical infrastructure systems in New York and New Jersey, including transportation, 18 energy (electric power and fuel distribution), water provision, 19 and healthcare (e.g. hospitals and nursing homes). Numerous points of vulnerability and challenging problems emerged ranging from the vulnerability of the subway system and the cable installations servicing it to flood damage, cascading interdependencies between electric power and various nodes in the fuel distribution network, and fragility of hospital power backup systems in the face of massive storm surge and flooding (e.g., the evacuation of N.Y.U. Langone Medical Cente). 20 Following Hurricane Sandy, there have been a large number of after-action reports, inquiries, and research studies about various aspects of the crisis, response, and recovery associated with it. Many of these efforts have focused on or significantly involved critical infrastructure functions and actors, and a variety of important lessons have been learned and changes made. 21
The experience of the Atlantic hurricanes of 2017 has begun to be systematically processed via a postcrisis multidimensional after action and research effort of the kind that took place for Hurricane Sandy and some previous major Atlantic storms (e.g., Katrina). There are significant indications that important lessons were learned from Sandy and previous cases that informed the proactive crisis management by many governmental actors and critical infrastructure operators regarding response and restoration efforts tied to Hurricanes Harvey and Irma ( Federal Emergency Management Agency [FEMA], 2018 ). However, the much more problematic response and delayed restoration of critical infrastructure services (e.g., power, fuel, telecommunications, water) to Hurricane Maria in the U.S. territory of Puerto Rico is both instructive and troubling. Clearly, resilience and preparedness for critical infrastructure crisis management can develop in an uneven fashion even in highly developed countries, leaving pockets of particular resilience and particular vulnerability. Contextual factors such as geographic centrality/remoteness, regional economic strength, and robustness or fragility of particular infrastructures, functionality of local governance, coincidence with other simultaneous or near simultaneous events, and not least political will can all affect response and recovery. The increased frequency and severity of events not only poses challenges to societal and organizational resilience but also to the ability of organizations to focus on and extract the lessons from individual events. Shifting to “seasonal” rather than individual event-based after-action reviews (as FEMA did regarding the eventful 2017 hurricane season) is understandable but can result in missed learning opportunities associated with particular events ( FEMA, 2018 ).
Although effective production and adaptive implementation of “lessons learned” is very challenging and requires significant investment of time and resources, failure to learn from experience is a recipe for stagnation and perpetuation of vulnerability. This may be extremely costly in humanitarian, political, and financial terms for the key actors involved in the governance and operation of critical infrastructure systems ( Boin & McConnell, 2007 ; Boin et al., 2017 ).
Cybersecurity as a Driver of Infrastructure Risk and a Complicating Factor in Infrastructure Crisis Management
Many of the components of crisis management are exacerbated by the move toward infrastructure that is informatized and automated. Sense-making and decision-making are both made more difficult, because they require the incorporation of new sets of experts and technical advisors. This is true because computers and related technologies are—despite their ubiquity— are still seen as “exotic” by some decision makers. Technical advisors will almost never eliminate uncertainty, one of the defining characteristics of crises; rather, the goal is to have such specialists help limit, reduce, or manage uncertainty—and thus improve decision-making and outcomes.
Another challenge to sense-making is not merely that vulnerabilities are latent, but that each technical upgrade potentially introduces new vulnerabilities, although they often remove old vulnerabilities as well. This changing landscape of technical vulnerability shifts much more rapidly than the vulnerability landscape of large physical infrastructures that were manually controlled. This problem, long struggled with in cybersecurity, is a result of increased complexity and processes that have tended to prize novelty or convenience over security. As Assante notes “incorporating new technology can introduce vulnerabilities” ( Assante, 2009 :4). However, maintaining the ability to manually control such infrastructure can play a major role in being able to mitigate the novel infrastructure crisis. This was discovered by Ukrainian power workers coping with several cyberattack-induced blackouts that would have been far worse without the ability to manually restart power systems without the computerized controls ( Greenberg, 2017 ).
Meaning-making becomes more complicated as complex information—from digital forensics to the impacts of data breaches—must be communicated to communities and stakeholders who lack the technical background to evaluate the evidence. From breaches such as those at Equifax and the Office of Personnel Management (OPM), as well as Russian attempts at interference in the 2016 U. S. election, increasingly crisis managers must discuss complex technical matters (as well as the complex sociopolitical matters that have always defined crises) in ways that risk oversimplifying or misleading.
A particularly dangerous version of this challenge comes when this meaning-making and risk communication is undermined or countered by those deemed credible. Following the Sony Pictures Entertainment hack, which the United States subsequently attributed to North Korea, there was a broad dispute in the media in which cybersecurity professionals argued that the U.S. government had not released sufficient evidence to justify their assertions about attribution. Similar debates followed public assertions attributing election interference to Russia. Moving forward, this expectation that crisis leaders will conduct—and perhaps even demonstrate or share—highly technical analysis related to forensics and attribution may well constrain crisis decision-making and messaging.
The escalation of the consequences of cyberattacks from “financial and reputational risks” to “safety and operational risks” ( Thales, 2013 ) described earlier will only grow more serious with the IoT, smart cities, and the growing ubiquity of embedded computing in cyberphysical systems ranging from pacemakers to automobiles to traffic lights. These vulnerabilties will spawn novel forms of infrastructure crises moving forward. Some have already occurred, such as ransomware attacks on transportation infrastructure and hospitals. Others may eventually take place, such as ransomware attacks on prosthetic limbs and pacemakers or attacks on supply chains to manipulate financial markets. Furthermore, the increasing consequences of cybersecurity lapses will not only create novel crises but will also influence existing elements of crisis management. How easy is the “accounting” part of “ending and accounting” or the “learning” component of “learning and changing” when the malware that resulted in the incident erases logs and evidence of what happened and hinders documentation of crisis response efforts? How much public pressure—productive or counterproductive—will follow the first documented cyberhijacking of an automated driverless vehicle that results in the death of a passenger? Understanding the future of infrastructure risk, and the future of infrastructure crises, will increasingly require understanding cybersecurity. Both infrastructure managers and crisis managers will increasingly be expected to operate knowledgably and effectively in this environment.
Preparing for Critical Infrastructure Crisis Management
Having explored the five crisis management tasks discussed in the previous section, it is important to note that a key additional imperative for leaders is the broader task of preparing for crisis management ( Boin et al., 2017 , chapter 7; Stern, 2013 ).
Preparing refers to the task of creating preconditions and dispositions that facilitate collaborative effort as well as effective and legitimate intervention when crises occur as well as during their aftermath. Elements of preparing include activities such as organizing, networking, planning, training, and exercising. This generally entails attempting to identify key players and roles likely to be required for effective societal, community, or organizational response and making sure that each role-player is capable of enacting that role skillfully and in a fashion conducive to not just particularistic but also collective success. Leadership with regard to this task has a key motivational component—preparedness requires investments in time and resources that compete with other priorities in times of “peace.” Ironically, when a crisis is imminent, such as when meteorological experts predict that a hurricane is on its way, motivation tends to be high. However, in such acute preparedness efforts in the face of an escalating event, although the will to prepare may be high, difficult dispositions must be made under crisis-like conditions of uncertainty, time pressure, and resource scarcity.
On the basis of the literature reviewed previously, it is possible to identify a number of target areas for improvement and good practices with regard to improving preparedness and capacity for critical infrastructure crisis management and rapid restoration in the wake of critical infrastructure disruptions in highly developed countries. These include:
Improving international cooperation and joint management of critical infrastructure systems
Building trust and relationships in support of crisis management across the public-private-nonprofit sector divide, and among disconnected organizations in each sector
Understanding, improving, and planning relationships with internal and external sources of technical “reach-back” and expertise
Improving knowledge and understanding of critical infrastructure systems through mapping, modeling, and simulation
Reinvigorating critical incident response and recovery planning
Training and exercises involving key public, private, and nonprofit actors at both strategic and operational levels
Improving accountability and facilitating organizational and interorganizational learning, to foster more effective investment in infrastructure planning and maintenance
Improving International Cooperation and Collaborative Response and Recovery of Critical Infrastructure System s
As seen previously, contemporary critical infrastructure systems transcend national boundaries in various ways. Disruptions may originate in physical events occurring in or cyberattacks launched from on one or more countries but end up affecting many others. Many natural hazard scenarios—such as extreme terrestrial and space weather events—can have an impact on critical infrastructures across national boundaries and influence global supply chains. Preventing attacks and limiting the damage of disruptions stemming from natural, man-made, or mixed events often requires rapid, even real-time, exchange of intelligence and system status information as well as expert knowledge. In addition, effective coordination of protective, response, and recovery measures is essential to prevent further cascades of disruption and damage and facilitate rapid restoration (e.g., via effective use of scarce repair capabilities and component resources across countries). This is true not only of power and other energy systems, but also of transportation, and telecommunications.
Building Trust and Relationships in Support of Crisis Management Across the Public and Private Nonprofit Divide
A broad set of public, private, and nonprofit players share authority, responsibility, capabilities, information, knowledge, and resources needed to cope with severe critical infrastructure disruptions and their cascading effects. Although these actors are diverse in many respects, they have significant common interests in mitigating disruptions and promoting rapid restoration of critical infrastructures. Whole society/community approaches increasingly in emergency management and resilience-building are highly relevant to improving capacity to cope with critical infrastructure failure as well. Creating opportunities, incentives, and fora for these individuals and organizations to familiarize themselves and cooperate with each other—before, during, and after crisis events—is extremely valuable. Governments are actively supporting this process in many of the most developed countries (e.g., United States, United Kingdom, Sweden, Switzerland 22 ) via resilience fora, critical infrastructure sector groupings, and other equivalent efforts, although much more can be done.
Understanding, Improving, and Planning Relationships with Internal and External Sources of Technical “Reachback” and Expertise
Although the critical importance of access to technical expertise is perhaps most obvious in the relatively arcane cybersecurity field, parallel needs for technical expertise arise in many other types of infrastructure-related crises. During the Deepwater Horizon spill, there were significant challenges of accessing, coordinating, and integrating different types of expertise into the crisis management effort. ( Birkland & DeYoung, 2011 ; cf. Koraeus & Stern, 2013 ). Public and private sector crisis managers were dependent upon a small community of experts in offshore drilling and underwater geology from many organizations and sectors ( Busch & Givens, 2012 ). This is, of course, just one among many other types of expertise urgently needed to deal with the cascading environmental, social, and economic consequences of the oil spill.
Similar mobilization of expertise is necessary in many types of infrastructure crises. In cyberattacks or large-scale cyberdisruptions, owners and operators of infrastructure need to be able to assemble rapidly highly qualified teams of cybersecurity, digital forensics, or industrial control system experts. This expertise need not be resident in the owners’ or operators’ company or agency. There certainly could be internal resources such as the personnel at the Security Operations Center (SOC) or incident response team, but would also include contractors and security vendors, industry or sectorwide resources such as Information Sharing and Analysis Organizations (ISAOs) and Information Sharing and Analysis Centers (ISACs), and even regulators and law enforcement. Building the “right” team at the right time is key to sense-making, decision-making, and meaning-making in critical infrastructure crises.
Improving Knowledge and Understanding of Critical Infrastructure Systems Through Mapping, Modeling, and Simulation
As demonstrated in the preceding sections, critical infrastructure systems are highly complex and dynamic. Such systems and the environments in which they operate evolve and change on an ongoing basis. Human ability to understand and predict outcomes with regard to disruptions and protective interventions with regard to such systems is limited (cf. Perrow, 1984 ; Roe & Schulman, 2016 ) and must be leveraged by investments in efforts to map, monitor, and develop deeper understanding of them. Significant advancements of methodology and technology may be harnessed to improve mapping (both geographic and functional) to identify pathways to and from failure and toward rapid restoration. Empirically based modeling, simulation, and visualization tools can be used to improve reliability and resilience more generally as well as to facilitate effective response and rapid restoration to critical infrastructure disruptions. 23
Reinvigorating Critical Incident Response and Recovery Planning
Emergency planning in many countries has been criticized for a tendency toward unrealistic planning assumptions regarding environmental and system vulnerabilities, magnitude and duration of disruptions, and availability of capabilities and resources ( Clarke, 1999 ; Eriksson & McConnell, 2011 ) as well as for neglecting key actors, stakeholders, and particularly vulnerable populations. Conversely, improved planning for critical infrastructure disruptions will:
involve a broader set of public, private, and nonprofit actors and stakeholders.
build on state-of-the-art knowledge of systems, hazards, and interventions informed by mapping, historical experience base with regard to critical infrastructure crises, and empirically informed and rigorous modeling and simulation methods and technology.
include explicit decision criteria and intervention protocols for proactive system protective measures and planning for the operational and political consequences associated with such measures.
consider the impact of interdependencies among critical infrastructure systems ( Roe &Schulman, 2016 ), cascading consequences, and capability degradation stemming from the event itself or protective shutdown measures with regard to one or more critical infrastructure systems.
Think bigger and emphase “bad” or “worst” case scenarios—the so-called maximum of maximums as it has been labeled in U.S. FEMA doctrine—in order to reveal limitations and lay a foundation for adaptive behavior in the face of catastrophic events.
Depart from conservative assumptions regarding available resources for response and recovery efforts.
Plan explicitly and seek to secure preferential access to resources and capabilities in support of rapid restoration of service.
Training and Exercises Involving Key Actors—at Both Strategic and Operational Levels—From Government, Critical Infrastructure Operators, and Other Key Private and Nonprofit Sector Stakeholders
Government and critical infrastructure operator leaders should take steps to make sure that they and their team members, other key subordinates, and counterparts from essential partners are educated, trained, and exercised in preparation for critical infrastructure crisis management. 24 When facing major crises, partnership between strategic leaders and “professionals” inside and outside of government is essential. This means that political leaders who are not “professionals” must be educated as to the nature of critical infrastructure crisis management, informed of what is required of them in scenarios and contingencies involving (the risk of) significant disruption to critical infrastructure systems, familiarized with relevant crisis planning and organization, and equipped to engage effectively in meaningful communicative interaction with others inside and outside of their organizations. Individual and collective crisis management skills are best acquired and honed through hands-on practice in simulated as well as real-world incidents and crises. There are a wide variety of powerful instructional designs and techniques (both traditional and technology enhanced) suitable for critical infrastructure crisis management training and exercises. 25 Instructional designs and techniques should be consciously chosen and explicitly adapted to the goals and purposes of a given training or exercise for maximum positive impact.
Improving Accountability and Enabling Organizational and Interorganizational Learning
Critical infrastructure failures raise significant issues of political, legal, and financial accountability. Regulatory regimes, divisions of labor, and responsibility continue to vary greatly across countries and sectors and ambiguities and gaps persist, contributing to suboptimal resilience and suboptimal preparedness for response and rapid restoration. As noted previously, public, private, and nonprofit sector actors participating in the governance and operation of critical infrastructure systems are subject to multiple forms of accountability and must be prepared to answer questions not only in Congress (or Parliament), but also literally in court and in the metaphorical “courts” of (social) media and public opinion.
Disruption of critical infrastructure systems, by definition, may be costly in lives and treasure, as well as in organizational (and in many cases personal) trust and legitimacy. As a result, it is imperative to learn the lessons of experience. Doing so effectively requires fostering a culture of safety and resilience in which information is shared and performance before, during, and after crises that are subjected to benchmarking and critical scrutiny. Experts should resist temptations to withhold “embarrassing” information and whitewash subpar performances in favor of systematic and methodologically informed inquiry and vigorous and forward-looking implementation of measures to address preparedness gaps and shortcomings.
There is good reason to believe that managing infrastructure crises effectively could be improved by a better integration of the scholarship and practice of crisis management and the parallel scholarship and practice of infrastructure management. Achieving this integration will require several things. First and foremost, it requires viewing critical infrastructure disruptions—whether resulting from natural disaster, industrial accident, or adversarial action—as crises that threaten core values and entail decision-making and management under conditions of uncertainty and urgency. If critical infrastructure disruptions can be fruitfully viewed as crises—then good practices from the crisis management literature with regard to sense-making , decision-making , meaning-making , ending and accounting , and learning and changing ( Boin et al., 2017 ; Stern, 2013 ) promise to be helpful in improving organizational and societal critical infrastructure resilience as well. Finally, if infrastructure disruptions are indeed understood as crises, and models as well as experience-based lessons from crisis management are brought to bear in seeking to understand better and meet such challenges, then both practitioners and scholars of crisis management and infrastructure management will be better equipped to learn from each other ( Trainor et al., 2018 ).
- Akhgar, B. , Staniforth, A. , & Waddington, D. (Eds.). (2017). Application of social media in crisis management . Springer.
- Alberts, D. S. , & Hayes, R. E. (2003). Power to the edge . Command and Control Research Program.
- Alexander, D. (2013). Volcanic ash in the atmosphere and risks for civil aviation: A study in European crisis management. International Journal of Disaster Risk Science , 4 (1), 9–19.
- Almklov, P. , Antonsen, S. , & Fenstad, J. (2012). Organizational challenges regarding risk management in critical infrastructures. In P. Hokstad , I. B. Utne , & J. Vatn (Eds.), Risk and interdependencies in critical infrastructures: A guideline for analysis (pp. 211–225). Springer London.
- Andrade, E. R. , Reis, A. L. Q. , Alves, D. F. , Alves, I. S. , Andrade, E. V. S. , Stenders, R. M. , Federico, C. , & Silva, A. X. (2020). Urban critical infrastructure disruption after a radiological dispersive device event. Journal of Environmental Radioactivity , 222 , 106358.
- Assante, M. J. (2009, January). Infrastructure protection in the ancient world. In System Sciences, 2009. HICSS’09. 42nd Hawaii International Conference on (pp. 1–10). IEEE.
- Baker, A. B. , Eagan, R. J. , Falcone, P. K. , Harris, J. M. , Herrera, G. V. , Hines, W. C. , Hutchinson, R. , Moonka, A. , Swinson, M. , Webb, E. , Woodall, T. D. , & Wyss, G. (2002). A scalable systems approach for critical infrastructure security . Sandia National Laboratories.
- Baker, C. , Fratto, D. , & Reiners, L. (2019). Banking on the Cloud. Tennessee Journal of Business Law , 21 , 381.
- Barabadi, A. , Ghiasi, M. H. , Qarahasanlou, A. N. , & Mottahedi, A. (2020). A holistic view of health infrastructure resilience before and after COVID-19. Archives of Bone and Joint Surgery , 8 (Suppl 1), 262.
- Baubion, C. (2013). OECD risk management: Strategic crisis management (OECD Working Papers on Public Governance 23). Organization for Economic Cooperation and Development.
- Bernardi, L. , Morales, L. , Lühiste, M. , & Bischof, D. (2018). The effects of the Fukushima disaster on nuclear energy debates and policies: A two-step comparative examination. Environmental Politics , 27 (1), 42–68.
- Birkland, T. A. , & DeYoung, S. E. (2011). Emergency response, doctrinal confusion, and federalism in the Deepwater Horizon oil spill. Publius: The Journal of Federalism , 41 (3), 471–493.
- Blokus, A. , & Dziula, P. (2019). Safety analysis of interdependent critical infrastructure networks. TransNav, International Journal on Marine Navigation and Safety of Sea Transportation , 13 (4), 781–787.
- Boin, A. , & McConnell, A. (2007). Preparing for critical infrastructure breakdowns . Journal of Contingencies and Crisis Management , 15 (1), 50–59.
- Boin, A. , ‘t Hart, P. , Stern, E. , & Sundelius, B. (2017). The politics of crisis management: Public leadership under pressure (2nd ed.). Cambridge University Press.
- Breen, S. P. , & Markey, S. (2019). Half empty? Drinking water systems and regional resilience in rural Canada. Planning Practice & Research , 34 (2), 168–183.
- Brooker, P. (2010). Fear in a handful of dust: Aviation and the Icelandic volcano. Significance , 7 (3), 112–115.
- Brown, T. , Beyeler, W. , & Barton, D. (2004). Assessing infrastructure interdependencies: The challenge of risk analysis for complex adaptive systems. International Journal of Critical Infrastructures , 1 (1), 108–117.
- Busch, N. E. , & Givens, A. D. (2012, October). Public-private partnerships in homeland security: Opportunities and challenges . Homeland Security Affairs , 8 , Article 18.
- Chou, J. S. , & Ongkowijoyo, C. S. (2019). Hybrid decision-making method for assessing interdependency and priority of critical infrastructure. International Journal of Disaster Risk Reduction , 39 , 101134.
- Clarke, L. (1999). Mission improbable: Using fantasy documents to tame disasters . University of Chicago Press.
- de Gooyert, V. (2020). Long term investments in critical infrastructure under environmental turbulence: Dilemmas of infrastructure responsiveness . Sustainable Futures , 2 , 100028.
- Department of Homeland Security . (2013). NIPP. Partnering for Critical Infrastructure Security and Resilience . Homeland Security.
- Deverell, E. , & Olsson, E. K. (2009). Learning from crisis: A framework of management, learning and implementation in response to crises. Journal of Homeland Security and Emergency Management , 6 (1), 1–20.
- Dunn-Cavelty, M. , & Suter, M. (2009). Public-private partnerships are no silver bullet: An expanded governance model for critical infrastructure protection . International Journal of Critical Infrastructure Protection , 2 (4), 179–187.
- Edwards, P. N. (2003). Infrastructure and modernity: Force, time, and social organization in the history of sociotechnical systems. In T. J. Misa , P. Brey , & A. Feenberg (Eds.), Modernity and technology (pp. 185–226). The MIT Press.
- Eriksson, K. , & McConnell, A. (2011). Contingency planning for crisis management: Recipe for success or political fantasy? Policy and Society , 30 (2), 89–99.
- Farnham, B. R. (2000). Roosevelt and the Munich crisis: A study of political decision-making . Princeton University Press.
- Federal Emergency Management Agency (2018). 2017 hurricane season after action report .
- Fekete, A. , Tzavella, K. , & Baumhauer, R. (2017). Spatial exposure aspects contributing to vulnerability and resilience assessments of urban critical infrastructure in a flood and blackout context. Natural Hazards , 86 (1), 151–176.
- Fischbacher-Smith, D. (2006). The crisis of management: Managing ahead of the curve. In D. Smith & D. Elliott (Eds.), Key readings in crisis management: Systems and structures for prevention and recovery (pp. 301–317). Routledge.
- Fuggini, C. , & Bolletta, F. (2020). Identification of indicators, metrics and level of service for the resilience of transport critical infrastructure. International Journal of Sustainable Materials and Structural Systems , 4 (2–4), 330–346.
- Gallais, C. , & Filiol, E. (2017). Critical infrastructure: Where do we stand today? A comprehensive and comparative study of the definitions of a critical infrastructure. Journal of Information Warfare , 16 (1), 64–87.
- Gharehyakheh, A. , Tolk, J. N. , Cantu, J. , & Fritts, S. (2017). A survey paper of protecting critical infrastructure: Applying high reliability theory to advance organizational resilience (Unpublished).
- Gordon, P. , Moore, J. E. , Park, J. Y. , & Richardson, H. W. (2007). The economic impacts of a terrorist attack on the US commercial aviation system. Risk Analysis: An International Journal , 27 (3), 505–512.
- Greenberg, A. (2017) How an entire nation became Russia’s test lab for cyberwar . Wired .
- Grönvall, J. (2001). Mad cow disease: The role of experts and European crisis management. In U. Rosenthal , A. Boin , & L. Comfort (Eds.), Managing crises: Threats, dilemmas, opportunities (pp. 155–174). Charles C. Thomas.
- Grosse, C. , & Olausson, P. M. (2019). Blind spots in interaction between actors in Swedish planning for critical infrastructure protection. Safety Science , 118 , 424–434.
- Hannah, S. T. , Uhl-Bien, M. , Avolio, B. J. , & Cavarretta, F. L. (2009). A framework for examining leadership in extreme contexts. The Leadership Quarterly , 20 (6), 897-919.
- Heifetz, R. A. , & Laurie, D. L. (2001). The work of leadership. Harvard Business Review , 79 (11), 5–14.
- Helsloot, I. , & Beerens, R. (2009). Citizens’ response to a large electrical power outage in the Netherlands in 2007. Journal of Contingencies and Crisis Management , 17 (1), 64–68.
- Helsloot, I. , Boin, A. , Jacobs, B. , & Comfort, L. K. (2012). Mega-crises: Understanding the prospects, nature, characteristics, and the effects of cataclysmic events . Charles C Thomas.
- Hermann, C. F. (1963). Some consequences of crisis which limit the viability of organizations. Administrative Science Quarterly , 8 (1), 61–82.
- Hermann, M. G. , & Dayton, B. W. (2009). Transboundary crises through the eyes of policymakers: Sense making and crisis management. Journal of Contingencies and Crisis Management , 17 (4), 233–241.
- Herrera, L. C. , & Maennel, O. (2019). A comprehensive instrument for identifying critical information infrastructure services. International Journal of Critical Infrastructure Protection , 25 , 50–61.
- Holeguin-Veras, J. , Jaller, M. , Van Wassenhove, L. , Perez, N. , & Wachtendorf, T. (2012). On the unique features of humanitarian logistics . Journal of Operational Research , 30 (7–8), 494–506.
- Jennex, M. ed. (2012). Managing crises and disasters with emerging technologies: Advancements . IGI Global.
- Kaplan, S. , & Garrick, B. J. (1981). On the quantitative definition of risk. Risk Analysis , 1 (1), 11–27.
- Kitagawa, K. , Preston, J. , & Chadderton, C. (2017). Preparing for disaster: A comparative analysis of education for critical infrastructure collapse. Journal of Risk Research , 20 (11), 1450–1465.
- Kleinman, A. , & Watson, J. L. (2006). SARS in China: prelude to pandemic? Stanford University Press.
- Koraeus, M. , & Stern, E. (2013). Exploring the crisis management/knowledge management nexus. In B. Akghar & S. Yates (Eds.), Strategic intelligence management (pp. 134–149). Butterworth-Heinemann.
- Leavitt, W. M. , & Kiefer, J. J. (2006). Infrastructure interdependency and the creation of a normal disaster: The case of Hurricane Katrina and the City of New Orleans . Public Works Management Policy , 10 (4), 306–314.
- Lebow, R. N. , & Stein, J. G. (2019). Afghanistan, Carter, and foreign policy change: The limits of cognitive models. In D. Caldwell (Ed.), Diplomacy, force, and leadership (pp. 95–127). Routledge.
- Leonard, H. B. , & Howitt, A. M. (2012). Leading in crisis: Observations on the political and decision-making dimensions of response. In I. Helsloot , B. Jacobs , & A. Boin (Eds.), Mega-crises. Understanding the prospects, nature, characteristics and the effects of the cataclysmic events (pp. 25–34). Charles C. Thomas.
- Liu, W. , & Song, Z. (2020). Review of studies on the resilience of urban critical infrastructure networks. Reliability Engineering & System Safety , 193 , 106617.
- Loggins, R. , Little, R. G. , Mitchell, J. , Sharkey, T. , & Wallace, W. A. (2019). CRISIS: Modeling the restoration of interdependent civil and social infrastructure systems following an extreme event. Natural Hazards Review , 20 (3), 04019004.
- Michel‐Kerjan, E. (2003). New challenges in critical infrastructures: A US perspective. Journal of Contingencies and Crisis Management , 11 (3), 132–141.
- Millington, N. (2018). Producing water scarcity in São Paulo, Brazil: The 2014–2015 water crisis and the binding politics of infrastructure. Political Geography , 65 , 26–34.
- Monaghan, J. , & Walby, K. (2017). Surveillance of environmental movements in Canada: Critical infrastructure protection and the petro-security apparatus. Contemporary Justice Review , 20 (1), 51–70.
- Monstadt, J. , & Schmidt, M. (2019). Urban resilience in the making? The governance of critical infrastructures in German cities. Urban Studies , 56 (11), 2353–2371.
- Newlove, L. , Stern, E. , & Svedin, L. (2003). Auckland unplugged: Coping with critical infrastructure failure . Lexington.
- Nye, D. (2010). When the lights went out: A history of blackouts in America . MIT Press.
- O’Brien, P. , Pike, A. , & Tomaney, J. (2019). Governing the “ungovernable”? Financialisation and the governance of transport infrastructure in the London “global city-region.” Progress in Planning , 132 , 100422.
- Odenwald, S. F. , & Green, J. L. (2008). Bracing for a solar superstorm. Scientific American , 299 (2), 80–87.
- Organisation for Economic Cooperation and Development . (2015). The changing face of strategic crisis management . OECD Press.
- Olsson, E. K. , Stern, E. K. , & Xue, L. (2011). SARS: Meeting an epidemic head-on. In E. K. Olsson & Lan Xue (Eds.), SARS from East to West (pp. 1–16). Lexington Books.
- Parker, A. M. , Nelson, C. , Shelton, S. R. , Dausey, D. J. , Lewis, M. W. , Pomeroy, A. , & Leuschner, K. J. (2009). Measuring crisis decision making for public health emergencies . RAND Corporation, TR-712-DHHS.
- Parker, C. F. (2014). Complex negative events and the diffusion of crisis: Lessons from the 2010 and 2011 Icelandic volcanic ash cloud events. Geografiska Annaler: Series A, Physical Geography , 97 (1), 97–108.
- Perrow, C. (1984). Normal accidents: Living with high-risk technologies . Basic Press.
- Petersen, L. , Fallou, L. , Reilly, P. , & Serafinelli, E. (2017a). European expectations of disaster information provided by critical infrastructure operators: Lessons from Portugal, France, Norway and Sweden. International Journal of Information Systems for Crisis Response and Management (IJISCRAM) , 9 (4), 23–48.
- Petersen, L. , Fallou, L. , Reilly, P. , & Serafinelli, E. (2017b, December). Should CI operators use social media to communicate with the public during crisis situations?: Lessons learned from a pilot study in Oslo Harbour. In 2017 4th International Conference on Information and Communication Technologies for Disaster Management (ICT-DM) (pp. 1–6). IEEE.
- Petersen, L. , Fallou, L. , Reilly, P. , & Serafinelli, E. (2020). Public expectations of critical infrastructure operators in times of crisis. Sustainable and Resilient Infrastructure , 5 (1–2), 62–77.
- Petrova, S. , & Prodromidou, A. (2019). Everyday politics of austerity: Infrastructure and vulnerability in times of crisis. Environment and Planning C: Politics and Space , 37 (8), 1380–1399.
- Pursiainen, C. (2018). Critical infrastructure resilience: A Nordic model in the making? International Journal of Disaster Risk Reduction , 27 , 632–641.
- Rehak, D. , Senovsky, P. , Hromada, M. , & Lovecek, T. (2019). Complex approach to assessing resilience of critical infrastructure elements. International Journal of Critical Infrastructure Protection , 25 , 125–138.
- Riedman, D. (2016, May). Questioning the criticality of critical infrastructure: A case study analysis . Homeland Security Affairs , 12 (3).
- Rinaldi, S. M. , Peerenboom, J. P. , & Kelly, T. K. (2001). Identifying, understanding, and analyzing critical infrastructure interdependencies . Control Systems Magazine, IEEE , 21 (6), 11–25.
- Rød, B. (2020). Operationalising critical infrastructure resilience. From assessment to management [Dissertation]. UiT The Arctic University of Norway.
- Roe, E. (2016). Critical infrastructures, high reliability and their enemy, economism. Journal of Contingencies and Crisis Management , 24 (3), 191–196.
- Roe, E. , & Schulman, P. (2016). Reliability and risk: The challenge of managing interconnected infrastructures . Stanford Business Books.
- Rosenthal, U. , Charles, M. T. , & Hart, P. T. (1989). Coping with crises: The management of disasters, riots, and terrorism . Charles C. Thomas.
- Shakou, L. M. , Wybo, J. L. , Reniers, G. , & Boustras, G. (2019). Developing an innovative framework for enhancing the resilience of critical infrastructure to climate change. Safety Science , 118 , 364–378.
- Simon, T. (2017). Chapter seven: Critical infrastructure and the internet of things . In Cyber security in a volatile world (Research Volume 5 of the Global Commission on Internet Governance) (pp. 93–104). Chatham House.
- Soyata, T. , Habibzadeh, H. , Ekenna, C. , Nussbaum, B. , & Lozano, J. (2019). Smart city in crisis: Technology and policy concerns. Sustainable Cities and Society , 50 , 101566.
- Stern, E. (1997). Crisis and learning: A conceptual balance sheet. Journal of Contingencies and Crisis Management , 5 (2), 69–86.
- Stern, E. (1999) Crisis Decisionmaking: A cognitive institutional approach [Dissertation]. Stockholm University Department of Political Science.
- Stern, E. (2009). Crisis navigation: Lessons from history for the crisis manager in chief. Governance , 22 (2), 189–202.
- Stern, E. (2003). Crisis studies and foreign policy analysis: Insights, synergies, and challenges. International Studies Review , 5 ( 2 ), 183–202.
- Stern, E. (2013). Preparing: The sixth task of crisis leadership. Journal of Leadership Studies , 7 (3), 51–57.
- Stern, E. (2015). Understanding and identifying crises through early warning and sensemaking. In The changing face of strategic crisis management , pp.41-74. OECD.
- Stern, E. (2017a). Crisis management, social media, and smart devices. In B. Akghar , A. Staniforth , & D. Waddington (Eds.), Applications of social media in crisis management (pp. 21–33). Springer.
- Stern, E. (2017b, June) “Unpacking and exploring the relationship between Crisis Management and Social Media in the Era of ‘Smart Devices’ .” Homeland Security Affairs , 13 , Article 4.
- Stern, E. , Deverell, E. , Fors, F. , & Newlove Ericsson, L. (2014). Post-mortem crisis analysis: Dissecting the London bombings of July 2005. Journal of Organizational Effectiveness , 1 (4), 402–422.
- Thales UK Ltd. (2013). Cyber security for SCADA systems [White Paper]. Accessed May 2017.
- Trainor, J. E. , Stern, E. K. , & Subbio, T. (2018). On bridging research and practice in disaster science and management: Unified system or impossible mission? In H. Rodriguez , W. Donner , & Trainor, J. (Eds.), Handbook of disaster research (pp. 161–178). Springer.
- Weick, K. E. (1988). Enacted sensemaking in crisis situations. Journal of Management Studies , 25 , 305–317.
- Weick, K. E. (1993). The collapse of sensemaking in organizations: The Mann Gulch disaster. Administrative Science Quarterly , 38 (4), 628–652.
- Yang, C. , Su, G. , & Chen, J. (2017, March). Using big data to enhance crisis response and disaster resilience for a smart city . In 2017 IEEE 2nd International Conference on Big Data Analysis (ICBDA) (pp. 504–507). IEEE.
- Zhang, B. , Li, J. , Tian, W. , Chen, H. , Kong, X. , Chen, W. , Zhao, M. , & Xia, X. (2020). Spatio-temporal variances and risk evaluation of land finance in China at the provincial level from 1998 to 2017. Land Use Policy , 99 , 104804.
1. See, for example, Hurricane Harvey Aftermath ; Irma: A hurricane for the history books ; What's Happening With the Relief Effort in Puerto Rico? for overviews.
2. See After Chemical Fires, Texans Worry About Toxic Effects .
3. For an example of such an attack (on the U.K. National Health Service) .
4. The following four paragraphs have been adapted from Stern (2009 , pp. 190–191). It is beyond the scope of this paper to take a position on exactly how to define the term critical infrastructure and determine which infrastructures should be regarded as critical. Definitions and attributions of criticality vary considerably, and it has been suggested that some governments define the term so broadly that prioritization becomes problematic. See, for example, Riedman (2016) .
5. See Critical
6. For a critical discussion of the relative importance of these criteria, see Hermann and Dayton (2009) .
7. For an introduction to this issue .
8. See Shift in Hurricane Irma's predicted path catches western Florida off-guard . For an introduction to hurricane modeling for the non-specialist .
9. See Houston knew it was at risk of flooding, so why didn’t the city evacuate? .
10. The following paragraphs summarize the crisis leadership tasks framework developed in Boin et al. (2017) .
11. For an overview of this challenge and discussion of emerging good practices based on the results of a workshop hosted by OECD and U.K. Met .
12. See the OECD Risk Governance Tool kit for numerous examples of such efforts in the OECD countries.
13. See the power point briefing from the European Joint Research Center (Giannoupolous) .
14. See 85% of Cell Sites Down in Puerto Rico .
15. See Trump attacks San Juan mayor over hurricane response , Trump tosses paper towels into Puerto Rico crowd , and In Puerto Rico, Trumps paper towel toss reveals where his empathy lies .
16. See, for example, Lead Contamination in Flint — An Abject Failure to Protect Public Health , After Months of Anger in Hoosick Falls, Hearings on Tainted Water Begin , and Mexico City, Parched and Sinking, Faces a Water Crisis .
17. FEMA (2003, p. iii) Hurrican Sandy FEMA After Action Report
18. For a study of impacts on transportation during and after Hurricane Sandy .
19. American Waterworks and Wastewater Association WARN (Water and Wastewater Response Network) .
20. Patients Evacuated from City Medical Center After Power Failure
21. See, for example, the integrated New York City report ; the integrated FEMA after action report ; U.S. Department of Energy after action report .
22. See, for example, the presentation by M. Henauer at the Geneva workshop .
23. See Modelling Complexity and Interdependencies for Improving CI Resilience .
24. This draws on Chapter Four of OECD (2015) The Changing Face of Strategic Crisis Management and the discussion of the 2017 Geneva workshop. See also Stern (2013) ; For example, the U.S. Department of Homeland Security Critical Infrastructure Protection and Resilience Toolkit contains exercise planning resources to help operators develop table top exercises .
25. See, for example, the description of the Dutch VITEX exercise presented by Mutsaers .
Related Articles
- Networks and Crisis Management
- Managing Critical Infrastructures in Crisis
- Organizational and Institutional Crisis Management
Printed from Oxford Research Encyclopedias, Politics. Under the terms of the licence agreement, an individual user may print out a single article for personal use (for details see Privacy Policy and Legal Notice).
date: 26 April 2024
- Cookie Policy
- Privacy Policy
- Legal Notice
- Accessibility
- [66.249.64.20|185.80.151.9]
- 185.80.151.9
Character limit 500 /500
Thank you for visiting nature.com. You are using a browser version with limited support for CSS. To obtain the best experience, we recommend you use a more up to date browser (or turn off compatibility mode in Internet Explorer). In the meantime, to ensure continued support, we are displaying the site without styles and JavaScript.
- View all journals
- Explore content
- About the journal
- Publish with us
- Sign up for alerts
- Published: 31 July 2023
A socio-technical approach for the assessment of critical infrastructure system vulnerability in extreme weather events
- Juan P. Montoya-Rincon ORCID: orcid.org/0000-0002-3787-9309 1 ,
- Said A. Mejia-Manrique ORCID: orcid.org/0000-0001-7018-339X 2 ,
- Shams Azad ORCID: orcid.org/0000-0003-3683-7761 3 ,
- Masoud Ghandehari 3 ,
- Eric W. Harmsen ORCID: orcid.org/0000-0003-1462-1281 4 ,
- Reza Khanbilvardi 2 &
- Jorge E. Gonzalez-Cruz ORCID: orcid.org/0000-0002-2324-0205 1 , 5
Nature Energy volume 8 , pages 1002–1012 ( 2023 ) Cite this article
2648 Accesses
5 Citations
19 Altmetric
Metrics details
- Energy grids and networks
- Power distribution
- Social policy
The recurrence of extreme weather events has led to the development of methods for assessing the vulnerability and interdependencies of physical and human systems. A case example is Hurricane Maria (H-Maria), where Puerto Rico experienced damage to 80% of its electrical power system, leading to massive disruptions of essential services for months. Here we evaluate the effectiveness of various interventions aimed at reducing vulnerability by considering power and water infrastructure and respective water–power dependencies while also considering the social vulnerability of affected communities associated with the physical infrastructure upgrades. On the basis of the current infrastructure configuration, we found that all communities suffered enormously from power and water outages. As one upgrade option, we show that incorporating regional energy grids would reduce outages in an H-Maria scenario. However, a large portion of disadvantaged communities will face service disruption under this option. In contrast, hardening transmission lines, as the second option, would improve service delivery and, most importantly, provide uninterrupted service to the higher portion of the vulnerable population.
This is a preview of subscription content, access via your institution
Access options
Access Nature and 54 other Nature Portfolio journals
Get Nature+, our best-value online-access subscription
24,99 € / 30 days
cancel any time
Subscribe to this journal
Receive 12 digital issues and online access to articles
111,21 € per year
only 9,27 € per issue
Buy this article
- Purchase on Springer Link
- Instant access to full article PDF
Prices may be subject to local taxes which are calculated during checkout
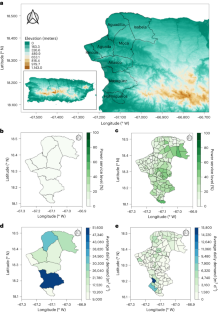
Data availability
The datasets generated and/or analysed during the current study are available in the Zenodo repository https://doi.org/10.5281/zenodo.7388021 .
Bennett, J. A. et al. Extending energy system modelling to include extreme weather risks and application to hurricane events in Puerto Rico. Nat. Energy 6 , 240–249 (2021).
Article Google Scholar
Yates, D. et al. Stormy weather: assessing climate change hazards to electric power infrastructure: a Sandy case study. IEEE Power Energy Mag. 12 , 66–75 (2014).
Schmeltz, M. T. et al. Lessons from Hurricane Sandy: a community response in Brooklyn, New York. J. Urban Health 90 , 799–809 (2013).
Pasch, R. J., Penny, A. B. & Berg, R. National Hurricane Center Tropical Cyclone Report Hurricane Maria (AL152017) 16–30 September 2017 . National Hurricane Center (2019).
Birk Jones, C., Bresloff, C. J., Darbali-Zamora, R., Lave, M. & Aponte Bezares, E. E. Geospatial assessment methodology to estimate power line restoration access vulnerabilities after a hurricane in Puerto Rico. IEEE Open Access J. Power Energy https://doi.org/10.1109/OAJPE.2022.3191954 (2022).
Lu, D. & Alcantara, C. After Hurricane Maria, Puerto Rico was in the dark for 181 days, 6 hours and 45 minutes. The Washington Post. April 4, 2018 (2018).
Mahzarnia, M., Moghaddam, M. P., Baboli, P. T. & Siano, P. A review of the measures to enhance power systems resilience. IEEE Syst. J. 14 , 4059–4070 (2020).
Panteli, M., Trakas, D. N., Mancarella, P. & Hatziargyriou, N. D. Power systems resilience assessment: hardening and smart operational enhancement strategies. Proc. IEEE 105 , 1202–1213 (2017).
Bagheri, A., Zhao, C., Qiu, F. & Wang, J. Resilient transmission hardening planning in a high renewable penetration era. IEEE Trans. Power Syst. 34 , 873–882 (2019).
Elizondo, M. A. et al. Risk-Based Dynamic Contingency Analysis Applied to Puerto Rico Electric Infrastructure (US Department of Energy, 2020); https://doi.org/10.2172/1771798
Boyle, E. et al. Social vulnerability and power loss mitigation: a case study of Puerto Rico. SSRN Electron. J. https://doi.org/10.2139/SSRN.3838896 (2021).
Kwasinski, A., Andrade, F., Castro-Sitiriche, M. J. & O’Neill-Carrillo, E. Hurricane Maria effects on Puerto Rico electric power infrastructure. IEEE Power Energy Technol. Syst. J. 6 , 85–94 (2019).
O’Neill-Carrillo, E. & Irizarry-Rivera, A. How to harden Puerto Rico’s grid against hurricanes. IEEE Spectr. 56 , 42–48 (2019).
Siddiqi, A., Kajenthira, A. & Anadón, L. D. Bridging decision networks for integrated water and energy planning. Energy Strategy Rev. 2 , 46–58 (2013).
Roni, M. S. et al. Integrated water–power system resiliency quantification, challenge and opportunity. Energy Strategy Rev. 39 , 100796 (2022).
Zuloaga, S. & Vittal, V. Integrated electric power/water distribution system modeling and control under extreme mega drought scenarios. IEEE Trans. Power Syst. 36 , 474–484 (2021).
Pant, R., Thacker, S., Hall, J. W., Alderson, D. & Barr, S. Critical infrastructure impact assessment due to flood exposure. J. Flood Risk Manage. 11 , 22–33 (2018).
Dargin, J., Berk, A. & Mostafavi, A. Assessment of household-level food–energy–water nexus vulnerability during disasters. Sustainable Cities Soc. 62 , 102366 (2020).
Flanagan, B. E., Gregory, E. W., Hallisey, E. J., Heitgerd, J. L. & Lewis, B. A social vulnerability index for disaster management. J. Homeland Secur. Emerg. Manage. 8 , 1–8 (2011).
Google Scholar
Cutter, S. L., Mitchell, J. T. & Scott, M. S. Revealing the vulnerability of people and places: a case study of Georgetown County, South Carolina. Ann. Assoc. Am. Geogr. 90 , 713–737 (2000).
Emrich, C. T. & Cutter, S. L. Social vulnerability to climate-sensitive hazards in the Southern United States. Weather Clim. Soc. 3 , 193–208 (2011).
Blaikie, P., Cannon, T., Davis, I. & Wisner, B. At risk: natural hazards, people’s vulnerability and disasters. Risk https://doi.org/10.4324/9780203974575 (2005).
West, J., Riosmena, F. & Thomas, K. Comparing social vulnerability and population loss in Puerto Rico after Hurricane Maria. M.A. thesis, University of Colorado Boulder. 1–5 (2022).
Wood, E., Sanders, M. & Frazier, T. The practical use of social vulnerability indicators in disaster management. Int. J. Disaster Risk Reduct. 63 , 102464 (2021).
Bergstrand, K., Mayer, B., Brumback, B. & Zhang, Y. Assessing the relationship between social vulnerability and community resilience to hazards. Social Indic. Res. 122 , 391–409 (2015).
Spielman, S. E. et al. Evaluating social vulnerability indicators: criteria and their application to the Social Vulnerability Index. Nat. Hazards 100 , 417–436 (2020).
Montoya-Rincon, J. P., Gonzalez, J. E. & Jensen, M. P. Evaluation of Transmission Line Hardening Scenarios using a Machine Learning Approach. ASCE-ASME Journal of Risk and Uncertainty in Engineering Systems Part B: Mechanical Engineering https://doi.org/10.1115/1.4063012 (2023).
Carvalhaes, T. et al. A simulation framework for service loss of power networks under extreme weather events: a case of Puerto Rico. In IEEE International Conference on Automation Science and Engineering 1532–1537 https://doi.org/10.1109/CASE48305.2020.9216849 (2020).
Román, M. O. et al. NASA’s Black Marble nighttime lights product suite. Remote Sens. Environ. https://doi.org/10.1016/j.rse.2018.03.017 (2018).
Montoya-Rincon, J. P. et al. On the use of satellite nightlights for power outages prediction. IEEE Access 10 , 16729–16739 (2022).
Román, M. O. et al. Satellite-based assessment of electricity restoration efforts in Puerto Rico after Hurricane Maria. PLoS ONE 14 , e0218883 (2019).
Azad, S. & Ghandehari, M. A study on the association of socioeconomic and physical cofactors contributing to power restoration after Hurricane Maria. IEEE Access 9 , 98654–98664 (2021).
Wang, Z. et al. Monitoring disaster-related power outages using NASA Black Marble nighttime light product. Int. Arch. Photogramm. Remote Sens. Spat. Inf. Sci. 42 , 1853–1856 (2018).
Innovyze Store InfoWater (Innovyze Store, 2021); https://store.innovyze.com/WaterDistribution/InfoWater?cclcl=en_US
Manrique, S. A. M., Harmsen, E. W., Khanbilvardi, R. M. & González, J. E. Flood impacts on critical infrastructure in a coastal floodplain in western Puerto Rico during Hurricane María. Hydrology 8 , 104 (2021).
US Army Corps of Engineers Gridded Surface Subsurface Hydrologic Analysis (US Army Corps of Engineers, 2022); https://www.erdc.usace.army.mil/Media/Fact-Sheets/Fact-Sheet-Article-View/Article/476714/gridded-surface-subsurface-hydrologic-analysis/#:~%7B%7D:text=GSSHA
Giovanni-Prieto, M. Development of a Regional Integrated Hydrologic Model for a Tropical Watershed. MSc thesis, Univ. of Puerto Rico, Mayaguez Campus (2007).
Rojas-González, A. M. Flood Prediction Limitations in Small Watersheds with Mountains Terrain and High Rainfall Variability. Ph.D dissertation, Univ. of Puerto Rico, Mayaguez Campus (2012).
Silva-Araya, W. F., Santiago-Collazo, F. L., Gonzalez-Lopez, J. & Maldonado-Maldonado, J. Dynamic modeling of surface runoff and storm surge during hurricane and tropical storm events. Hydrology 5 , 13 (2018).
Moriasi, D. N., Gitau, M. W., Pai, N. & Daggupati, P. Hydrologic and water quality models: performance measures and evaluation criteria. Trans. ASABE 58 , 1763–1785 (2015).
Yuan, H., Zhang, W., Zhu, J. & Bagtzoglou, A. C. Resilience assessment of overhead power distribution systems under strong winds for hardening prioritization. ASCE-ASME J. Risk Uncertainty Eng. Syst. Part A Civ. Eng. 4 , 04018037 (2018).
Salman, A. M., Li, Y. & Stewart, M. G. Evaluating system reliability and targeted hardening strategies of power distribution systems subjected to hurricanes. Reliab. Eng. Syst. Saf. 144 , 319–333 (2015).
Wang, Y., Rousis, A. O. & Strbac, G. On microgrids and resilience: a comprehensive review on modeling and operational strategies. Renew. Sustain. Energy Rev. 134 , 110313 (2020).
Aros-Vera, F., Gillian, S., Rehmar, A. & Rehmar, L. Increasing the resilience of critical infrastructure networks through the strategic location of microgrids: a case study of Hurricane Maria in Puerto Rico. Int. J. Disaster Risk Reduct. 55 , 102055 (2021).
Puerto Rico Integrated Resource Plan 2018-2019 Vol. 1 (Siemens Industry, 2019); https://energia.pr.gov/wp-content/uploads/sites/7/2019/06/2-IRP2019-Main-Report-REV2-06072019.pdf
Zamzam, A. S., Dall’Anese, E., Zhao, C., Taylor, J. A. & Sidiropoulos, N. D. Optimal water–power flow-problem: formulation and distributed optimal solution. IEEE Trans. Control Network Syst. 6 , 37–47 (2019).
Article MathSciNet MATH Google Scholar
Shinozuka, M. et al. Resilience of integrated power and water systems. Seism. Eval. Retrofit Lifeline Syst. 65 , 65–86 (2003).
Dugan, J., Byles, D. & Mohagheghi, S. Social vulnerability to long-duration power outages. Int. J. Disaster Risk Reduct. 85 , 103501 (2023).
Tarling, H. A. Comparative Analysis of Social Vulnerability Indices: CDC’s SVI and SoVI . Division of Risk Management and Societal Safety, Lund University (2017) .
Cutter, S. L., Boruff, B. J. & Shirley, W. L. Social vulnerability to environmental hazards*. Social Sci. Q. 84 , 242–261 (2003).
Carvalhaes, T. et al. Integrating spatial and ethnographic methods for resilience research: a thick mapping approach for Hurricane Maria in Puerto Rico. Ann. Am. Assoc. Geogr . https://doi.org/10.1080/24694452.2022.2071200 (2022).
Chakalian, P. M., Kurtz, L. C. & Hondula, D. M. After the lights go out: household resilience to electrical grid failure following Hurricane Irma. Nat. Hazards Rev. 20 , 05019001 (2019).
Puerto Ricans at risk of waterborne disease outbreaks in wake of Hurricane Maria. CBS News. Oct 25, 2017 (2017).
Breiman, L. Random forests. Mach. Learn . https://doi.org/10.1023/A:1010933404324 (2001).
Pokhrel, R., Cos del, S., Montoya Rincon, J. P., Glenn, E. & González, J. E. Observation and modeling of Hurricane Maria for damage assessment. Weather Clim. Extremes https://doi.org/10.1016/j.wace.2021.100331 (2021).
Skahill, B. E., Charles, D. W. & Bagget, J. S. A Practical Guide to Calibration of a GSSHA Hydrologic Model Using ERDC Automated Model Calibration Software – Effective and Efficient Stochastic Global Optimization. U.S. Army Engineer Research and Development Center (2012).
2014–2018 ACS 5-year Estimates (US Census Bureau, 2018); https://www.census.gov/programs-surveys/acs/technical-documentation/table-and-geography-changes/2018/5-year.html
OpenStreetMap contributors. Planet Dump. OpenStreetMap (2017).
Tormos-Aponte, F., García-López, G. & Painter, M. A. Energy inequality and clientelism in the wake of disasters: from colorblind to affirmative power restoration. Energy Policy 158 , 112550 (2021).
Download references
Acknowledgements
The authors gratefully acknowledge the financial support received by the US National Science Foundation under grant CBET-1832678, the National Science Foundation Program for Critical Resilient Interdependent Infrastructure Systems and Processes titled ‘Integrated Socio-Technical Modeling Framework to Evaluate and Enhance Resiliency in Islanded Communities’ under award 1832678.
Author information
Authors and affiliations.
Department of Mechanical Engineering, The City College of New York, New York, NY, USA
Juan P. Montoya-Rincon & Jorge E. Gonzalez-Cruz
Department of Civil Engineering, The City College of New York, New York, NY, USA
Said A. Mejia-Manrique & Reza Khanbilvardi
Department of Civil and Urban Engineering, New York University, Brooklyn, NY, USA
Shams Azad & Masoud Ghandehari
Department of Agricultural and Biosystems Engineering, University of Puerto Rico, Mayaguez, Puerto Rico, USA
Eric W. Harmsen
Department of Atmospheric and Environmental Sciences, University at Albany, Albany, NY, USA
Jorge E. Gonzalez-Cruz
You can also search for this author in PubMed Google Scholar
Contributions
J.P.M.-R.: data collection, formal analysis, writing, review and editing. S.A.M.-M.: data collection, formal analysis, writing, review and editing. S.A.: data collection, formal analysis, writing, review and editing. M.G.: funding acquisition, review and editing. E.W.H.: funding acquisition, review and editing. R.K.: funding acquisition and review. J.E.G.-C.: funding acquisition, project lead, review and editing.
Corresponding authors
Correspondence to Juan P. Montoya-Rincon or Said A. Mejia-Manrique .
Ethics declarations
Competing interests.
The authors declare no competing interests.
Peer review
Peer review information.
Nature Energy thanks the anonymous reviewers for their contribution to the peer review of this work.
Additional information
Publisher’s note Springer Nature remains neutral with regard to jurisdictional claims in published maps and institutional affiliations.
Rights and permissions
Springer Nature or its licensor (e.g. a society or other partner) holds exclusive rights to this article under a publishing agreement with the author(s) or other rightsholder(s); author self-archiving of the accepted manuscript version of this article is solely governed by the terms of such publishing agreement and applicable law.
Reprints and permissions
About this article
Cite this article.
Montoya-Rincon, J.P., Mejia-Manrique, S.A., Azad, S. et al. A socio-technical approach for the assessment of critical infrastructure system vulnerability in extreme weather events. Nat Energy 8 , 1002–1012 (2023). https://doi.org/10.1038/s41560-023-01315-7
Download citation
Received : 25 October 2022
Accepted : 28 June 2023
Published : 31 July 2023
Issue Date : September 2023
DOI : https://doi.org/10.1038/s41560-023-01315-7
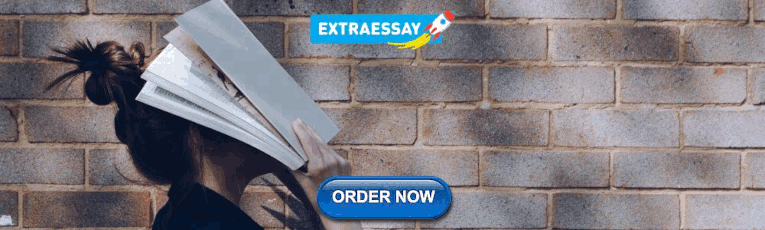
Share this article
Anyone you share the following link with will be able to read this content:
Sorry, a shareable link is not currently available for this article.
Provided by the Springer Nature SharedIt content-sharing initiative
Quick links
- Explore articles by subject
- Guide to authors
- Editorial policies
Sign up for the Nature Briefing newsletter — what matters in science, free to your inbox daily.

