Urban Areas and Air Pollution: Causes, Concerns, and Mitigation
- First Online: 02 December 2023
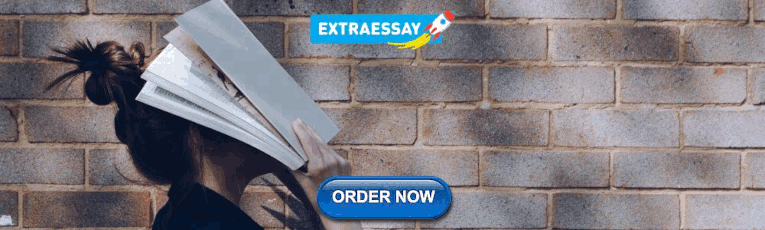
Cite this chapter
- Shivali Gupta 5 &
- Rakesh Kumar ORCID: orcid.org/0000-0003-4139-300X 5
529 Accesses
Urbanization has proven to be a catalyst for global economic growth. However, the concomitant progress in economic development has led to a degradation in air quality within urban settlements, primarily attributable to copious anthropogenic sources of pollutant emissions. Air pollution has numerous negative impacts on the well-being of humans and the environment. This includes the deleterious impacts on climate change as well as the emergence of serious cardiovascular and respiratory diseases. This chapter, therefore, discusses urban air pollution, encompassing the causal factors, associated concerns, and various strategies employed to mitigate its adverse effects. These strategies involve regulatory, technological, and behavioural responses, which are imperative to effectively address the issue of air pollution. Therefore, the examination of the complex interplay between urbanization across varying stages of development and air pollution is integral in attaining ambient air quality targets with respect to upcoming economic advancement and sustainable progression.
This is a preview of subscription content, log in via an institution to check access.
Access this chapter
Subscribe and save.
- Get 10 units per month
- Download Article/Chapter or eBook
- 1 Unit = 1 Article or 1 Chapter
- Cancel anytime
- Available as PDF
- Read on any device
- Instant download
- Own it forever
- Available as EPUB and PDF
- Durable hardcover edition
- Dispatched in 3 to 5 business days
- Free shipping worldwide - see info
Tax calculation will be finalised at checkout
Purchases are for personal use only
Institutional subscriptions
Similar content being viewed by others
Urban Air Pollution
How can urban policies improve air quality and help mitigate global climate change: a systematic mapping review.
Urban Air Pollution and Health in Developing Countries
Agarwal, P., Sarkar, M., Chakraborty, B., & Banerjee, T. (2019). Phytoremediation of air pollutants. In Phytomanagement of polluted sites: Market opportunities in sustainable phytoremediation . Elsevier Inc. https://doi.org/10.1016/B978-0-12-813912-7.00007-7
Chapter Google Scholar
Ahmad, S. S., Urooj, R., & Nawaz, M. (2015). Air pollution monitoring and prediction. In Current air quality issues . Intech.
Google Scholar
Alissa, E. M., & Ferns, G. A. (2011). Heavy metal poisoning and cardiovascular disease. Journal of Toxicology, 2011 , 870125.
Article Google Scholar
Ashmore, M. (2013). Air Pollution., 1 , 136–147. https://doi.org/10.1016/B978-0-12-384719-5.00283-5
Banerjee, T., & Srivastava, R. K. (2011). Evaluation of environmental impacts of Integrated Industrial Estate-Pantnagar through application of air and water quality indices. Environmental Monitoring and Assessment, 172 (1–4), 547–560. https://doi.org/10.1007/s10661-010-1353-3
Banerjee, T., Murari, V., Kumar, M., & Raju, M. P. (2015). Source apportionment of airborne particulates through receptor modeling: Indian scenario. Atmospheric Research, 164–165 , 167–187. https://doi.org/10.1016/j.atmosres.2015.04.017
Article CAS Google Scholar
Barwise, Y., & Kumar, P. (2020). Designing vegetation barriers for urban air pollution abatement: A practical review for appropriate plant species selection. Climate and Atmospheric Science, 3 (1), 12.
Beaulant, A. L., Perron, G., Kleinpeter, J., Weber, C., Ranchin, T., & Wald, L. (2008). Adding virtual measuring stations to a network for urban air pollution mapping. Environment International, 34 (5), 599–605.
Bechle, M. J. (2011). Effects of income and urban form on urban {NO}. Environmental Science & Technology, 45 (11), 4914–4919.
Bikis, A., & Pandey, D. (2021). Air quality at public transportation stations/stops: Contribution of light rail transit to reduce air pollution. Aerosol Science and Engineering, 6 , 1–16.
Bozyazi, E., Incecik, S., Mannaerts, C., & Brussel, M. (2000). Analysis and mapping of air pollution using a GIS approach: A case study of Istanbul. WIT Transactions on Ecology and the Environment, 42 . https://doi.org/10.2495/AIR000431
Cariolet, J. M., Colombert, M., Vuillet, M., & Diab, Y. (2018). Assessing the resilience of urban areas to traffic-related air pollution: Application in Greater Paris. Science of the Total Environment, 615 , 588–596.
