Thank you for visiting nature.com. You are using a browser version with limited support for CSS. To obtain the best experience, we recommend you use a more up to date browser (or turn off compatibility mode in Internet Explorer). In the meantime, to ensure continued support, we are displaying the site without styles and JavaScript.
- View all journals
- Explore content
- About the journal
- Publish with us
- Sign up for alerts
- Introduction
- Published: 22 April 2015
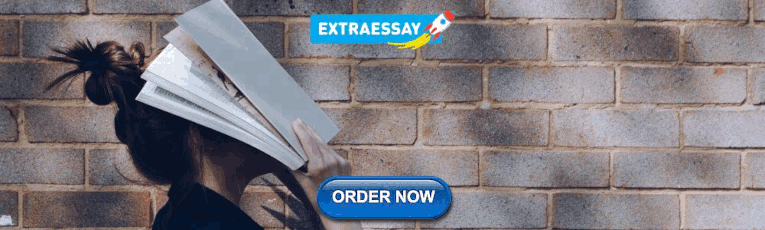
Origin and evolution of vertebrates
- Henry Gee 1
Nature volume 520 , page 449 ( 2015 ) Cite this article
15k Accesses
1 Citations
32 Altmetric
Metrics details
- Developmental biology
- Palaeontology
To celebrate the golden jubilee of On the Origin of Species , in 1909, the Linnean Society of London held a special meeting on a hot biological topic of the day — the origin of the vertebrates. Such was the lack of consensus that one commentator, the zoologist T. R. R. Stebbing, wrote that “the disputants agreed on one single point, namely, that their opponents were all in the wrong.”
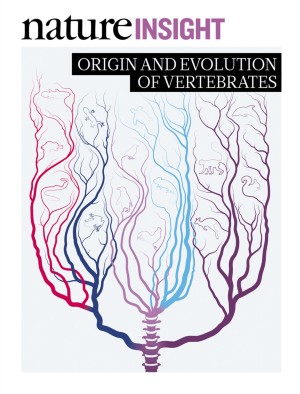
The problem is easily stated — vertebrates have so many special features, from large brains to complex physiologies to unique tissues such as enamel and bone — that their evolution from invertebrates is obscure. The question had intrigued Aristotle, and foxed minds as keen as those of William Bateson and Thomas Hunt Morgan, who, by way of finding a more rewarding problem, went off to discover genetics instead.
The same tools that Bateson and Hunt Morgan helped to create have now returned to address the old problem. Although our understanding is far from complete, it is much better than it was even 20 years ago, and is summarized in this collection of reviews.
Nicholas Holland and colleagues set out how the varied theories advanced to explain vertebrate origins, before Lowe et al . show how they fit in to the deuterostomes, a larger branch of the animal kingdom. Diogo et al . add new perspectives to a central question of vertebrate origins, namely, the origin of the head. Marianne Bronner and colleagues then look at the embryonic tissue known as neural crest, another uniquely vertebrate feature. Philippe Janvier surveys the wealth of newly found, and often curious, fossil evidence, and Martin Brazeau and Matt Friedman chart the evolution of jawed vertebrates from jawless forms. If Stebbing was able to peruse this collection, I hope he would agree that we have come a long way.
Author information
Authors and affiliations.
Senior Editor,
You can also search for this author in PubMed Google Scholar
Rights and permissions
Reprints and permissions
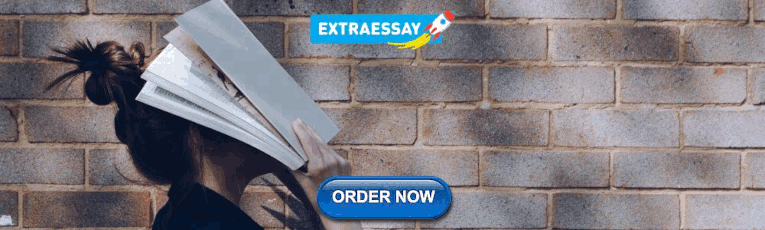
About this article
Cite this article.
Gee, H. Origin and evolution of vertebrates. Nature 520 , 449 (2015). https://doi.org/10.1038/520449a
Download citation
Published : 22 April 2015
Issue Date : 23 April 2015
DOI : https://doi.org/10.1038/520449a
Share this article
Anyone you share the following link with will be able to read this content:
Sorry, a shareable link is not currently available for this article.
Provided by the Springer Nature SharedIt content-sharing initiative
Quick links
- Explore articles by subject
- Guide to authors
- Editorial policies
Sign up for the Nature Briefing newsletter — what matters in science, free to your inbox daily.

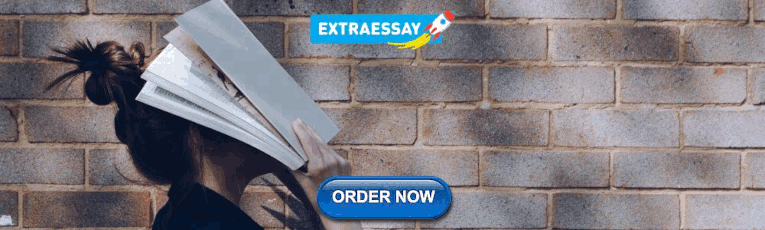