Why defining and securing systemically important critical infrastructure is so vital
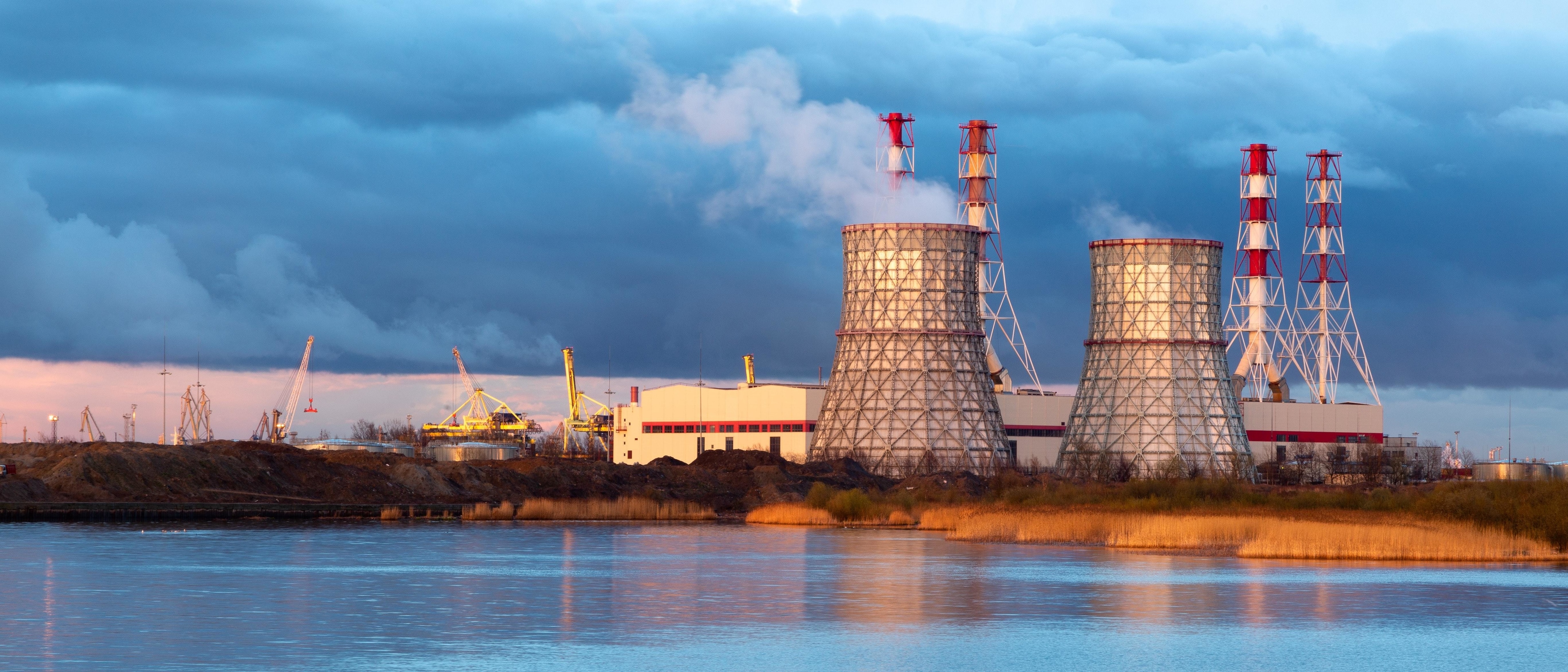
Defining 'systemically important critical infrastructure' is vital to keep services running in the event of a cyberattack. Image: Unsplash/Kirill Shavlo
.chakra .wef-1c7l3mo{-webkit-transition:all 0.15s ease-out;transition:all 0.15s ease-out;cursor:pointer;-webkit-text-decoration:none;text-decoration:none;outline:none;color:inherit;}.chakra .wef-1c7l3mo:hover,.chakra .wef-1c7l3mo[data-hover]{-webkit-text-decoration:underline;text-decoration:underline;}.chakra .wef-1c7l3mo:focus,.chakra .wef-1c7l3mo[data-focus]{box-shadow:0 0 0 3px rgba(168,203,251,0.5);} Alexander Klimburg
Akshay joshi, filipe beato.
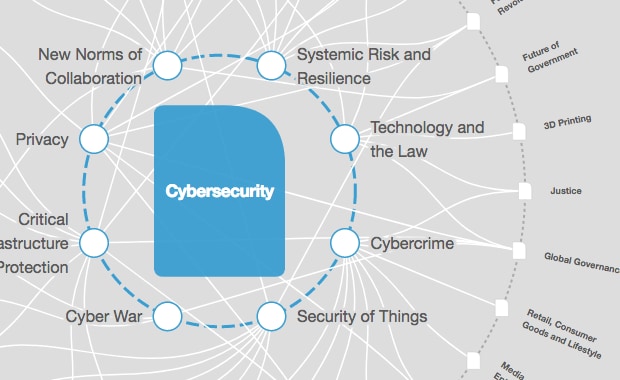
.chakra .wef-9dduvl{margin-top:16px;margin-bottom:16px;line-height:1.388;font-size:1.25rem;}@media screen and (min-width:56.5rem){.chakra .wef-9dduvl{font-size:1.125rem;}} Explore and monitor how .chakra .wef-15eoq1r{margin-top:16px;margin-bottom:16px;line-height:1.388;font-size:1.25rem;color:#F7DB5E;}@media screen and (min-width:56.5rem){.chakra .wef-15eoq1r{font-size:1.125rem;}} Cybersecurity is affecting economies, industries and global issues
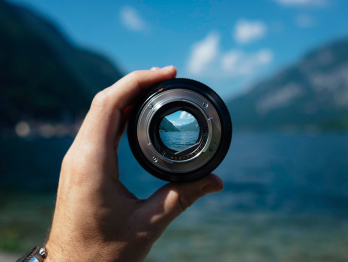
.chakra .wef-1nk5u5d{margin-top:16px;margin-bottom:16px;line-height:1.388;color:#2846F8;font-size:1.25rem;}@media screen and (min-width:56.5rem){.chakra .wef-1nk5u5d{font-size:1.125rem;}} Get involved with our crowdsourced digital platform to deliver impact at scale
Stay up to date:, cybersecurity.
- Critical infrastructure protection is vital to keep essential services running and often relies on public-private cooperation models.
- But while failure of critical infrastructure is often considered a worst-case scenario, there is often a question over who pays for its security.
- Identifying 'systemically important critical infrastructure' could help open up new cooperation models and unlock new funding mechanisms.
Government efforts to engage in critical infrastructure protection are hardly new. In the United States, the first efforts were codified all the way back in 1998 in the Presidential Decision Directive 63 , which reads:
“ Critical infrastructures are those physical and cyber-based systems essential to the minimum operations of the economy and government. They include, but are not limited to, telecommunications, energy, banking and finance, transportation, water systems and emergency services, both governmental and private. ”
This mission seems clear: to set up comprehensive public-private cooperation models that help assure the provision of essential services to the government, the economy and the public.
In the US, the governmental programmes in this regard have led to a huge increase in cybersecurity spending just in the entities directly affected – over $105 billion in 2021 alone , according to one estimate.
Challenges of critical infrastructure protection
However, despite well over two decades of experience, getting critical infrastructure protection right still seems to be a challenge. The recent Colonial Pipeline attack paralysed the gas supply on the east coast of the US. Similar impacts were witnessed as a result of the Amsterdam-Rotterdam-Antwerp attack in February 2022, and the Florida water plant incident in February 2021.
While full-scale outages in the electricity sector have yet been relatively contained, for instance the 2015 power grid hack in Ukraine , several cyber powers have reportedly prepositioned malware in each other`s power grids.
It is far from clear if critical infrastructure protection programmes would be sufficient in dealing with the effects of such a worst-case act – one that could even rise to the level of an actual cyberwar if committed by states.
The wide-scale and prolonged failure of critical infrastructure is sometimes considered the worst-case outcome of a political conflict – exactly what we would do as a society if the power fails for days, let alone weeks, is a matter of widespread speculation.
Cyberattack on critical infrastructure 'prime fear'
But the concern is not only one of overexcited journalists or filmmakers. For the Global Cybersecurity Outlook 2022 report , the World Economic Forum surveyed 120 senior cyber leaders to understand their concerns, both for their enterprises but also for themselves personally. When asked what they worried about personally, infrastructure breakdown due to a cyberattack emerged as the number one concern, substantially ahead of identity theft.
Over the last 20 years, virtually all Organisation for Economic Co-operation and Development governments have experimented with various carrots and sticks to increase private sector collaboration.
The World Economic Forum's Centre for Cybersecurity at the forefront of addressing global cybersecurity challenges and making the digital world safer for everyone.
Our goal is to enable secure and resilient digital and technological advancements for both individuals and organizations. As an independent and impartial platform, the Centre brings together a diverse range of experts from public and private sectors. We focus on elevating cybersecurity as a key strategic priority and drive collaborative initiatives worldwide to respond effectively to the most pressing security threats in the digital realm.
Learn more about our impact:
- Cybersecurity training: In collaboration with Salesforce, Fortinet and the Global Cyber Alliance, we are providing free training to the next generation of cybersecurity experts . To date, we have trained more than 122,000 people worldwide.
- Cyber resilience: Working with more than 170 partners, our centre is playing a pivotal role in enhancing cyber resilience across multiple industries: oil and gas , electricity , manufacturing and aviation .
Want to know more about our centre’s impact or get involved? Contact us .
More recent discussion in Europe and the US has concentrated on the “sticks” – in particular, new legal requirements by governments that operators of critical infrastructure must report serious breaches in their networks.
These regulations – like the EU Cybersecurity Act and the very recent US Cyber Incident reporting for Critical Infrastructure act of 2022 – were seen as relatively low-cost options and were supposed to incentivize private companies to invest more in security.
But it didn’t answer the question that is really on many critical infrastructure operators’ minds – more security and operational resilience would be great, but who was going to pay for it?
Who pays for critical infrastructure protection?
A significant challenge of critical infrastructure protection programmes is simply that the societal needs are not the same as many industry needs. For instance, the emergency services in many countries depend on the same mobile phone infrastructure as everyone else.
Cellular base stations are critical, but only few have standby generators in case of a wide scale power blackout, and only for a day or two at most. The government can (and sometimes does) force these companies to build more redundancy into these networks, but overall telecom companies work under tight profit margins making investors wary of any additional burdens.
Have you read?
A 3-step path to securing critical infrastructure, water scarcity, energy security and underinvestment in infrastructure are interconnected risks affecting development in mena countries, explainer: as cybercrime evolves, how can companies keep up with their cybersecurity, how to follow davos 2022.
And while government might also just purchase, subsidize or otherwise reward the purchase of such equipment, there may remain a legal question: if such subsidies were to apply to all critical infrastructures – and in the US these are likely to be many thousands of companies – would it not represent a major anti-competitive act, especially where being “critical” was hardly an exceptional situation anymore?
Defining systemically important critical infrastructure
The solution to this conundrum is an entire new type of critical infrastructure, which potentially may even result in an entire new type of corporation: the “systemically important critical infrastructure”.
The concept of systemically important critical infrastructure was floated in the US Cyberspace Solarium Commission’s 2020 report as “the entities, responsible for the most important critical systems and assets in the US, that would be granted special assistance from the federal government as well as assume increased responsibility for additional security and information security requirements that are vital to their unique status and importance”.
In other words, it encompasses only the “critical of the critical” enterprises – those like power and telecoms that are needed to make the others run.
In the US there is a clear move to adopting the concept wholesale, and the legislation pushed forward might represent the start of a very new idea of critical infrastructure. However, the exact deliberations of what may constitute systemically important critical infrastructure and how it can be enacted are still very much at the start.
Rethinking regulation models to ensure resilience
In addition to collaboration between governments and critical infrastructure organizations, there is a need to establish improved cost-sharing models and co-regulatory models that ensure resilience of the basic underpinnings of daily life.
A new legal category of ‘systemically important’ infrastructure may provide government with the ability to unlock new funding mechanisms that were previously unavailable. This is clearly needed for some infrastructure, whereas as mentioned previously, the sums needed to ensure business continuity and disaster recovery at the level that society may need clearly exceeds the budgets the operators can spend on this.
What is the World Economic Forum doing on infrastructure?
Infrastructure is one of the least technologically transformed sectors in the entire economy, with crucial components like construction ranking second to last in digitization according to industry rankings . Technologically-enabled infrastructure has the potential to change the way we plan, design, finance, build and operate our infrastructure systems and, more importantly, help achieve broader goals around sustainability, social cohesion and inclusive economic growth.
The World Economic Forum’s Infrastructure 4.0 initiative, supported by the Global Infrastructure Hub , is working to improve the adoption of emerging infrastructure technologies across asset and system lifecycles.
By creating recommendations for decision-makers and providing best-practice case study examples to the Global Infrastructure Hub’s G20 Infratech Use Case library , this initiative aims to refocus the infrastructure development conversation around how infrastructure as a tool to provide better outcomes in people’s lives and technology’s role in enabling this people-first future.
Beyond even capital expenditure-related measures, it could even include operational expenditure-related issues – such as the costs associated with maintaining sufficiently large cybersecurity organizations and similarly to deal with the threats at hand. The concept opens the door for creative thinking.
The new concept of systemically important critical infrastructure organizations may be the best way to cut the Gordian knot that has bedevilled public-private cooperation in critical infrastructure for decades: how to properly share the cost burden of modern societies’ reliance on certain life-essential industries. Getting this right will be a huge step in the Fourth Industrial Revolution.
Don't miss any update on this topic
Create a free account and access your personalized content collection with our latest publications and analyses.
License and Republishing
World Economic Forum articles may be republished in accordance with the Creative Commons Attribution-NonCommercial-NoDerivatives 4.0 International Public License, and in accordance with our Terms of Use.
The views expressed in this article are those of the author alone and not the World Economic Forum.
Related topics:
The agenda .chakra .wef-n7bacu{margin-top:16px;margin-bottom:16px;line-height:1.388;font-weight:400;} weekly.
A weekly update of the most important issues driving the global agenda
.chakra .wef-1dtnjt5{display:-webkit-box;display:-webkit-flex;display:-ms-flexbox;display:flex;-webkit-align-items:center;-webkit-box-align:center;-ms-flex-align:center;align-items:center;-webkit-flex-wrap:wrap;-ms-flex-wrap:wrap;flex-wrap:wrap;} More on Forum Institutional .chakra .wef-17xejub{-webkit-flex:1;-ms-flex:1;flex:1;justify-self:stretch;-webkit-align-self:stretch;-ms-flex-item-align:stretch;align-self:stretch;} .chakra .wef-nr1rr4{display:-webkit-inline-box;display:-webkit-inline-flex;display:-ms-inline-flexbox;display:inline-flex;white-space:normal;vertical-align:middle;text-transform:uppercase;font-size:0.75rem;border-radius:0.25rem;font-weight:700;-webkit-align-items:center;-webkit-box-align:center;-ms-flex-align:center;align-items:center;line-height:1.2;-webkit-letter-spacing:1.25px;-moz-letter-spacing:1.25px;-ms-letter-spacing:1.25px;letter-spacing:1.25px;background:none;padding:0px;color:#B3B3B3;-webkit-box-decoration-break:clone;box-decoration-break:clone;-webkit-box-decoration-break:clone;}@media screen and (min-width:37.5rem){.chakra .wef-nr1rr4{font-size:0.875rem;}}@media screen and (min-width:56.5rem){.chakra .wef-nr1rr4{font-size:1rem;}} See all
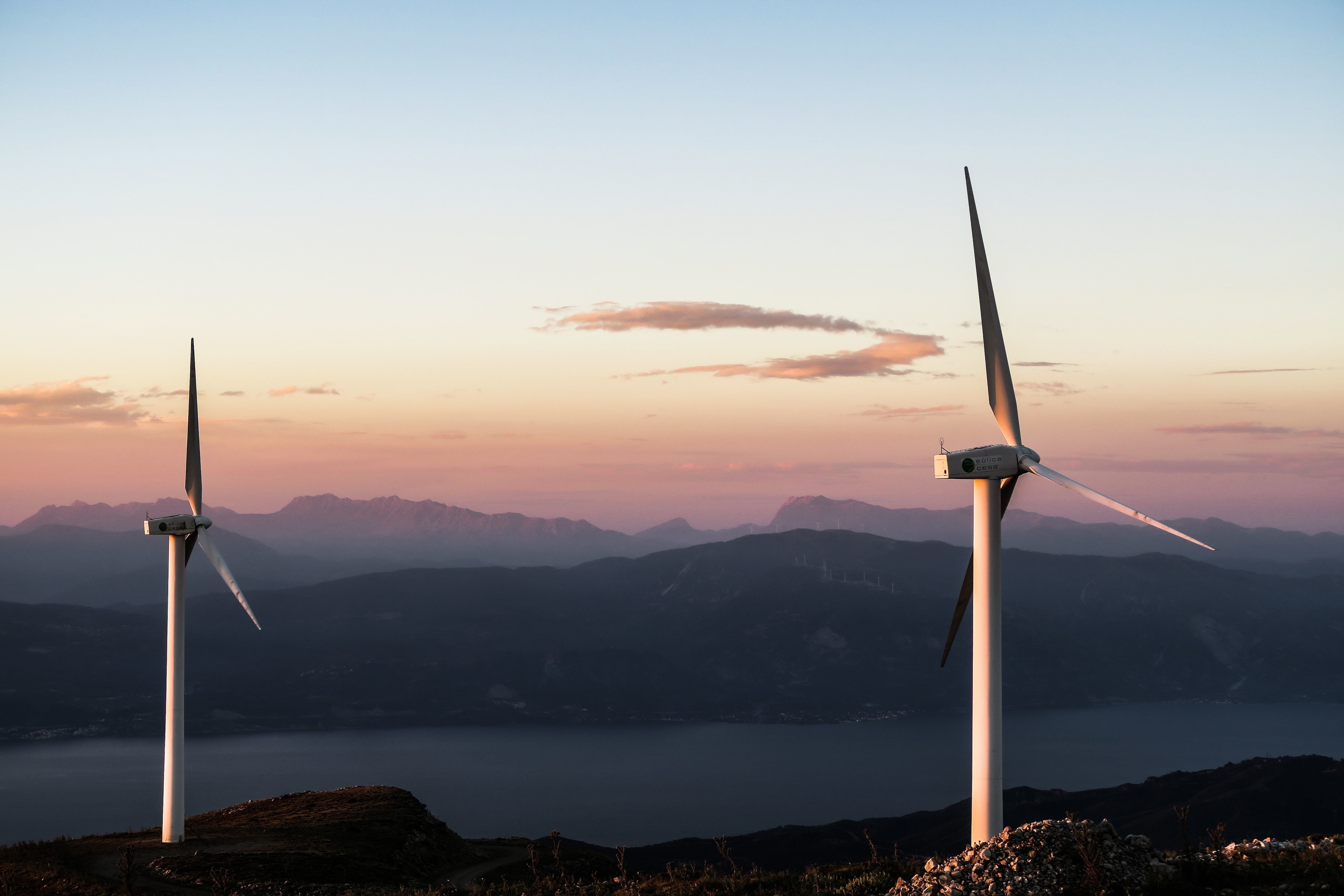
Climate finance: What are debt-for-nature swaps and how can they help countries?
Kate Whiting
April 26, 2024
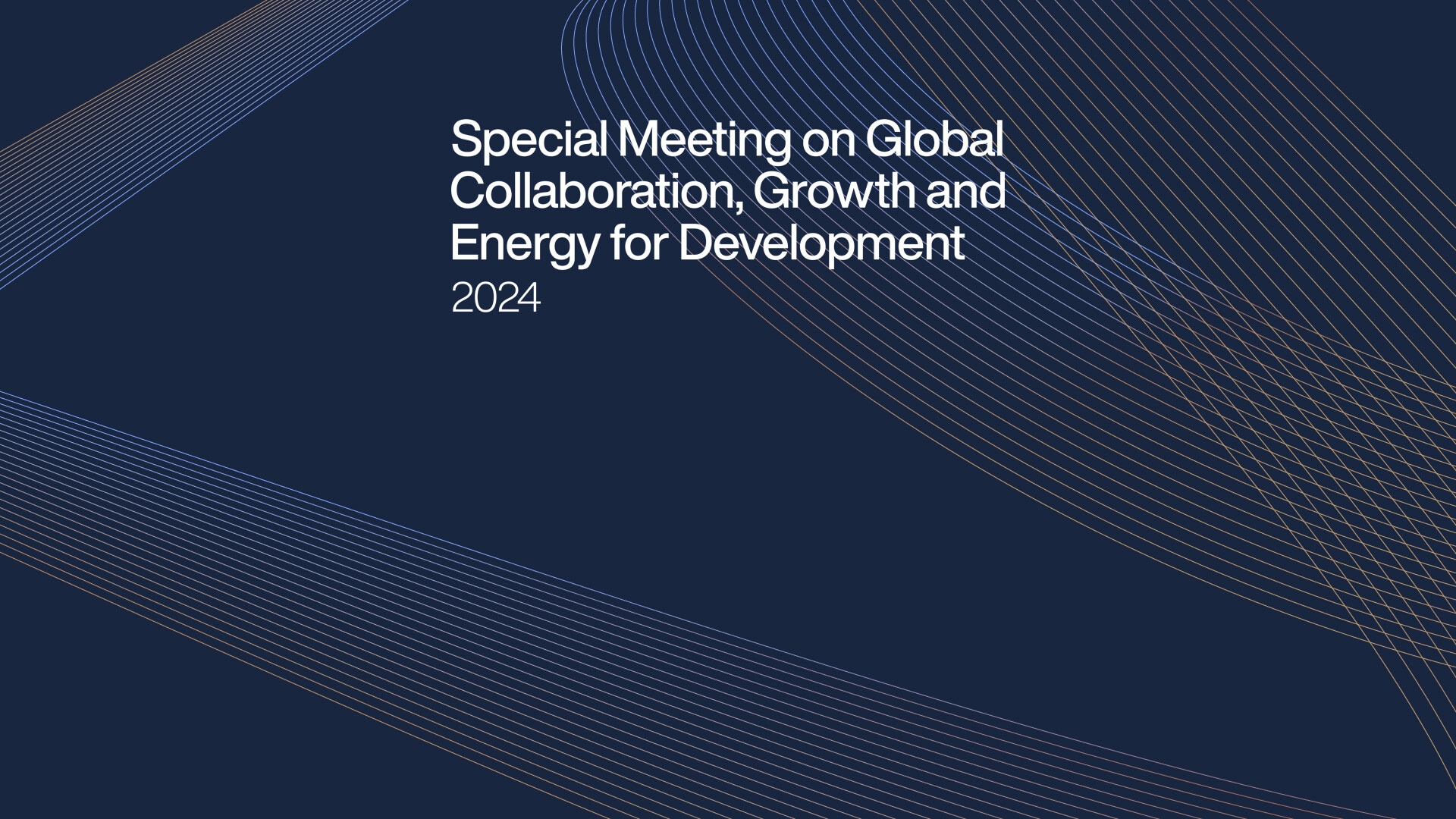
What to expect at the Special Meeting on Global Collaboration, Growth and Energy for Development
Spencer Feingold and Gayle Markovitz
April 19, 2024
From 'Quit-Tok' to proximity bias, here are 11 buzzwords from the world of hybrid work
April 17, 2024