Chen, H., Deng, G., & Liu, Y. (2022). Monitoring the influence of industrialization and urbanization on spatiotemporal variations of AQI and PM2.5 in three provinces, China. Atmosphere, 13 (9). https://doi.org/10.3390/atmos13091377
Cheng, W. C., Liu, C. H., & Leung, D. Y. (2009). On the correlation of air and pollutant exchange for street canyons in combined wind-buoyancy-driven flow. Atmospheric Environment, 43 (24), 3682–3690.
Cope, W. G., Leidy, R. B., & Hodgson, E. (2004). Classes of toxicants: Use classes. In A textbook of modern toxicology (pp. 49–74).
Eleni, D., Dessinioti, C., & Christina, V. A. (2014). Air pollution and the skin. Frontiers in Environmental Science, 2 , 11.
Ferrante, M., Fiore, M., Copat, C., Morina, S., Ledda, C., Mauceri, C., & Conti, G. O. (2015). Air pollution in high-risk sites–Risk analysis and health impact. Current Air Quality Issues . 419–442. https://doi.org/10.5772/60345
Fino, A. (2018). Air quality legislation. In Encyclopedia of environmental health (2nd ed.). Elsevier Inc. https://doi.org/10.1016/B978-0-12-409548-9.11045-0
Fortoul-van der Goes, T., Rodriguez-Lara, V., Gonzalez-Villalva, A., Colin-Barenque, L., Rojas-Lemus, M., Bizarro-Nevares, P., et al. (2015). Health effects of metals in particulate matter. In Current air quality issues .
Ghosh, S., & Maji, T. (2011). An environmental assessment of urban drainage, sewage and solid waste management in Barddhaman municipality, West Bengal. International Journal of Environmental Sciences, 2 (1), 92–104. http://www.ipublishing.co.in/jesvol1no12010/volumetwo/EIJES3010.pdf
CAS Google Scholar
Glaeser, E. L., & Kahn, M. E. (2003). Handbook (2003) urban sprawl. In The handbook of regional and urban economics, IV (pp. 2481–2528).
Haque, M. S., & Singh, R. B. (2017). Air pollution and human health in Kolkata, India: A case study. Climate, 5 (4), 1–16. https://doi.org/10.3390/cli5040077
He, C., Morawska, L., Hitchins, J., & Gilbert, D. (2004). Contribution from indoor sources to particle number and mass concentrations in residential houses. Atmospheric Environment , 38 (21), 3405–3415. https://doi.org/10.1016/j.atmosenv.2004.03.027
Heal, M. R., Heaviside, C., Doherty, R. M., Vieno, M., Stevenson, D. S., & Vardoulakis, S. (2013). Health burdens of surface ozone in the UK for a range of future scenarios. Environment International, 61 , 36–44.
Ho, B. Q. (2012). Urban air pollution. In Air pollution monitoring, modelling and health (pp. 1–38). InTech.
Kamińska, I. A., Ołdak, A., & Turski, W. A. (2004). Geographical Information System (GIS) as a tool for monitoring and analysing pesticide pollution and its impact on public health. Annals of Agricultural and Environmental Medicine, 11 (2), 181–184.
Komar, I., & Lalić, B. (2015). Sea transport air pollution. In Current air quality issues (pp. 165–202). InTech.
Kumar, B., & Singh, R. B. (2003). Urban development and anthropogenic climate change: Experience in Indian metropolitan cities . Manak Publication Pvt Ltd..
Kumar, M., Singh, R. K., Murari, V., Singh, A. K., Singh, R. S., & Banerjee, T. (2016). Fireworks induced particle pollution: A spatiotemporal analysis. Atmospheric Research, 180 (June), 78–91. https://doi.org/10.1016/j.atmosres.2016.05.014
Leung, D. Y. C. (2015). Outdoor-indoor air pollution in urban environment: Challenges and opportunity. Frontiers in Environmental Science, 2 (JAN), 1–7. https://doi.org/10.3389/fenvs.2014.00069
Li, X. X., Liu, C. H., & Leung, D. Y. C. (2009). Numerical investigation of pollutant transport characteristics inside deep urban street canyons. Atmospheric Environment, 43 (15), 2410–2418. https://doi.org/10.1016/j.atmosenv.2009.02.022
Liang, L., & Gong, P. (2020). Urban and air pollution: A multicity study of long-term effects of urban landscape patterns on air quality trends. Scientific Reports, 10 (1), 1–13. https://doi.org/10.1038/s41598-020-74524-9
Manisalidis, I., Stavropoulou, E., & Stavropoulos, A. (2020). Environmental and Health Impacts of Air Pollution: A Review., 8 (February), 1–13. https://doi.org/10.3389/fpubh.2020.00014
Martínez-Bravo, M., & Martínez-del-Río, J. (2019). Urban pollution and emission reduction. Sustainable Cities and Communities. Encyclopedia of the UN Sustainable Development Goals , 1–11. https://doi.org/10.1007/978-3-319-71061-7_30-1
Memon, R. A., Leung, D. Y., & Liu, C. H. (2009). An investigation of urban heat island intensity (UHII) as an indicator of urban heating. Atmospheric Research , 94 (3), 491–500. https://doi.org/10.1016/j.atmosres.2009.07.006
Mhawish, A., Banerjee, T., Broday, D. M., Misra, A., & Tripathi, S. N. (2017). Evaluation of MODIS Collection 6 aerosol retrieval algorithms over Indo-Gangetic Plain: Implications of aerosols types and mass loading. Remote Sensing of Environment, 201 (March), 297–313. https://doi.org/10.1016/j.rse.2017.09.016
Milner, J., Harpham, C., Taylor, J., Davies, M., Le Quéré, C., Haines, A., & Wilkinson, P. (2019). The challenge of urban heat exposure under climate change: An analysis of cities in the Sustainable Healthy Urban Environments (SHUE) database. Climate (Basel, Switzerland), 5 (4), 93.