Davos 2024 Opening Film
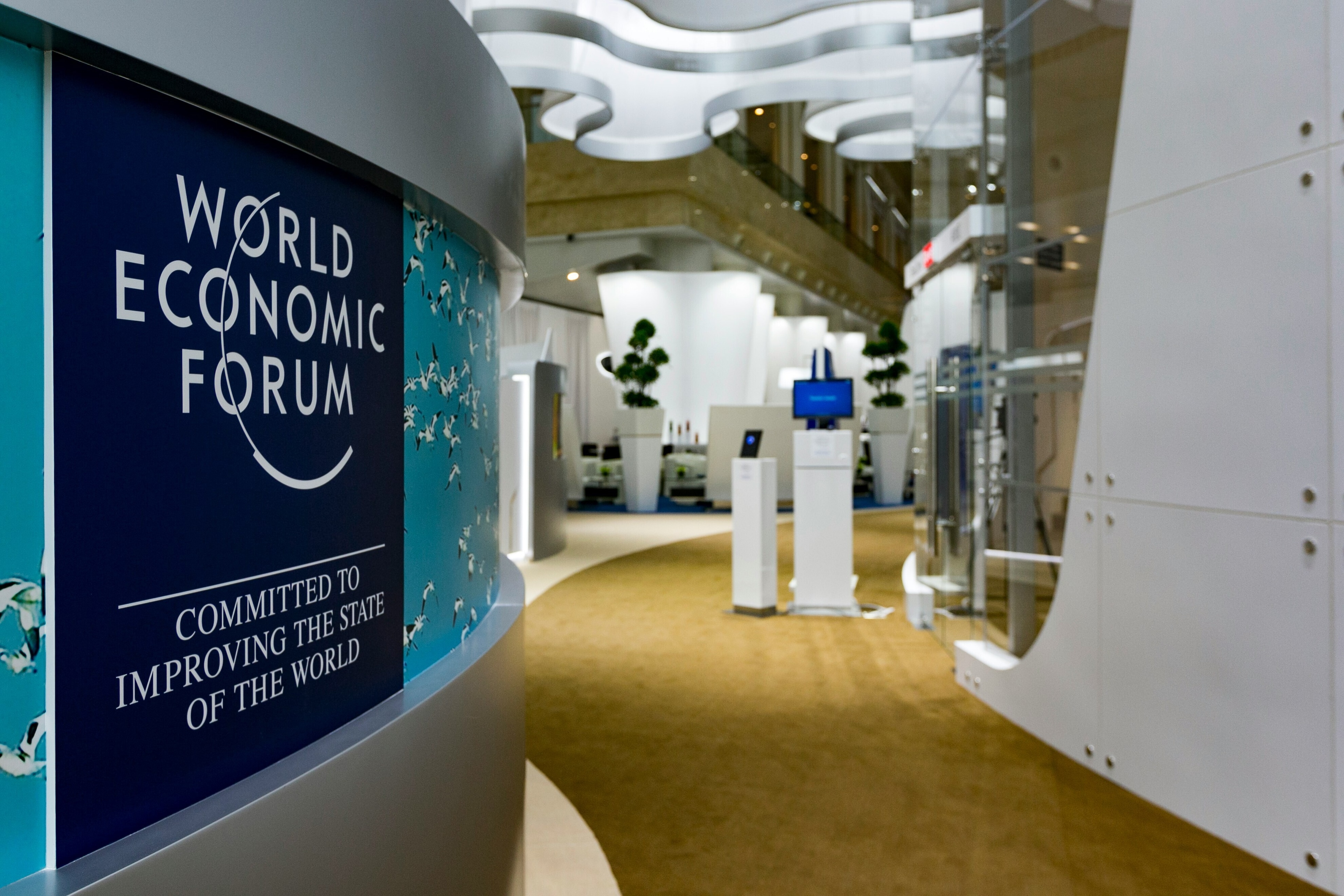
Building trust amid uncertainty – 3 risk experts on the state of the world in 2024
Andrea Willige
March 27, 2024
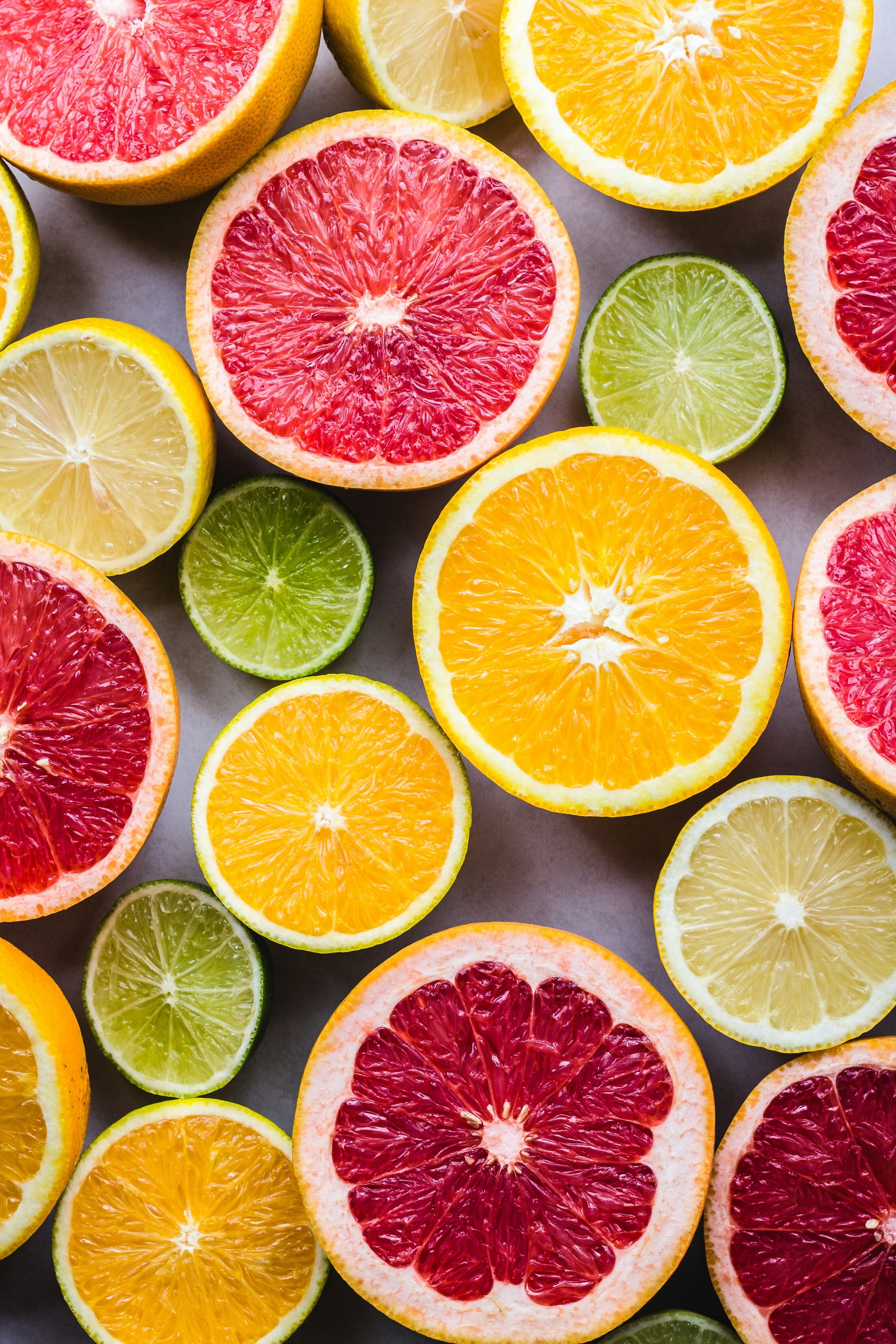
Why obesity is rising and how we can live healthy lives
Shyam Bishen
March 20, 2024
The Journal of the NPS Center for Homeland Defense and Security
Incorporating Prioritization in Critical Infrastructure Security and Resilience Programs
By Duane Verner, Frederic Petit, and Kibaek Kim
Protecting critical infrastructure, especially in a complex urban area or region, should focus on identifying and prioritizing potential failure points that would have the most severe consequences. Such prioritization can inform targeted planning and investment decisions, such as what infrastructure should be hardened or relocated first or what infrastructure should receive priority restoration following a disaster, among other uses. Without a prioritization process, assessment and protection programs are typically guided by intuition or expert judgement, and they often do not consider system-level resilience. While understanding how to prioritize high-consequence failure points for assessments and, for protection is essential, the complexity of infrastructure systems can quickly overwhelm. For example, in a notional region with 1,000 electric power assets, almost one million failure scenarios are associated with an N-2 contingency and nearly one billion failure scenarios are associated with an N-3 contingency. As a result, it is simply not feasible technically nor financially for system operators and government agencies to assess and prepare for all possible disruptions. Therefore, a primary goal of critical infrastructure protection and resilience programs should be to identify and prioritize the most critical contingencies affecting infrastructure systems. Achieving this goal will allow decision makers to identify high-impact isolated failures as well as cascading events, and to prioritize protection investments and restoration planning accordingly. To solve this problem, Argonne National Laboratory developed an optimization framework capable of modeling and prioritizing high-consequence failure points across critical infrastructure systems. The optimization framework can model at the system level or the interdependent “system-of-systems” level and is applicable to any infrastructure.

Suggested Citation
Verner, Duane, Frederic Petit, and Kibaek Kim. “Incorporating Prioritization in Critical Infrastructure Security and Resilience Programs.” Homeland Security Affairs 13, Article 7 (October 2017). https://www.hsaj.org/articles/14091
Introduction
Argonne National Laboratory (Argonne) has developed an optimization algorithm and modeling framework capable of identifying the highest-consequence failure points within critical infrastructure systems. The optimization algorithm and framework can be applied to any infrastructure at the system level or the interdependent “system-of-systems” level and can be used to model any combination of infrastructure failures. Results from the optimization modeling can be used by analysts to identify priority assets for assessments and to assist infrastructure system owners and operators and government agencies when they are making critical infrastructure protection and mitigation investment decisions.
Understanding Infrastructure Failures
A fundamental component of critical infrastructure security and resilience programs should include understanding how, why, and where systems fail. This understanding should guide decisions on where to conduct in-depth assessments as well as which protection and mitigation measures to pursue. However, a complicating factor is that infrastructure failures vary significantly. Some failures will generate significant consequences at the system or regional level, whereas effects from other failures remain local, while still others have little to no effect on the overall service provided. For illustration purposes, Figure 1 shows a 345-kV electric power transmission system between a generator substation and a remote substation.

Figure 01. Electric Transmission Lines 1
In this example, the generation plant produces 1,520 MW 2 of power that is transported to the remote substation via three transmission corridors. Corridor 1 combines two circuits (lines) that allow transport of a maximum of 750 MW. Corridor 2 is a single circuit that allows transport of a maximum of 400 MW. Corridor 3 combines two circuits that allow transport of a maximum of 800 MW. By design, the three corridors operate below their maximum capacity levels, which allows for the relocation of power among the remaining circuits in the event of a disruption in one of them. For example, if the Corridor 2 circuit fails, the system’s overall vulnerability will increase but it will not experience cascading system failure because the two other corridors can compensate for the loss (Figure 2).

Figure 02. Loss of Corridor 2 Circuit
Corridor 1 circuits would operate at 97% of their capability and Corridor 3 circuits would operate at 91% of their capability. Similarly, the loss of one circuit from Corridor 1 would not trigger a cascading system failure because of the ability of the remaining circuits to compensate (Figure 3).

Figure 03. Loss of One Circuit in Corridor 1 3
Building on the operating conditions identified in Figure 3, Corridor 3 would operate near full capacity (97%); Corridor 2 would operate at 72%; and the remaining circuit of Corridor 1 would operate at 103%, which, over time, could lead to the loss of the second circuit and therefore a failure of Corridor 1 (Figure 4).

Figure 04. Loss of Two Circuits in Corridor 1
A loss of Corridor 1 would impede the ability of the two other corridors to operate safely. Corridor 2’s circuit would operate at 104% of its capability, and Corridor 3’s circuits would operate at 129% of their capability. Under this scenario, the circuits could begin to heat and ultimately trip, triggering a system failure. Assuming all other risk factors are equal, this simplified example shows that the consequence of disruption of Corridor 1 is greater than disruption of Corridor 2, and, as such, Corridor 1 should receive priority when making security and risk management decisions.
Infrastructure fails in many different ways with varying consequences. This N-1 contingency test shows that this system can sustain the disruption of Corridor 2. However, in our example, the loss of one circuit in Corridor 1 would generate an overuse of the remaining circuit in the corridor and could lead to additional consequences. The N-1 contingency can be mitigated by shedding some of the load to bring the transfer capability in Corridor 1 back to 100%, which could avoid problems leading to the N-2 contingency case. The N-2 contingency test, resulting in the total loss of the two circuits in Corridor 1, would cascade to the two other corridors and lead to an overall system failure.
While this section focused on electric power, there are many similar nuances associated with failures in other infrastructure. For example, within the telecommunications sector, loss of a cellular tower does not necessarily mean that your phone will lose service, the closing of a road does not always mean that you can’t get to your destination, and so on. In other words, infrastructure system failures are not all created equal.
The Need for Prioritization
Without a prioritization process, infrastructure assessment, protection and mitigation programs are typically guided by intuition or expert judgement, and they often do not consider system-level reliability, redundancy, and overall resilience. While understanding how to prioritize high-consequence failure points for assessments and, for protection is essential, the complexity of infrastructure systems can quickly overwhelm decision-makers. For example, in a region with 1,000 electric power assets, almost one million failure scenarios are associated with an N-2 contingency, and nearly one billion failure scenarios are associated with an N-3 contingency (Figure 5). As a result, system operators and government agencies find it technically and financially prohibitive to assess and prepare for all possible disruptions.

Figure 05. Possible Failure Scenarios with an N-3 Contingency for 1,000 Electric Power Assets
Therefore, a primary goal of critical infrastructure protection and resilience programs should be to identify and prioritize critical contingencies affecting infrastructure systems. Achieving this goal will allow decision makers to identify high-impact isolated infrastructure failures, as well as cascading events, and to prioritize protection investments and resilience planning accordingly. Such an approach should also consider infrastructure interdependencies.
Considering Infrastructure Interdependencies
Interdependencies among critical infrastructure assets increase risk to individual assets and the overall system. These interconnected infrastructure components constitute a “system of systems” where the failure of one or multiple infrastructure elements can cascade and affect the resilience of the entire system and ultimately the region. Figure 6 illustrates interdependencies among seven different infrastructure sectors and subsectors.

Figure 06. Critical Infrastructure Interdependencies 4
However, as highlighted in the earlier electricity example, simply identifying connections between infrastructure does not provide a sufficient understanding of why or whether a connection is critical to the operational integrity of the system. The following case study of electric power and natural gas interdependencies in Florida further illustrates this point. Because Florida is a terminal state, this case study represents one of the simplest examples of interactions between electric power and natural gas because there is no complex downstream system to consider that could further propagate the disruption. Furthermore, the natural gas system is relatively simple with only two major high-pressure transmission pipelines serving the state (i.e., Florida Gas Transmission Co, and Gulfstream Natural Gas System). Figure 07. Cascading Failure Simulation in Florida shows the results of the cascading failure simulation between natural gas and electric distribution systems in Florida.

Figure 07. Cascading Failure Simulation in Florida
The scenario postulates the occurrence of a guillotine (i.e., complete) break on a major interstate transmission pipeline supplying natural gas to the state, resulting in a 100% reduction in the flow of gas through the pipeline. The pipeline break also disrupts fuel delivery to a large number of gas-fired power plants in the state. These power plants would cease operation, leading to a statewide electricity outage with varying load curtailment intensity ranging from 10% to 100%. 5
In addition, the scenario assumed that Florida has three small natural gas processing plants located in an area that would experience a 40% percent load curtailment, requiring them to curtail operations temporarily. However, because the combined output from these facilities is small relative to the total load, the associated gas curtailment would have no notable impact on gas customers in Florida. 6
As discussed in the previous section, infrastructure failures are not all created equal. When interdependencies are involved, a failure in one infrastructure can cascade to other systems increasing the overall consequences. Therefore, considering interdependencies should be an integral part of critical infrastructure security and resilience programs.
Applying an Optimization Algorithm to Prioritize Infrastructure
Managing risk associated with infrastructure inte rdependencies requires an understanding of infrastructure failures and, especially in complex urban environments, an ability to prioritize protection and mitigation efforts. Argonne has developed an optimization algorithm for selection and prioritization of infrastructure that runs at the system-level or the interdependent “system of systems-level”. The algorithm can apply to the assessment of any infrastructure system.
The optimization algorithm assumes that the physical behavior of a system (e.g., a power network, gas pipeline, or coupled system) is described by the following optimization problem:
F(d) := min u Є U(d) f(u)
d is the 0-1 vector representing the failures at infrastructure assets,
u is the control(s) that can be manipulated to mitigate disturbances, and
f(u) is a system output metric of interest such as cost, delivered load, or deviations from a target operation.
This problem can be solved by the generalized Benders decomposition method proposed by Salmeron et al. (2009). 7 This method solves the master problem max d Є D F(d) by iteratively approximating the function F(d) with a set of linear inequalities. Set D contains a set of failure scenarios denoted by d . An element of the set D is denoted by d = (d 1 , d 2 , …, d n ) , where an element d i of the vector is either 0 or 1 for i = 1, …, n to create a combination of the asset states. For example, d = (0,0,1,0) can model an event in which, out of n = 4 assets, the third asset is disrupted whereas the other assets are not.
The dependence of the control set U(d) on d captures the fact that the control actions available to counteract the disruption might be affected by the disruption d . The control set implicitly captures the network topology and physical laws of an infrastructure system.
Worst-case contingency analysis aims to find a contingency that causes the maximum damage to the system. The worst-case event (denoted by d (1) can be found by solving the optimization problem:
D(1) = argmax d Є D min u Є U(d) f(u)
The second most damaging event (denoted by d (2) ) can be identified by restricting the event set as D\{ d (1) } and by solving the problem d (2) = argmax d Є D\{d(1)} min u Є U(d) f(u). This procedure can be applied recursively to identify the k -th most damaging disturbance. This step is performed by restricting the disturbance set as D\{ d (1) , d (2) , …, d (k-1) }. Our optimization algorithm systematically restricts the disturbance set by iteratively adding the linear inequalities to the worst-case interdiction problem. This approach significantly saves the computational times, as compared with an exhaustive search.
The algorithmic steps are then summarized for identifying the most damaging disturbances as follows:
- Create the initial set of disturbances D and the control set U(d) that is dependent on disturbance d Є D . Set k = 1.
- Solve the worst-case interdiction problem to find d(k) = armax d Є D min u Є U(d) f(u).
- If k = K, then STOP .
- Update the disturbance set in order to exclude the k -th most damaging disturbance d (k) .
- Update k = k + 1, and go to step 2.
In step 2 of this algorithm, updating the disturbance set (step 4) is also equivalent to adding a linear constraint to the Benders master problem. The optimization algorithm has been implemented in Julia script language, and CPLEX is used to solve the master and subproblems in the generalized Benders decomposition.
Argonne has applied this optimization algorithm to a test system of the California Independent System Operator (CAISO) interconnected with the Western Electricity Coordinating Council (WECC). The test system is obtained from Kim et al. (2017). 8 This test system consists of 225 buses, 375 transmission lines, 135 generation units, and 40 loads. 9 The algorithm ran to detect the 100 most critical substations in the system. The criticality of substations is measured based on the amount of load lost resulting from the event that a substation is disabled. In this computational test, the objective function f(u) is defined as the amount of load lost. The control set U(d) is defined by a set of constraints for the security-constrained economic dispatch problem as in Kim et al. (2017). 10 Note, however, that our algorithmic approach is generic to have a user-defined objective function and additional constraints (e.g., generation cost, repair time of the failure components etc.). Figure 8 shows the results based on the test system.

Figure 8. Result of the Optimization Algorithm for the Test System of CAISO Interconnected with the WECC
In this example, a total of 36 substations resulted in significant load loss and failures; the other substations did not cause any load loss. The optimization algorithm terminated after the detection of zero-load substation failure. Government analysts and infrastructure owners and operators can use this type of information to protect the highest consequence failure points within infrastructure systems.
Protecting critical infrastructure, especially in complex urban areas, should focus on identifying and prioritizing potential failure points that would have the most severe consequences. Applying a technique like this optimization algorithm can inform this prioritization process. For example, the algorithm can identify the highest-consequence failures resulting from a cyber-attack against a specific critical infrastructure system, or identify the most consequential failures affecting complex interdependent infrastructure systems supporting a large urban area, regardless of the cause of disruption. Infrastructure system owners and operators, and government agencies can use results from optimization modeling to identify priority assets for in-depth security and resilience assessments, and to inform investment decisions related to critical infrastructure protection and mitigation.
Argonne is currently refining the optimization algorithm framework described within this paper through the Resilient Infrastructure Initiative, which is funded through Laboratory Directed Research and Development (LDRD) resources. 11 The list of critical assets resulting from the optimization algorithm can be analyzed further by infrastructure impact models such as EPfast 12 for electric power. Because of the computational complexity of assessing high numbers of infrastructure connections and associated failure scenarios, these studies are performed on Blues, a 350-node, high-performance computing cluster at Argonne.
About the Authors
Duane Verner is the Resilience Analysis Group Leader within the Global Security Sciences Division at Argonne National Laboratory. He oversees staffing and technical assignments, including critical infrastructure vulnerability assessments, modeling, and dependency analyses. He has provided methodology development and project implementation support to the U.S. Department of Homeland Security Regional Resiliency Assessment Program since its inception in 2009. Duane is vice-chair of the National Academies Transportation Research Board’s (TRB) Committee on Critical Transportation Infrastructure Protection and a member of the TRB Military Transportation Committee. He regularly contributes to the international resilience research community through publications and trans-Atlantic collaboration. Prior to his position with Argonne, he was a project manager for a private sector engineering firm in New York City, working in the transportation, homeland security, and defense sectors. He may be reached at [email protected]
Frédéric Petit is a Research Scientist specializing in critical infrastructure interdependencies and resilience at Argonne National Laboratory. With a background in earth sciences and civil engineering, Dr. Petit has focused on risk management and business continuity since 2002. Dr. Petit leads the development of methodologies for the assessment of preparedness, mitigation, response, recovery, and overall resilience capabilities of facilities, communities, and regions. He also lends his expertise to work on risk, vulnerability and threat analysis of critical infrastructure. Dr. Petit received his PhD from the École Polytechnique de Montreal in Civil Engineering, focusing on vulnerability analysis techniques for critical infrastructure cyber dependencies. Dr. Petit is member of various program committees for conferences, such as the Symposium on Risk Management and Cyber-Informatics (RMCI) and the National Symposium on Resilient Critical Infrastructure. He serves as Regional Director for North America of the International Association of Critical Infrastructure Protection Professionals (IACIPP) and is member of the International Advisory Board for the SmartResilience Project. He may be reached at [email protected]
Kibaek Kim is an assistant computational mathematician in the Mathematics and Computer Science Division at Argonne National Laboratory. He holds Ph.D. and M.S. degrees from Northwestern University and a B.S. degree from Inha University in Korea, all in industrial engineering. He currently serves as a reviewer for several peer-reviewed journals, including Operations Research , Mathematical Programming , Computational Optimization and Applications, European Journal of Operational Research , and IEEE Transactions on Power Systems . His research interests are in modeling and parallel algorithms for large-scale optimization problems in applications to network design, planning, and operations. He may be reached at [email protected]
Acknowledgment
The work presented in this paper was partially supported by Argonne National Laboratory under U.S. Department of Energy contract number DE-AC02-06CH11357. The submitted manuscript has been created by UChicago Argonne, LLC, Operator of Argonne National Laboratory (“Argonne”). Argonne, a U.S. Department of Energy Office of Science laboratory, is operated under Contract No. DE-AC02-06CH11357. The U.S. Government retains for itself, and others acting on its behalf, a paid-up nonexclusive, irrevocable worldwide license in said article to reproduce, prepare derivative works, distribute copies to the public, and perform publicly and display publicly, by or on behalf of the Government.
If you would like more information regarding this paper, please contact Duane Verner at [email protected] .
1 The percentages represent the line transfer capabilities.
2 About 5% of power is lost during transmission because of energy dissipated in the conductors and the equipment used for transmission. Thus, from a starting generation capability of 1,520 MW, a maximum of about 1,450 MW of power arrives at the substation. For the purpose of illustration, the example assumes that electric power is divided equally among the transmission circuits that remain operable.
3 For the purposes of illustration, the example assumes that electric power is divided equally among the transmission circuits that remain operable. In a real case, it would be expected that Corridor 2 would operate at higher capacity to compensate.
4 Adapted from J. Phillips, et al. , State Energy Resilience Framework , Argonne National Laboratory, Global Security Sciences Division, (2016) ANL/GSS-16/4, Argonne, Ill, USA, available at https://www.energy.gov/sites/prod/files/2017/01/f34/State%20Energy%20Resilience%20Framework.pdf , accessed February 14, 2017.
5 E. Portante et al. , “Modeling Electric Power and Natural Gas Systems Interdependencies,” The CIP Report , Center for Infrastructure Protection and Homeland Security, George Mason University School of Law, Washington, D.C., USA, May–June 2016, available at http://cip.gmu.edu/2016/06/03/modeling-electric-power-natural-gas-systems-interdependencies/ , accessed February 14, 2017.
7 J. Salmeron, K. Wood, and R. Baldick, “Worst-Case Interdiction Analysis of Large-Scale Electric Power Grids ,” IEEE Transactions on Power Systems 24.1: (2009) 96–104.
8 Kibaek Kim, et al., “Data Centers as Dispatchable Loads to Harness Stranded Power,” IEEE Transactions on Sustainable Energy 8.1 (2017): 208-218.
11 Argonne Energy and Global Security, undated, Resilient Infrastructure , available at https://www.anl.gov/egs/group/resilient-infrastructure , accessed February 14, 2017.
12 E.C Portante et al., “EPfast: A Model for Simulating Uncontrolled Islanding in Large Power Systems,” Proceedings of the Winter Simulation Conference , 2011 Winter Simulation Conference.
Copyright © 2017 by the author(s). Homeland Security Affairs is an academic journal available free of charge to individuals and institutions. Because the purpose of this publication is the widest possible dissemination of knowledge, copies of this journal and the articles contained herein may be printed or downloaded and redistributed for personal, research or educational purposes free of charge and without permission. Any commercial use of Homeland Security Affairs or the articles published herein is expressly prohibited without the written consent of the copyright holder. The copyright of all articles published in Homeland Security Affairs rests with the author(s) of the article. Homeland Security Affairs is the online journal of the Naval Postgraduate School Center for Homeland Defense and Security (CHDS).
More Articles

Cyber Border Security – Defining and Defending a National Cyber Border

Applying an Organizational Framework to Examine Jihadi Organizations as an Industry

A Right-Brained Approach to Critical Infrastructure Protection Theory in support of Strategy and Education: Deterrence, Networks, Resilience, and “Antifragility”

The Roots of Community Resilience: A Comparative Analysis of Structural Change in Four Gulf Coast Hurricane Response Networks
1 thought on “incorporating prioritization in critical infrastructure security and resilience programs”.
Priority acceleration piece is missing. After the Fukishima Nuclear Meltdown, the routine business accelerates to criticality like the airline industry network. So if the network is down a week, the priority stays low then the backlog goes critical and severe consequences. Then after disaster recovery, it returns to routine.
The same for the telephone service. This can be perceived as routine, but after a disaster every one calls those affected by the disaster to see if they are okay. So it means any critical call get stopped by the bulk traffic. So you have to properly size the telephone service and prioritize the traffic to get the critical calls through. This involves priority acceleration. Should the routine call, like food service, be stopped, the backlog goes critical and must restored. Then after disaster recovery, it returns to routine.
This hsaj article provides additional insght on topics pertaining to the Argonne model similar to:
White, Richard. “Towards a Unified Homeland Security Strategy: An Asset Vulnerability Model (AVM) .” Homeland Security Affairs 10, Article 1 (February 2014). https://www.hsaj.org/articles/254
Leave a Comment Cancel Reply
Your email address will not be published. Required fields are marked *

You are using an outdated browser. Please upgrade your browser to improve your experience.
Partnership
Partner with Microsoft
Maximize your partnership
Strengthen your security
Compare offerings
Microsoft Action Pack
Go to market
Marketing resources
Commercial marketplace
Sell with Microsoft
Cloud Solution Provider
New commerce experience
Partner incentives
Differentiate
Solutions Partner designations
Specializations
Azure Expert MSP
By opportunity
Device partners
Learning partners
Education partners
By solution area
Solution areas
Azure for partners
Business Applications for partners
Modern Work for partners
Security for partners
By initiative
Build for 2030
Inclusive economy
Microsoft Inspire
Partner Communities
Microsoft Partner Community
Partner-led associations
Microsoft Partner blog
Find a partner
Partner Center
All Microsoft
Netways IAC Mastery delivers hassle-free deployment of Azure resources and lowers change failure rate by 50%
By designing and implementing secure and scalable solutions on Microsoft Azure, Netways IAC Mastery service helps customers cut costs by 40%.
Complex and expensive infrastructure management
An iac service using microsoft resources, improving outcomes by minimizing inefficiencies, a microsoft partner using infrastructure as code.
With over two decades of proven expertise in delivering turnkey solutions based on Microsoft technologies, Netways uses a blend of advisory, project, and managed services to accelerate digital transformation. Headquartered in Riyadh, Saudi Arabia, Netways serves more than 2,500 customers through 12 global locations, with a solid presence in the MENA region and in North America.
As a member of the Microsoft Business Applications Inner Circle, an exclusive community comprising top-tier Microsoft partners, Netways delivers consultancy and high-quality business technology solutions in the financial, government, healthcare, education, retail, telecommunication, and engineering sectors. The company offers these services and solutions utilizing a variety of Microsoft technologies, such as Azure DevOps, Azure Resource Manager, Dynamics 365, and Power Platform.
Netways IAC Mastery, available in Azure Marketplace , is a service the company provides to create and control cloud resources in Microsoft Azure with code. IaC employs DevOps practices and versioning to define and deploy infrastructure, ensuring the same environment is consistently generated every time it's deployed.
Microsoft provides native support for IaC via the Azure Resource Manager model to manage cloud infrastructure projects and assets. Azure Resource Manager uses declarative templates instead of scripts to manage cloud infrastructure projects and assets. It centralizes an organization’s billing by grouping resources and using tags for logical organization. Users can create and re-create the solution during the development process and ensure consistent states. It also works well with all Azure services and provides a management framework to lock, tag, and secure Azure resources after deployment.
It is one of the most dependable IaC tools because of its continuous monitoring, support, and smart management administrative framework.
Helping customers cut costs and avoid pitfalls
"Our journey with Infrastructure as Code marks a pivotal moment in our operations,” said Armand Honein, Unit Manager at Netways. “By codifying infrastructure, our customers achieved greater agility and consistency. The ability to automate deployments and scale rapidly has not only enhanced efficiency but has truly transformed how we approach IT. Our commitment to innovation is mirrored in the code that now defines infrastructure."
Using Netways IAC Mastery service, organizations were able to lower change failure rate by 50 percent on average and recover from incidents 24 times faster than before. Customers are no longer stuck with hardware expansion problems such as disks, storage, electricity, and network switches. With just the push of a button, all these elements can be effortlessly integrated, allowing for seamless operation. This has cut costs by 40 percent on average and all resources are up and running 99.95 percent of the time.
"Partnering with Microsoft has been instrumental in realizing the full potential of Infrastructure as Code. The comprehensive suite of tools, especially Azure Resource Manager and Azure DevOps, has empowered our customers to streamline processes. Through this collaboration, we've witnessed a significant reduction in deployment times, improved resource utilization, and enhanced collaboration between development and operations teams. Microsoft's commitment to providing robust IaC solutions has been a key driver of our success in modernizing our customers’ infrastructure,” said Carla Naufal, Director, Strategic Partnership, Netways.
“Microsoft's commitment to providing robust IaC solutions has been a key driver of our success in modernizing our customers’ infrastructure.”
Share your success.

An official website of the United States government
The .gov means it’s official. Federal government websites often end in .gov or .mil. Before sharing sensitive information, make sure you’re on a federal government site.
The site is secure. The https:// ensures that you are connecting to the official website and that any information you provide is encrypted and transmitted securely.
- Publications
- Account settings
Preview improvements coming to the PMC website in October 2024. Learn More or Try it out now .
- Advanced Search
- Journal List
- Elsevier - PMC COVID-19 Collection

The emergence of new critical infrastructures. Is the COVID-19 pandemic shifting our perspective on what critical infrastructures are?
Cornelia scholz.
a Austrian Institute of Technology, Vienna, Austria
Stefan Schauer
Martin latzenhofer, associated data.
Data will be made available on request.
Our modern world is highly dependent on the functioning of a complex system of interdependent infrastructures. Failure of one infrastructure can have severe and far-reaching impacts on other infrastructures and jeopardize the functioning of the whole system. While certain infrastructures have been considered highly critical and their dependencies and protection has been addressed extensively and for decades, others have been considered less or not at all critical and have been barely debated. The COVID-19 pandemic has caused an unprecedented strain on infrastructure systems and has revealed that different infrastructures become highly critical throughout an ongoing and long-lasting crisis than during a sudden but short-term crisis. This paper investigates the representation of critical infrastructure dependency descriptions in the literature before and since the start of the COVID-19 pandemic. In this qualitative study, the quantity of descriptions per critical infrastructure dependency is analyzed and visualized and used to discuss the perception of how critical those infrastructures are. The study revealed that new infrastructures have been identified as critical in recent literature and that the focus was shifted to specific infrastructures that were in more pressing need during the pandemic. This shift of focus was observed to happen from the sectors of energy, water, transport & traffic, and ICT before the start of the COVID-19 pandemic to the sectors public health, constitutional institutions, transport & traffic, and food since the start of the COVID-19 pandemic. Further, analysis of the literature revealed infrastructures which had previously not been classified as critical, being discussed as new critical infrastructures. Urban green spaces, for example, have proven to be essential for the health and well-being of citizens during lockdown times. Further, social services like childcare, care of the elderly, delivery services, and online grocery shopping have been highlighted as essential services for maintaining workforces and the functioning of society during a pandemic. Overall, the analysis of descriptions of critical infrastructure dependencies before and since the start of the COVID-19 pandemic has revealed changes in the focus on critical infrastructures and in the perception of what makes critical infrastructures critical .
1. Introduction and state of the art
Dependencies of critical infrastructures can be documented, analyzed and investigated in multiple ways. Case studies (e.g., Schneidhofer & Wolthusen (2015) [ 1 ]) or incident reports (e.g., Hassanzadeh et al. (2019) [ 2 ]) document failures and their effects in real scenarios, while theoretical modelling and simulations (e.g., Rinaldi 2002 [ 3 ], Setola 2008 [ 4 ], Oliva et al. (2010) [ 5 ], Cavallini et al. (2014) [ 6 ]) investigate and envision what might be done in order to prepare responsible actors, processes and systems to protect critical infrastructures from potential threats. Luiijf et al. (2009 [ 7 ]) took an empirical approach, analyzing critical infrastructure dependencies in Europe from a database of public reports. All these investigation approaches focus on either past or potential future events or failures of critical infrastructures in order to gain a comprehensive understanding of critical infrastructures, their systems, interactions and dependencies. However, documentation and research articles align in their perception of what critical infrastructures are, focusing efforts on those pre-defined critical infrastructures. Meanwhile, the COVID-19 pandemic unraveled and challenged what is seen as a critical infrastructure. Prior to 2020 the topic of new or emerging critical infrastructures was a rather rare focus in the literature. Since the start of the global COVID-19 pandemic articles have started to emerge addressing potential new, situational critical infrastructures and their impacts on society and citizens’ welfare (e.g., urban green spaces: Xie et al. (2020) [ 8 ]; Venter et al. (2020) [ 9 ]). However, no substantial overview analysis has been conducted yet, analyzing those newly emerging topics, and reflecting on the perception of critical infrastructures in the literature affected by the impacts of the COVID-19 pandemic. This study aims to fill this knowledge gap and analyze if and how the COVID-19 pandemic impacted the representation and understanding of critical infrastructures in the literature.
The objectives of this study are:
- 1. To identify changes of critical infrastructure dependencies described in the literature before the start and since the start of the COVID-19 pandemic.
- 2. To investigate the impact of the COVID-19 pandemic on the understanding of what critical infrastructures are.
2. Critical infrastructure and society
Our contemporary society depends on infrastructures and their services, as well as the supply of essential goods. Critical infrastructures are those infrastructures and their components and services, which are essential for the functioning of the social system [ 10 ]. Critical infrastructures include basic supply networks (water, electricity), networks of information and communication services, social services (e.g., care of children or elderly), as well as complex networks of essential supply to the population (e.g., medical supply, food supply). Infrastructure systems and their components are interdependent. If a disruption or failure occurs in one system, it can spread to other systems and subsequently amplify the impact of a disruption many times and impact further systems. Due to the constant growth and increase in complexity of the connections between infrastructures, the interdependencies within the overall system also continue to increase, causing the system to become more susceptible to disruption [ 3 ]. A critical infrastructure system (e.g., public health system) comprises several sectors, with networks and components, interconnected at nodes. This creates a complex network of interconnections, whose characteristics determine how and with what intensity the effects of critical infrastructure system failures spread to dependent subsystems and society [ 11 ].
Records of past events illustrate how severe and far-reaching the effects of critical infrastructure system failures can be. During the past months, the world's countries and their infrastructures have faced a new challenge: the COVID-19 pandemic. Unlike sudden events, such as flash floods or terrorism attacks, a pandemic causes continuous stress on infrastructures. Failure does not occur abruptly, but rather announces itself and the cascading effects reach sectors which have typically not been affected in past events. For example, the Austrian food sector was affected by border closures as harvest workers from Eastern Europe could not enter the country. The lack of suitable workers threatened the harvest and thus the food production of the season [ [12] , [13] , [14] ]. Within the social and distribution systems sector, there was a shortage of elderly care workers who could not travel to their workplaces due to the border closures and lack of transportation [ 15 ]. With a large portion of the population working from home, the strain on information and communication services increased significantly [ 14 ]. To prevent overloading the connections, major providers such as YouTube and Netflix decided to temporarily reduce the video quality to relieve the Internet lines [ 16 ]. These are just a few examples of the impacts the COVID-19 pandemic has had on critical infrastructure systems. The consequences are far-reaching and long-lasting and cannot be fully assessed at this point in time. But what can be said with certainty is that the current situation highlights the importance of researching critical infrastructures, with their interrelationships, impacts, and the interconnectedness of our modern world more thoroughly than ever before. After all, only interconnections that are recognized and understood can be protected.
2.1. When systems fail – cases of critical infrastructure failure
What happens when system failures of critical infrastructure occur despite all precautions? In recent years, there have been several incidents worldwide that led to disruptions and failures of critical infrastructures and continued as cascading failures in other systems. A few example cases are described below in more detail in order to give real-world insights on potential causes of failure and the following impact those failures caused on other infrastructures and subsequently, people's lives.
One of the first extensively documented events was the Canadian ice storm in the winter of 1998. The event began with several waves of freezing rain that caused outages in the transmission and distribution network of the power supply. Power supply disruption turned an originally climatological catastrophe into a technological disaster, as infrastructures dependent on electricity began to fail. 4.7 million inhabitants were without power, and for many the disruption lasted for hours, days or even weeks. The transport & traffic sector was immediately affected as traffic lights failed, causing several rear-end collisions and subsequent traffic jams. Furthermore, rail traffic had to be stopped, as crossing signals, operating signals, and the setting of points failed. As the power outage continued, it started to affect food production and other infrastructures. Farmers suffered major losses due to lack of ventilation and heating in barns, and even the loss of dairy cows due to milking machine failures. Countless other problems arose due to heating system failures. Four people died from hypothermia and seven others died from carbon monoxide poisoning due to the use of poorly ventilated heating sources. An additional burden was placed on firefighters when some homes and apartments caught fire due to inadequate heating sources. The events of the 1998 ice storm led to increased awareness of the interdependencies of critical infrastructures in Canada, and the protection of these was included as an essential element of disaster preparedness [ 17 ].
An event that shook the world was the terrorist attack on the World Trade Center in 2001. In addition to the social and political impact, the attacks also led to failures in several of New York City's infrastructure systems. The collapsing buildings caused severe damage to the pipes of the water system. Initially, flooding of the area and surrounding areas occurred, followed by a loss of pressure in water mains and fire hydrants, which hampered the work of firefighters. The outflowing water from burst pipes flooded the underground tunnels of the PATH trains, which subsequently failed. In addition, the basement of Verizon's 140 West Street building was flooded, resulting in a loss of data and communications network outages which affected approximately 24,000 customers. A long-term consequence of this event was, that the U.S. tightened its critical infrastructure protection strategies [ 18 ].
In 2005, the city of New Orleans was hit hard by Hurricane Katrina. Hurricane Katrina was an infrastructure disaster: when the levees failed, all infrastructure systems (power, communications, water, energy, sewage) necessary for human survival in New Orleans failed as well. Without the necessary infrastructure services, medical care, public health, and relief and emergency services failed. In addition to the damage from high winds, excessive rainfall and resulting flooding and failure of parts of the levee system caused severe damage to New Orleans’ infrastructure. New Orleans' two major water treatment plants were inoperable due to excessive wind damage and flooding, and pumps of the city potable water systems failed due to power outages, resulting in a shortage of potable water [ 19 ]. About seven years later, Hurricane Sandy hit the North American East Coast, causing power outages and flooding. The most critical impact on transportation infrastructure was caused by the flooding of the New York Metropolitan Transit Authority (MTA) subway tunnels, which left millions of commuters stranded for several days. In terms of interdependencies between infrastructures, the traffic & transportation sector was mainly affected by cascading failures, initiated from areas of commercial power generation, oil and gas supply, and ICT systems. Simultaneously, cascading effects of the transport & traffic sector prolonged repair work: blocked roads or lack of access to the terrain prolonged outages, especially of the energy and ICT infrastructures [ 20 ].
2.2. Critical infrastructures in the literature
Critical infrastructures consist of a complex composition of systems, facilities, processes, networks, or parts thereof [ 10 , 21 ]. A dependency between infrastructures arises from the linkage or connection between two infrastructures through which the state of the second depends on the state of the first. There is a unidirectional connection between them: in a dependency from infrastructure j to infrastructure i , infrastructure i depends on infrastructure j through the link; but infrastructure j does not depend on infrastructure i through that link. For example, a power plant requires supplies such as workforces, fuel, and a functioning transport & traffic sector in order to operate. In this case, the energy sector is the supported sector, while the sectors transport & traffic and chemical industry are the supporting sectors. In case of an emergency, the type of dependency on critical infrastructures needed for the immediate functioning of the power plant might shift (e.g., transport & traffic needed for the deployment of repair crews) [ 3 ].
An interdependency refers to a bidirectional relationship between two infrastructures through which the states of the two infrastructures affect each other: Infrastructure i is dependent on Infrastructure j ; and Infrastructure j is dependent on Infrastructure i . The concept of interdependencies is simple, meaning the connection between different parts of infrastructures, including paths, feedback, and feedforward in a system is bi-directional. However, the complexity of the system is strongly increased with the increase of interdependencies between infrastructures. This complex web of connections and linkages can transmit shocks across numerous infrastructures and cause far-reaching and unforeseeable impacts. Therefore, it is essential to analyze and understand infrastructures in their interconnected environment. For example, the ICT sector requires power supply for its operation; the energy sector, in turn, depends on an intact ICT network in order to function and to coordinate repairs in case of a failure [ 3 ]. Impact Due to the dependencies and interdependencies between critical infrastructures, there are several ways how an event in one infrastructure can have an effect on or within another infrastructure ( Table 1 ).
–This table describes four types of possible effects between two infrastructures, where a change in an initially affected infrastructure I impacts the functioning of the affected infrastructure II. Those effects can be positive (an improvement of an infrastructure e.g., repair) or negative (causing stress on an infrastructure and lead to further disruption or failure).
Failure Critical infrastructures are constantly exposed to threats that fall into five categories [ 22 ]:
- • Climatological threats (e.g., hurricanes, major fires, flooding)
- • Geological threats (e.g., earthquakes, volcanic activity, landslides)
- • Biological threats (e.g., pandemics)
- • Technological threats (e.g., radiation emergencies, chemical accidents, major road, rail, or air accidents)
- • Criminal threats (e.g., terrorism, criminal activities, armed conflicts)
Depending on the characteristics and temporal development of the initiating event, threats can evolve into crisis or disasters of two types [ 23 ]:
- • Sudden-onset disasters are initiated by a hazardous event which emerges unexpectedly or within a very short time period (e.g., flood, earthquake, chemical explosion, volcanic eruption, transport accident, etc.) .
- • Slow-onset disasters emerge gradually over a longer period of time (e.g., sea-level rise, desertification, drought, epidemics, etc.). Slow-onset disasters differ from sudden-onset disasters in their spatio-temporal scale and impacts. While there is still a debate about the exact definition of slow-onset disasters, within this study they are understood as phenomena, which evolve slowly (over weeks, months up to years), impacting a system in various and complex ways. Typically, the type, reach, and intensity of those impacts are very difficult to anticipate The COVID-19 pandemic is considered a slow-onset disaster within this study due to its characteristics and slow development of impacts and reach. Further, the pandemic has been affecting multiple infrastructures and their dependencies and interdependencies in ways which were not anticipated, which is an example of the complexity of slow-onset disasters.
Both, sudden-onset and slow-onset disasters can cause disruptions or, in extreme cases, failures of critical infrastructure systems or subsystems. These lead to a performance drop of certain functions, whereby the severity of the drop depends on the intensity of the disruption and the resilience of the system components. Rinaldi et al. [ 3 ] defines the basic types of fault propagation in a critical infrastructure system as follows.: Cascading failure (e.g., a power failure leads to the failure of a subway line), Escalating failure (e.g., the blockage of a road by a fallen power pole impedes emergency responders from getting to a damaged transmission tower to repair the failure of an ICT network), Common cause failure (e.g., a hurricane causes simultaneous damage to the power grid, water grid, and road network in a city) [ 3 ].
3. Methodology
In this qualitative study, a systematic literature review and comparative analysis was conducted to investigate differences between the representation of descriptions in the literature of:
- • Critical infrastructure dependencies, interdependencies, and failure before the start of the COVID-19 pandemic (March 2001–March 2020)
- • Critical infrastructure dependencies, interdependencies, and failure in connection with the COVID-19 pandemic since the start of the COVID-19 pandemic (literature published between 1. March 2020–1. March 2021)
The number of literature resources analyzed for each period was not pre-determined but was determined during the course of a trial analysis. A preliminary literature analysis and visualization of the quantity of descriptions of critical infrastructure dependencies in literature was conducted through chord diagrams. For the period before the start of the COVID-19 pandemic, this trial analysis revealed that once 45 literature sources were reached, the chord diagram showed tendencies which did not noticeably change anymore by adding additional literature. For the period since the start of the COVID-19 pandemic the same approach was taken and at 35 literature resources clear tendencies showed in the chord diagram and no more sources could be identified within the period, matching the selecting criteria, and adding additional insights to the analysis.
3.1. Identification of literature
In a first step “identification”, a search for articles through online libraries, Google Scholar and an online search for grey literature was carried out. Literature was selected which described at least one dependency or connection between critical infrastructures. Literature in either English or German was reviewed. For the analysis of literature since the start of the COVID-19 pandemic, only articles including topics of COVID-19 and critical infrastructures were included. The authors are aware that this causes a bias towards articles focusing on infrastructures impacted by the pandemic. However, since this analysis focuses on changes in context of the pandemic, it was decided to apply this selection criterion. Keywords used in the search included: “critical infrastructure dependencies”; “critical infrastructure failure”; “critical infrastructure protection”; “critical infrastructure & COVID-19”. In a second step, the “snowballing” approach was applied to identify articles which did not show up in the initial search requests. In this approach, the references listed by a source that were relevant for the search topic were reviewed to identify further relevant literature. The authors decided not to limit literature to only scientific journal articles but also include case studies of real incidents, failure reports, guidelines for prevention, articles, books on critical infrastructure dependencies and/or failure, and studies of possible failure scenarios.
Specific topics of literature that was selected included among others, failure analysis of real events (e.g., ‘power outage consequences in the 1998 Ice Storm’ analysis published in Natural Hazards Journal [ 17 ]), critical infrastructure protection (e.g., ‘Water Critical Infrastructure Security and its Dependencies, published in ‘Journal of Terrorism Research’ [ 24 ]), analysis of types of failure (e.g., ‘The state and the threat of cascading failure across critical infrastructures: The implication of empirical evidence from media incident reports’ published in ‘Public Administration’ Journal [ 25 ]), topics of dependencies between specific infrastructures (e.g., ‘On the Rising Interdependency between the Power Grid, ICT Network, and E-Mobility: Modeling and Analysis’ published in ‘Energy’ Journal [ 26 ]), or specific analysis of the COVID-19 pandemic impacts on critical infrastructure sectors (e.g., ‘The COVID-19 Pandemic: Energy Market Disruptions and Resilience’ published in ‘Critical Infrastructure Policy’ Journal [ 27 ]).
3.2. Comparative analysis
Sectors of critical infrastructure that were analyzed included: public health (e.g., hospitals), the chemical industry (e.g., water treatment products), first responders (e.g., police), research facilities (e.g., epidemiological research institute), finance (e.g., banks or ATMs), ICT (e.g., cell service), transportation and traffic (e.g., street network, railway), water (e.g., water supply and sanitation), energy (e.g., electricity supply), constitutional institutions (e.g., government institutions), social systems (e.g., schools, elderly- or childcare facilities), and food (e.g., production and packaging). The sectors were defined, based on the Austrian Council of Ministers decision of April 2, 2008, classifying critical infrastructure into the above-mentioned sectors [ 21 ].
Dependency matrices were used to conduct a systematic, comparative analysis of selected literature's content. Each description of a dependency between two sectors that was identified in a literature source was noted in the matrix. ‘Active sectors’ (an initiating sector, which performance impacts other sectors) were listed along the x-axis and passive sectors (a sector which performance is impacted by the functioning of other sectors) were listed along the y-axis. After conducting the literature review, the quantity of recorded representations per dependency between two sectors was counted, representing the frequency of descriptions in literature. Further, infrastructures which were discussed as critical infrastructures in the literature but did not match any of the sectors described above were identified and discussed as “emerging critical infrastructure”.
3.2.1. Visualization of dependencies
Chord diagrams were used to visualize the results of the analysis of the quantity of descriptions of critical infrastructure dependencies between sectors in the literature. The program used for data visualization was R version 4.0.4 (2021) [ 28 ] together with the package “chorddiag”. The dependency matrixthat was created was imported into R as a matrix and visualized as an interactive graph using the Chorddiagram package.
3.2.2. Limitations
This study evolved due to the opportunity and desire to gain insights into an ongoing, and unprecedented case of a phenomenon impacting people, systems, and infrastructures around the globe. The authors acknowledge that this study is limited in its scope and that conducting this type of research has inherent limitations. Firstly, the COVID-19 pandemic is still ongoing which impacted the author's work schedule, access to resources, and collaboration opportunities. Further, no complete picture of the pandemic from the start to end can be gained and assessing longitudinal effects is currently not possible. This analysis provides a snapshot of the current situation, but insights and findings are limited to this particular period of time.
An inherent limitation of literature reviews is the subjectivity in the collection and analysis process of data and subsequently the question of reliability and replicability of results. While the authors aimed to maximize rigor and minimize susceptibility to bias in this study, it is acknowledged that analyzing literature on infrastructure dependencies and failures specifically in connection with the COVID-19 pandemic creates a selection-bias. It is further acknowledged that comparing 19 years of literature (pre-pandemic) with one year of literature (since the start of the pandemic) means analyzing across very different periods of time. The time frame of 2001–2020 was chosen in order to capture varying topics and trends across the period prior to the COVID-19 pandemic. Considering the variation in the focus on topics of critical infrastructures throughout the past two decades, the authors decided to analyze literature across a long time period, leading up the COVID-19 pandemic rather than focus on, for example, the one year before the pandemic (2019) and create a bias towards focusing on topics in that year only. A further limitation inherent to this type of research is the limited availability of reliable data. Especially in a context such as the pandemic, which is a first-time case and a new field, the validity of available information is less assured than in a well-known and well-researched field. In particular, throughout the period since the start of the COVID-19 pandemic, the number of available sources limits the scope and comprehensiveness of the study. Therefore, the authors decided to also include grey literature which may compromise the validity of the results. However, due to the objective to identify newly evolving topics and trends, the inclusion of grey literature was considered a valid addition, with the benefit outweighing the risk of compromising validity. The current limited availability of literature resources on infrastructure dependencies and failures in connection with the COVID-19 pandemic highlights the need for future research.
It is noted that an inherent limitation to literature research is that approaches can result in overly descriptive summaries, providing an overview of topics or numbers of publications without generating any deeper analysis. This paper aims to provide valuable insights and conduct a deeper analysis of emerging topics, by including an analysis of the timeline of published articles and topics since the start of the COVID-19 pandemic, identifying knowledge gaps, and using chord diagrams to analyze and derive information on perceptions and newly evolving topics of future interests and research. This study focuses on changes in topics in critical infrastructure literature and visualizing frequency of descriptions to support the argument. However, the study does not aim to make a quantitative statement, as the number of literature sources and differing time periods do not allow for a quantitative data analysis. The limited number of literature resources (45 pre-pandemic and 35 since the start of the pandemic) allows an initial insight into those changes and topics in the context of the ongoing COVID-19 pandemic. However, to gain full insight into the impact of the COVID-19 pandemic, a study will need to be carried out after the end of the COVID-19 pandemic, analyzing all COVID-19 and critical infrastructure related literature from the beginning to the end of the pandemic.
Overall, the limitations mentioned impact the study's scope which provides a very specific analysis of a subset within a limited period of time, allowing for initial insights but with limited perspective regarding future conditions. Further information and reliable publications will be essential in order to conduct an extensive and comprehensive analysis of change. As we move forward in time, the pandemic is taking its course and new articles are being published, and the trends will become clearer. In this study, new topics might be over-rated while future analysis might conclude that interest in those topics was lost with the end of the pandemic. It can be expected that time will show which new topics are truly evolving topics and which are situational and were only addressed and of interest throughout the ongoing COVID-19 pandemic.
4.1. Critical infrastructure dependencies and failures
Critical infrastructures are interconnected in many ways and interrelationships arise through mutual dependencies and impacts. In today's world, these interconnections are increasing, and infrastructures are more interwoven than ever. These pronounced interdependencies mean that infrastructures can no longer be seen as individual, self-contained systems, but must be viewed and treated as interconnected systems. Case studies from the past show the vulnerability due to this high degree of interconnectedness between the critical infrastructure sectors and potentially severe consequences that failure can trigger [ [17] , [18] , [19] , [20] , 29 ]. In this study, based on a preliminary screening and analysis process, 45 literature sources were identified and analyzed for the time before the start of the COVID-19-pandemic, and 35 literature sources for the time since the start of the COVID-19-pandemic. The analysis of existing literature on critical infrastructure interconnections revealed that dependencies between certain infrastructures are described particularly frequently. The focus of infrastructure dependency descriptions differs between the time before COVID-19-pandemic and the time since the start of the pandemic, as explained in the following sections.
Before the COVID-19 pandemic.
Dependencies between the sectors ICT, transportation & traffic, water, and energy are the ones most frequently described in the literature in the time before the COVID-19-pandemic. Fig. 1 shows the trend in the frequency with which the individual sectors are described: The link between energy to the other sectors is most prevalent in the literature, while sectors such as constitutional institutions or research facilities are hardly mentioned.

Frequency of descriptions of dependencies between critical infrastructures in literature published before the start of the COVID-19 pandemic. The band between two sectors in the graph represents the direction of dependency and frequency of description. The width of the bands at their base shows the frequency of descriptions of sectors and the direction of connections between two sectors (e.g., The sector Energy has the widest base of bands, indicating that it was most often described as the ‘active’ sector in literature, compared to all other sectors). The color shows which sector was predominantly described as ‘active’ and which was predominantly described as a ‘passive’ sector: the band has the color of the sector predominantly described as ‘passive’ (e.g., the band between Energy (yellow) and Water (dark-red) is dark red, showing that more descriptions of the dependency between those two sectors described Energy as the ‘active’ and Water as the ‘passive’ sector). A band whose base on either side has about the same width indicates that the dependency was described as equally directed in both ways (e.g., Sector Energy depends on sector ICT, and sector ICT depends on sector Energy). If a band is narrow at one base but wide at the other, it indicates that one sector has been often described as impacting the second sector, while the second sector was rarely or not described as impacting the first sector (e.g., the band between Energy and Public Health is wide at the base on the Energy side but very narrow at the base on the Public Health side. This shows that most descriptions of dependencies between the two sectors mentioned Energy as the active sector and Public Health as the predominately passive sector). Source: own diagram.
ICT, transport & traffic, water, and energy sectors are the infrastructures most frequently affected by disruptive events and most extensively described in the literature. The sectors are interlinked in complex and diverse ways and failure in one sector may rapidly and severely impact the others. The analysis further revealed trends in the focus of the descriptions of the interrelationships of these four sectors ( Fig. 2 ). While some scenarios can be found described extensively in the literature (e.g., power failure [ 30 ]), there are far less precise descriptions of other dependencies (e.g., water sector impacts on transport & traffic [ 31 ]). In most cases, major infrastructure operators are already well prepared for these forms of disruptions (e.g., emergency generators for water pumps [ 30 ]). Conversely, impacts and mitigation measures in other sectors are much less frequently described (e.g., impacts of the water sector on ICT [ 32 ]).

Frequency of descriptions of dependencies between energy (upper left), water (upper right) transportation & traffic (lower left), ICT (lower right) and other sectors in literature published before the start of the COVID-19 pandemic. The band between two sectors in the graph represents the direction of dependency and frequency of description. The width of the bands at their base shows the frequency of descriptions of sectors and direction of connections between two sectors. The color shows which sector was predominantly described as ‘active’ and which was predominantly described as a ‘passive’ sector (the band has the color of the predominantly described as ‘passive’ sector). Source: own diagram.
A comparison of the four sectors showed that in the case of cascading failure, the initiating event usually originates from the energy sector [ 25 ]. This is also reflected in the fact that critical facilities or organizations (e.g., hospitals [ 30 , 33 ]) usually have security measures primarily for power supply and only secondarily for failure of other infrastructures. Conversely, disruptions to the other sectors, result far less often in impacts on the energy sector. The sectors of constitutional institutions, research facilities and social and distribution systems are mentioned least frequently in the literature with regard to infrastructure interdependencies (e.g., descriptions of effects of infrastructure failures due to sudden-onset events like hurricanes on social systems [ 34 ]). Fig. 3 shows the quantity of descriptions of each sector described as an active sector (providing services to other sectors), highlighting the role of energy, water, transportation & traffic, and ICT as service-providing sectors, on which services other sectors depend.

Quantity of descriptions of active sectors, affecting other sectors before the COVID-19 pandemic. The size of each section represents the count of each sector, reflecting how many of the descriptions of dependencies in the literature that were analyzed addressed each sector as an active/service-providing sector (The color scheme of the sectors has no special meaning and is intended to aid the visual interpretation of the chart). Source: Own diagram.
Dependencies that lead to a rapid and severe failure of essential functions are both well researched and in practice already secured with safety measures, and the majority of case studies and research papers deal with sudden-onset events (e.g., storms), while dependencies that would only have an impact in the event of a longer-lasting disruption (several days or longer) are addressed less often and in less detail in the literature. Effects of failures that occur slowly and last for a long time are rarely described. For example, while failures initiated due to the impact of a flood or hurricane were often described, no description of infrastructure failure due to a drought was described in the literature that was reviewed.
4.2. Since the start of the COVID-19 pandemic
For the period of March 2020 to March 2021 a total of 35 literature sources were identifed that address themes of critical infrastructure protection, dependencies or failures in the context of the COVID-19 pandemic. In order to illustrate the initiation and trajectory of those evolving topics, the number of publications per month were mapped in the timeline below Fig. 4 .

Number of publications identified that were published between March 2020 and March 2021 and included themes of the COVID-19 pandemic and critical infrastructures per month. Source: own diagram.
Topics of publications included supply chain interruptions, impact on health infrastructures, transportation interruptions, food chain interruption, safety of essential work forces, education, or overall impact. Table 2 lists the publishing month and title of identified literature. A detailed reference list of identified literature can be found in Appendix A .
Month of publication and title of identified literature published between March 2020 and March 2021 and including themes of the COVID-19 pandemic and critical infrastructures.
The analysis of literature published between March 2020 and March 2021 and including themes of the COVID-19 pandemic and critical infrastructure has revealed a strong representation of descriptions of dependencies between the sectors public health, transportation & traffic, constitutional institutions and food ( Fig. 5 ).

Frequency of descriptions of dependencies between critical infrastructures in literature published between March 2020 and March 2021 and including themes of COVID-19 pandemic and critical infrastructure. The band between two sectors in the graph represents the direction of dependency and frequency of description. The width of the bands at their base shows the frequency of descriptions of sectors and direction of connections between two sectors. The color shows which sector was predominantly described as ‘active’ and which was predominantly described as a ‘passive’ sector (the band has the color of the predominantly described as a ‘passive’ sector). Source: own diagram.
The sector public health was the one most often discussed as the active sector that others depend upon and as providing services to other sectors. Following public health were the sectors transportation & traffic and constitutional institutions as providing sectors. The sector food was discussed most frequently as the one depending upon other sectors (primarily public health and transportation & traffic) to function ( Fig. 6 ).

Frequency of description of dependencies between other sectors and constitutional institutions (top left), food (top right), public health (bottom left), transport & traffic (bottom right) in literature published between March 2020 and March 2021 and including themes of COVID-19 pandemic and critical infrastructure. The band between two sectors in the graph represents the direction of dependency and frequency of description. The width of the bands at their base shows the frequency of descriptions of sectors and direction of connections between two sectors. The color shows which sector was predominantly described as ‘active’ and which was predominantly described as a ‘passive’ sector (the band has the color of the predominantly described as a ‘passive’ sector). Source: own diagram.
Public health is the sector most frequently described as the ‘active’ sector, affecting other sectors during the time of the COVID-19 pandemic ( Fig. 7 ). The analysis of Fig. 6 focused on direct sector-to-sector dependencies, but it was noticeable that many descriptions in the literature mentioned the impacts of public health on multiple sectors and society in general. Public health includes among other services the treatment of patients, and facilitation of COVID-tests and vaccinations [ 14 , 35 ]. These services have proved to be crucial for the functioning of society and all other critical infrastructures [ 36 ]. The severity of potential failure and importance of the public health sector is the most frequent theme among the articles reviewed.

Quantity of descriptions of active sectors, affecting other sectors since the start of the COVID-19 pandemic. The size of each section represents the count of each sector, reflecting how many of the descriptions of dependencies in literature that were analyzed addressed each sector as active/service-providing sector (The color scheme of the sectors has no significance other than to aid the visual interpretation of the chart). Source: Own diagram.
The most discussed dependent sector is food with a special focus on food supply chains. The COVID-19 pandemic interrupted numerous parts of the food supply chains such as production, packaging, transportation, and distribution, highlighting the dependence and vulnerability of food supply chains. The effects of the COVID-19 pandemic impacted this sector through absence of workers, closure of packaging factories and interruptions in logistics and transportation due to border-closings [ [37] , [38] , [39] , [40] , [41] ]. Transportation & traffic is discussed multiple times in connection with critical infrastructure dependencies and the COVID-19 pandemic. The interruption of transportation caused far-reaching effects on dependent sectors and supply shortages of various goods [ 14 , 40 ].
Further, the impacts and dependencies of constitutional institutions is well represented in the literature. For example, a paper by Levinson et al. (2020) discusses the impact of the decision by policy makers on whether schools would be re-opened on the health of children and the overall impact on society [ 42 ]. Due to the responsibility for establishing measures to mitigate the pandemic (e.g., lockdown regulations) this sector has proved to have a large impact on all other sectors. The impact can be restrictive (e.g., school closures) or enabling (e.g., support of public health services) [ 42 , 43 ]. Overall, the analysis of literature on critical infrastructure dependencies during the COVID-19 pandemic has revealed a clear focus on the four sectors of public health, food, traffic & transportation, and constitutional institutions.
4.3. New critical infrastructures?
The European Union defines critical infrastructures as “an asset, system or part thereof located in Member States which is essential for the maintenance of vital societal functions, health, safety, security, economic or social well-being of people, and the disruption or destruction of which would have a significant impact in a Member State as a result of the failure to maintain those functions; ” [ 10 ] .
During the COVID-19 pandemic, infrastructures which are usually not considered critical have proved to fit the classification of “critical”. For example, Geng et al. (2021) [ 44 ] discuss the importance of urban green spaces across 48 countries during lock down times, Carvalhaes et al. (2020) [ 45 ] mention various examples of essential functionalities of parks during the pandemic and Derks et al. (2020) [ 46 ] discuss the role of urban forests in Germany in providing crucial societal functions for mental and physical health of citizens during lockdown times. The authors highlight the value and previous underestimation of green areas as critical infrastructure during a pandemic. In Austria, green areas such as urban green spaces and recreational areas provided essential functions to citizens. While those spaces were not directly impacted by the pandemic, they became a critical resource and service in context of the pandemic. What if access to parks, forest, or green spaces was disrupted (e.g., through closures) and green infrastructures were not accessible during lockdown times in Austria? Especially in urban areas, the lack of such spaces would cause negative impacts on the mental and physical well-being of citizens, further negatively impacting people's performance in their work roles, including essential workers in critical infrastructures (e.g., health care workers). Following the European Union's classification of infrastructures' criticality, urban green spaces and recreational areas like forests therefore meet the classification characteristics as critical infrastructure during an ongoing pandemic [ 8 , 9 , [44] , [45] , [46] ].
Due to lockdowns and stay-at-home orders by the government, the importance of delivery services and online grocery shopping increased significantly. For vulnerable individuals these options have become crucial in daily life and have protected their health by helping to avoid potential exposure to the virus in grocery stores [ 39 , 47 ]. Further, infrastructures that have been considered less critical in the past (e.g., social systems) as their functioning is rarely severely affected during or after sudden-onset disasters, have become increasingly critical throughout the sustained pandemic. The closure of social systems like schools and childcare facilities has strained families and impacted the work performance of parents. Disruptions to social systems further raised concerns about the impact of mental health and well-being especially on children and juveniles, lacking social interactions. Here, the impacts of the pandemic have revealed the temporal characteristics of the impacts of a slow-onset crisis. While school closure for a few days is not critical for the health, well-being and overall education of students, the longer the pandemic-caused closure lasts the more severe the negative impacts become. This example shows how the criticality of infrastructures that are not usually considered highly critical can increase as the duration of a crisis increases [ 42 , 47 ]. Additional examples discussed in the literature that was reviewed address the increased importance of constitutional institutions as decision-makers [ 43 ] and the strain noted on ICT due to the increased use of services for business continuity and an increase in cybercrimes [ 47 , 48 ].
The COVID-19 pandemic further revealed the importance of essential workers for the functioning of society [ 45 , 49 ]. The absence of workforces due to sickness, transportation interruption, border-closings or private matters has jeopardized the functioning of critical services. For example, in June 2020, the German news station ‘Tagesschau’ published an article on a critical lack of trained workers in the care of the elderly in Germany. This shortage was caused by border closings due to the pandemic, which prevented Polish care workers to travel to Germany for work [ 15 ]. Walters et al. (2020) describe how the absenteeism of workforces in concentrated industries (e.g., meat packaging) due to either illness or the fear of exposure to COVID-19 caused delays in operations. Those delays further impacted the supply chains and the availability of food products in the USA [ 49 ]. The first example showcases the issue of how the pandemic impacted one infrastructure (transportation & traffic due to border closings) which led to far-reaching impacts on the availability of workforces in other sectors (health and social services). The second example shows how the pandemic directly impacted the infrastructure food, through the absence of workforces due to illness and/or fear of contracting the virus at the workplace. Those examples highlight the important role of workforces as essential elements in critical infrastructure systems and their functioning. Considering preparedness and protection of critical infrastructures in case of a pandemic, the sudden absence of a large number of workforces must be considered and prepared for. Further, occupational safety measures need to be put in place in order to keep operations functioning, to provide essential goods and services [ 50 , 51 ].
Altogether, the analysis of emphases and topics connected to the COVID-19 pandemic and critical infrastructures has revealed that new and emerging topics are increasingly discussed by authors, raising questions about what is understood as critical infrastructure.
5. Discussion
5.1. impacts of a slow-onset crisis.
Critical infrastructure failures triggered by sudden-onset events (e.g., blackout, earthquakes) are extensively described in literature, while the effects of slowly evolving strains on critical infrastructure systems (e.g., effects of an ongoing pandemic) were rarely described before the start of the COVID-19 pandemic. One of the reasons that impedes the mitigation of slow-onset crisis is that the effects of the crisis are not immediately visible. They form over time, and some can be predicted, while some cannot, but overall, it is highly challenging to assess the approaching crisis in advance. The COVID-19 pandemic poses an example of how a crisis was approaching, but awareness alone did not equate to mitigation. This is also reflected in the strain on infrastructures. While sudden-onset events like blackouts may cause severe and immediate failure, a slow-onset event like the COVID-19 pandemic starts to form slowly and the strain on critical infrastructures builds up over time. For example, the pandemic did not cause immediate and absolute disruption of work and education like a significant, sudden disaster would have done, but impacts were slowly yet long-lasting. The advantage of dealing with slow-onset crisis in contrast to sudden-onset disasters is that potentially there is time to set new mitigation measures and coping strategies tailored to counteract the effects of the approaching crisis. A disadvantage is that the perception of an approaching crisis might be subject to doubt and a government and people might be reluctant to embark on action for something that has not happened and therefore not yet affected them. The pandemic further highlighted the differences of a crisis impacting society as a whole as opposed to a sudden and intense but localized disaster (e.g., earthquake). While the impacts of localized disasters are immediate and severe, the region's or country's infrastructure usually maintains its functioning, supporting the limited geographic area impacted by the disaster.
The literature analysis revealed that before the COVID-19 pandemic, critical infrastructures which are typically not affected or less affected during a sudden-onset crisis, were considered as overall less critical. Recent events of the COVID-19 crisis showed that a pandemic causes steady and prolonged stress on the overall system which causes different infrastructures to become critical compared with those impacted by sudden-onset events. It will need further investigation after the end of the COVID-19 pandemic to determine whether the impact of a slow-onset crisis reveals critical infrastructures which are critical but were not recognized before, and their criticality continues to be recognized after the pandemic ends, or whether certain infrastructures only become critical in the immediate context of and only for the duration of a certain crisis. For example, the services and products of research facilities (e.g., vaccines, epidemiological analysis & information provision) became highly essential commodities during the COVID-19 pandemic, resulting in a major impact on the overall system. While research services and products are important independently of any crisis, their direct and far-reaching impact on other critical infrastructures (e.g., providing vaccines to essential health care workers) strongly increased their situational criticality in context of the COVID-19 pandemic. So far, there has been little description of which infrastructures become essential for the well-being of the population in such a case. The example of the importance of urban green spaces for physical and mental health during lock-down times shows how criticality can emerge due to a specific crisis setting. The COVID-19 pandemic led to many sports and recreational facilities (e.g., gyms, public pools) being closed for long periods of time. Considering the temporal aspect of a slow-onset crisis, if those facilities were only closed for a few days, it would hardly impact citizens’ health and well-being. But the long-term closure (weeks to months) created an essential need for people to find alternative spaces for recreation and physical activity, which were provided by green infrastructures. This example shows a situational creation of criticality due to the impacts and characteristics of a slow-onset crisis.
A further characteristic of a slow-onset crisis is the impact on the overall vulnerability of society. An analysis of the impact of the functioning of the public health sector has shown that health institutions, social systems and constitutional institutions significantly impact vulnerabilities across society. Health institutions, care centers for the elderly and schools have a high density of vulnerable individuals, who depend on service provisions [ 36 ]. During a pandemic, those most vulnerable are also those most at risk. Failure of service-providing infrastructures affects the most vulnerable individuals of our society most intensely. This emphasizes the role and the importance of functioning health, social and constitutional infrastructures during a slow-onset crisis like an ongoing pandemic.
5.2. “Humans” as a component
Humans are rarely described as a component in a system of critical infrastructures, considering their vulnerabilities, failure, and protection (e.g., effects of failure of irreplaceable experts due to sickness in the water sector; essential workers not showing up to work due to concern of their own family). A study from 2006 on the possible impact of an influenza pandemic and critical infrastructure dependencies on hospitals, addresses the importance of skilled and qualified workers to the functioning of hospitals and other critical infrastructure facilities. If a significant number of workers of critical infrastructures fall ill, the functioning of those infrastructures and subsequently all the other ones connected to them, such as hospitals, are jeopardized [ 52 ]. An additional example is the water networks of Vienna, where operators of the infrastructure are excellently prepared for a wide range of technical failures (e.g., power outages). However, another essential component for the operation of water networks is professionally experienced workers since the water network cannot be operated without trained and experienced professionals. An analysis of the US water sector supports this, by highlighting that the functioning of drinking water utilities relies on multiple trained and specialized workers within water facilities, and also outside them (e.g., chemists, microbiologists, engineers, or public relations staff). These essential workers are crucial for the functioning of the water supply [ 53 ]. In most literature sources describing precautionary and protective measures for critical infrastructure, the measures are aimed at preventing or mitigating technical failure, and before the start of the COVID-19 pandemic the essential role of specialists was rarely mentioned. However, during the pandemic, the term ‘essential worker’ became widely known and much discussed. For example, in August 2021, US Cybersecurity and Infrastructure Security Agency published a guidance document on the safety of essential workers at their workplace. The document lists who qualifies as an essential worker (workers who conduct a range of operations and services that may be essential to continued critical infrastructure operations) per critical infrastructure sector during the pandemic and provides advice for COVID-19 risk management at workplaces [ 51 ]. This example emphasizes the importance of considering the potential effects on an infrastructure if several skilled personnel were to fail at the same time, for whose expertise or professional experience there is no substitute. Considering the risk of a shortage of workers due to illness, Flynn (2020) suggests reaching out to recent retirees and reintegrating them into the workplace for the duration of the pandemic for support as one possible solution [ 50 ]. Overall, the human being as an essential component in a critical infrastructure system needs to be paid more attention to and explored in more detail.
5.3. COVID-19 as paradigm shift
A clear change can be perceived between the focus in the literature on critical infrastructures before the COVID-19 pandemic and the literature focusing on critical infrastructure in connection with COVID-19 since the start of the pandemic. Galbusera et al. (2020) [ 47 ] conducted a study on emergency & business continuity and impacts on critical infrastructures during the COVID-19 pandemic. The results showed that the sectors transportation & traffic, public health, university & research, and public safety & civil protection were strongest affected by the COVID-19 pandemic in March and April 2020. The functioning of institutions and organizations was most affected due to health & hygiene protocols, mobility restrictions, staff unavailability, site & area isolation and working hours limitations [ 47 ]. The survey's results showed that critical infrastructures which were identified by experts as most affected during the COVID-19 pandemic are also the ones identified in this study as intensely discussed in the literature on critical infrastructure dependencies since the start of the COVID-19 pandemic (public health, transportation & traffic, constitutional institutions). One major difference in the findings is the strong impact of the pandemic on university & research facilities, which is not reflected as a topic in the literature sources analyzed. Overall, the comparison between descriptions of critical infrastructure dependencies before the COVID-19 pandemic and since the pandemic's start, revealed differences in the focus on sectors. While pre-COVID-19 pandemic, the main focus was on energy, water, ICT and transportation & traffic, the main focus since the start of the COVID-19 pandemic has been on public health, transportation & traffic, constitutional institutions, and the high dependency of the food sector on other sectors. These results highlight the importance of these three sectors as service providing sectors and the fragility of the food sector during an ongoing crisis like a pandemic.
Further, discussions on new critical infrastructures have emerged during the past year. In multiple countries, the importance of urban green spaces and forests for well-being and health during a pandemic has been highlighted through the COVID-19 pandemic, matching the classification of a critical infrastructure. The availability of social services like childcare services for essential staff with children has proved to be crucial for maintaining workforces and the functioning of sectors. With the increase in working from home, the dependency of many sectors on ICT increased. Though ICT had already been one of the most discussed critical infrastructures prior to the COVID-19 pandemic, its characteristic and way of impacting other sectors has changed. For example, online shopping delivery is strongly linked with ICT and has become an infrastructure providing especially vulnerable individuals with essential goods. These examples highlight the criticality of those infrastructures in maintaining vital societal functions, health, safety, security, and the economic and social well-being of people. A disruption or destruction can have a severe impact on the overall system (e.g., a disruption in child-care services can cause absenteeism of essential workers in other critical infrastructures if parents have no one to look after their children during work hours). These examples further show how situational critical infrastructures emerge due to specific circumstances. It needs to be considered whether to treat those infrastructures as overall new critical infrastructures or recognize them as situational critical infrastructures, becoming critical in specific settings.
What long-lasting impact on the perception of critical infrastructures could the COVID-19 pandemic have? The analysis from this study has shown how, in the context of the COVID-19 pandemic, new topics of critical infrastructures have emerged and have been increasingly discussed. This raises the question of whether this is a short-term phenomenon in the context of the COVID-19 pandemic, which will subside with the end of the pandemic. Or is the COVID-19 pandemic not just a phenomenon but an initiation and has it caused a permanent shift in considerations of criticality and discussions that will be carried on long after the end of the pandemic? Since the COVID-19 pandemic is still ongoing at the time of writing, no final answer can be given to these questions. However, examples from history have shown how severe disasters with a strong impact, which shocked a whole society (e.g., ice storm of 1998 led to improvements in critical infrastructure protection in Canada [ 17 ]; The terrorist attack on the World Trade Center in 2001 caused permanent changes in critical infrastructure protection measures in the USA [ 18 ]) can cause permanent changes in critical infrastructure protection, the effects of which ripple through research and policies. Even though the total impact that the COVID-19 pandemic will have on the understanding of critical infrastructures cannot be anticipated yet, the discussions that have started already show that it has provided an opportunity for an alternate perspective on criticality. Examples from guidance notes on essential workers and workplace safety during the COVID-19 pandemic (e.g., CISA [ 51 ]) show how new considerations are integrated and adapted to existing structures and approaches, serving as an extension rather than a separate, disconnected topic. In addition, the review of critical infrastructure issues discussed in relation to COVID-19 has shown how the impact of the pandemic relates to experience and existing knowledge on criticality. This shows that emerging topics of criticality are treated as a learning experience on critical infrastructure protection, dependencies and failures, and are connected and integrated with existing topics and knowledge rather than creating a new, separate domain and school of thought.
The pandemic has impacted society as a whole. The insights gained through the impact of the recent pandemic have unraveled essential elements and weaknesses of today's system of critical infrastructures and can be leveraged to plan for better infrastructure protection in the future. Due to this first real-world example of the impact of a global pandemic on our modern society, the COVID-19 pandemic has the potential to cause a paradigm shift in the understanding of what critical infrastructures are and what it is that makes critical infrastructures “critical”.
6. Conclusion
The COVID-19 pandemic is an unprecedented example, revealing the dependencies of our system of critical and non-critical infrastructures and how our modern society depends upon the functioning of this system. While infrastructures like water, energy, transportation & traffic, and ICT have always been perceived as highly critical, prioritized for protection measures, and well discussed in the literature, other critical and non-critical infrastructures have been neglected in discussions of what is critical and essential to our society. This study has revealed the following three main insights into the impacts of the COVID-19 pandemic on the perception of critical infrastructures and what is understood as critical:
Often overseen critical infrastructures have been brought into the spotlight through the COVID-19 pandemic, highlighting the importance of infrastructures such as public health, social systems, and constitutional institutions. Services such as child-care, care of the elderly, and distribution systems have become highly critical and essential for the functioning of society during the pandemic. Seeing the effects of a crisis jeopardizing these services, has revealed the high dependency of our society on not only technical infrastructures but also social and other infrastructures.
Infrastructures which were not perceived as critical before the pandemic were revealed to be critical during the pandemic. For example, online shopping and parcel delivery have become critical during the pandemic by providing essential goods to citizens and protecting vulnerable individuals from exposure to the virus during on-site shopping. Another example are urban green spaces and natural recreational areas. During lockdown periods green areas have become essential for the mental and physical well-being of citizens in multiple countries (e.g., urban forests in Germany). In those places, green infrastructure has played a significant role in providing essential services to citizens especially in urban areas, and without this infrastructure, the well-being of people and subsequently the functioning of critical infrastructures, would have been at risk. Consequently, issues arose for local communities in the surroundings of large cities to guide the mass of visitors, recreation-seeking and sporting people and to cope with negative side-effects like increased numbers of cars and road cyclists, and increased human impact on nature areas and wildlife, posing a threat to forest biodiversity [ 46 , 54 ]. In the context of a pandemic, the protection of natural areas therefore becomes more than just natural protection, but also protection of essential service provision to citizens.
The COVID-19 pandemic could be a paradigm shift and act as motivator to reconsider the understanding of criticality and what classifies it. The definition of critical infrastructures by the EU law includes “… health, safety, security, economic or social well-being of peop le..” [ 10 ]. This inclusion of the well-being of citizens highlights its essential role in the functioning of society. Therefore, it is necessary to understand what affects the well-being of citizens and how it can be best ensured and protected. Overall, the COVID-19 pandemic has provided insight, in a way which has not been seen before, into what keeps our society functioning. This insight should act as an impulse to reflect on the values and essentials of our society and how to best protect them.
6.1. Future research
Based on the study conducted, the following topics should be the focus of future research and ideally complemented with opinions end experiences from experts and workers in the different sectors:
- • Critical infrastructures during a slow-onset crisis: What are the effects of a slow-onset and long-lasting crisis like a pandemic on critical infrastructures? Which infrastructures are most affected and how can they be best protected?
- • Humans as a component: What role do humans such as trained workers play in a system of critical infrastructures and what happens if a large number of workers is unable to work?
- • New critical infrastructures: Considering the inclusion of well-being into the classification of critical infrastructures and the experience during the COVID-19 pandemic, which infrastructures classify as “critical” that were not considered critical before? What situational criticalities arise during a pandemic and how is their criticality perceived after the end of a crisis?
Declaration of competing interest
The authors declare that they have no known competing financial interests or personal relationships that could have appeared to influence the work reported in this paper.
Acknowledgements
This work was supported by the research Project ODYSSEUS (“Simulation und Analyze kritischer Netzwerk-Infrastrukturen in Städten”, Simulation and Analysis of Critical Network Infrastructures in Cities) funded by the Austrian Research Promotion Agency (FFG) under Grant No. 873539.
Appendix B Supplementary data to this article can be found online at https://doi.org/10.1016/j.ijdrr.2022.103419 .
Appendix A. Identified literature
The following two tables list the literature sources which were identified and analyzed for the time period before the start of the COVID-19 pandemic (45 sources) and since the start of the COVID-19 period (35 sources).
Appendix BSupplementary data
The following are the Supplementary data to this article:
Data availability
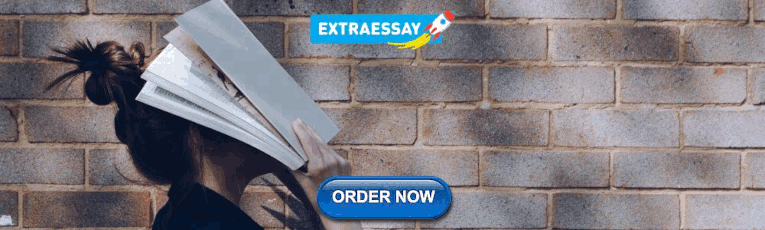
IMAGES
VIDEO
COMMENTS
The bridge would have been considered a critical component of the transportation infrastructure, but the resiliency within the system allowed for a mere traffic disruption rather than a catastrophic failure of the entire system occurring. 70 While sports stadiums and arenas are infrastructure considered critical to the local or regional economy ...
BUSINESS IMPLICATIONS. Critical infrastructure failure/shortfall can have adverse effects that directly impact businesses' operations. Companies may see their operations disrupted due to sudden power outages, while. in the long run, they may face a significant decrease in productivity and increase in costs.
Masahiko Haraguchi and Soojun Kim. 2016. "Critical Infrastructure Systems: A Case Study of the Interconnectedness of Risks Posed by Hurricane Sandy for New York City." International Journal of Disaster Resilience in the Built Environment, 7, 2, Pp. 133-143.
Once generated, the failures can propagate further within a critical infrastructure system and produce negative impacts of different character, intensity, and effect. Rinaldi et al. [ 6] were the first to define the basic types of failure propagation in a critical infrastructure system: A cascading failure occurs when a disruption in one ...
The most industrialized countries are equipped with increasingly extensive and sophisticated infrastructure systems, so-called critical infrastructures (CIs) such as energy distribution networks and transport infrastructures [].Critical infrastructures are, therefore, those material resources, services, information technology systems, networks and infrastructure assets that, if damaged or ...
Summary. Explicitly considering major critical infrastructure disruptions from the perspective of crisis/crisis management enables policymakers, analysts, and researchers to draw inspiration from an extensive multidisciplinary literature. Furthermore, this approach takes infrastructure failures or disruptions, and provides crucial institutional ...
For the case study, we simulated two different scenarios (baseline, and pessimistic) to generate a range of economic loss estimates, which we estimated to be somewhere between PhP 2.8-3.5 billion. ... Analysing critical infrastructure failure with a resilience inoperability input-output model. Economic Systems Research, 26, 39-59. https ...
Abstract At the case study of the city of Cologne and the neighbouring Rhein-Erft-Kreis (a county), selected resilience aspects of critical infrastructure (CI) ... Multiple flood cascading Path 1 (supporting infrastructure failure path): Flood - > bridges - > roads - > rescue cars - > hospital. ...
Assessing the economic ripple effects of critical infrastructure failures using the dynamic inoperability input-output model: a case study of the Taal Volcano ... template and case study results from this paper can provide policy insights to enhance disaster ... by determining probabilities of failure. This step is
We estimate that the economic loss for the case study under consideration is between €46 million and €173 million. ... for assessing the economic impact of critical infrastructure network ...
Aros-Vera, F., Gillian, S., Rehmar, A. & Rehmar, L. Increasing the resilience of critical infrastructure networks through the strategic location of microgrids: a case study of Hurricane Maria in ...
Construction of critical infrastructure chain failure case database The core of this study is to establish a case database containing information about the chain failure of critical infrastructure. The data involved in the case were all reported by public media channels, such as newspapers, magazines and other related publications, as well as ...
Critical infrastructure protection is vital to keep essential services running and often relies on public-private cooperation models. But while failure of critical infrastructure is often considered a worst-case scenario, there is often a question over who pays for its security. Identifying 'systemically important critical infrastructure' could ...
1. Introduction and state of the art. Dependencies of critical infrastructures can be documented, analyzed and investigated in multiple ways. Case studies (e.g., Schneidhofer & Wolthusen (2015) [1]) or incident reports (e.g., Hassanzadeh et al. (2019) [2]) document failures and their effects in real scenarios, while theoretical modelling and simulations (e.g., Rinaldi 2002 [3], Setola 2008 [4 ...
Cascading failure modeling in interdependent critical infrastructure systems (CISs) is an important but challenging work that lays the foundation for disaster impact assessment and recovery planning. However, despite the increasing volume of studies that examine cascading failure across interdependent CISs, the majority of modeling approaches proposed in prior studies do not capture the ...
Interdependencies among critical infrastructure assets increase risk to individual assets and the overall system. These interconnected infrastructure components constitute a "system of systems" where the failure of one or multiple infrastructure elements can cascade and affect the resilience of the entire system and ultimately the region.
the sector. In all case studies analyzed, the design standards of the infrastructure asset, and the ongoing maintenance of assets and the organized response (or lack of) has major implications for the performance of the electricity grid. This paper is a product of the Global Facility for Disaster Reduction and Recovery, Climate Change Group.
Using the developed system the critical infrastructure failure metric is measured, and the propagation of cascading failures in the Chennai area during the flooding event in December 2015 is evaluated, analyzed, and visualized in a spatiotemporal environment. The real-world case study showcases the dire need of the proposed simulation model system.
The purpose of this chapter is to provide a comprehensive overview of a critical infra-. structure system, of failures and impacts that occur within it and of the resilience, which. e ectively ...
The model is developed based on a real-world cascading CI failure case study on an interdependent HCI network during the flood disaster event in December 2015 at Chennai, TamilNadu, India.
The complexity of interacting systems, paired with the increasing dependency, the un-ability to predict the consequences of critical infrastructure breakdowns and increasing institutional fragmentation highlights the society's challenge in proactively addressing risks of infrastructure failure (Cedergren, Johansson, and Hassel Citation 2018 ...
The remainder of the article is organized as follows. A review of the literature on critical infrastructure, risk analysis, and FMEA is presented in Section 2. The research methodology is discussed in Section 3. A real-world case study demonstrating the application of the proposed model is presented in Section 4.
One of the most perilous natural hazards is flooding resulting from dam failure, which can devastate downstream infrastructure and lead to significant human casualties. In recent years, the frequency of flash floods in the northern part of Nicosia, Cyprus, has increased. This area faces increased risk as it lies downstream of the Kanlikoy Dam, an aging earth-fill dam constructed over 70 years ...
Using Netways IAC Mastery service, organizations were able to lower change failure rate by 50 percent on average and recover from incidents 24 times faster than before. Customers are no longer stuck with hardware expansion problems such as disks, storage, electricity, and network switches. With just the push of a button, all these elements can ...
Case studies from the past show the vulnerability due to this high degree of interconnectedness between the critical infrastructure sectors and potentially severe consequences that failure can trigger [, , , ,29]. In this study, based on a preliminary screening and analysis process, 45 literature sources were identified and analyzed for the ...