Mitchell, D., Heaviside, C., Vardoulakis, S., Huntingford, C., & Masato, G. (2016). Attributing human mortality during extreme heat waves to anthropogenic climate change. Environmental Research Letters, 11 , 074006.
Mo, Z., Fu, Q., Lyu, D., Zhang, L., Qin, Z., Tang, Q., et al. (2019). Impacts of air pollution on dry eye disease among residents in Hangzhou, China: A case-crossover study. Environmental Pollution, 246 , 183–189.
Pandey, M., Singh, V., Vaishya, R. C., & Shukla, A. K. (2013). Analysis & application of GIS based air quality monitoring- state of art. International Journal of Engineering Research and Technology, 2 (12), 3788–3796.
Piracha, A., & Chaudhary, M. T. (2022). Urban air pollution, urban heat island and human health: A review of the literature. Sustainability (Switzerland), 14 (15). https://doi.org/10.3390/su14159234
Ramanathan, V., & Carmichael, G. (2008). Global and regional climate changes due to black carbon. Nature Geoscience, 1 (4), 221–227. https://doi.org/10.1038/ngeo156
Rodríguez, M. C., Dupont-Courtade, L., & Oueslati, W. (2016). Air pollution and urban structure linkages: evidence from European cities. Renewable and Sustainable Energy Reviews, 53 , 1–9. https://doi.org/10.1016/j.rser.2015.07.190
Rosário Filho, N. A., Urrutia-Pereira, M., d'Amato, G., Cecchi, L., Ansotegui, I. J., Galán, C., et al. (2021). Air pollution and indoor settings. World Allergy Organization Journal, 14 (1), 100499.
Salmond, J., Sabel, C. E., & Vardoulakis, S. (2018). Towards the integrated study of urban climate, air pollution, and public health. Climate, 6 (1), 14.
Shendell, D. G. (2019). Community outdoor air quality: Sources, exposure agents and health. In Encyclopedia of environmental health (Vol. 1, Issue April, 2nd ed.). Elsevier. https://doi.org/10.1016/B978-0-12-409548-9.11824-X
Singh, R. B., Haque, S., & Grover, A. (1972). Drinking water, sanitation and health in kolkata metropolitan city: Contribution towards urban. Geography Environment Sustainability, 8 (4), 64–81.
Sofia, D., Gioiella, F., Lotrecchiano, N., & Giuliano, A. (2020). Mitigation strategies for reducing air pollution. Environmental Science and Pollution Research, 27 (16), 19226–19235. https://doi.org/10.1007/s11356-020-08647-x
Sówka, I., Badura, M., Pawnuk, M., Szymański, P., & Batog, P. (2020). The use of the GIS tools in the analysis of air quality on the selected University campus in Poland. Archives of Environmental Protection, 46 (1), 100–106. https://doi.org/10.24425/aep.2020.132531
Strosnider, H., Kennedy, C., Monti, M., & Yip, F. (2017). Rural and urban differences in air quality, 2008-2012, and community drinking water quality, 2010-2015 - United States. MMWR Surveillance Summaries, 66 (13), 2010–2015. https://doi.org/10.15585/mmwr.ss6613a1
Tecer, L. H., & Tagil, S. (2013). Spatial-temporal variations of sulfur dioxide concentration, source, and probability assessment using a GIS-based geostatistical approach. Polish Journal of Environmental Studies, 22 (November 2016), 1491–1498.
UNEP, UNICEF, & WHO. (2002). Children in the new millennium: Environmental impact on health . World Health Organization.
Ung, A., Wald, L., Ranchin, T., Groupe, T., De Paris, M., Antipolis, S., Weber, C., Hirsch, J., Image, L., & Pasteur, U. L. (2002). Satellite data for air pollution mapping over a city – Virtual stations . In Observing our environment from space - new solutions for a new millennium (pp. 147–151). CRC Press.
Vaddiraju, S. C. (2020). Mapping of air pollution using GIS: A case study of Hyderabad . June 2019 . https://doi.org/10.32622/ijrat.752019328
Vallero, D. (2014a). Air pollutant hazards. In Fundamentals of air pollution (pp. 197–214). https://doi.org/10.1016/b978-0-12-401733-7.00007-4
Vallero, D. (2014b). Air pollutant kinetics and equilibrium. In Fundamentals of air pollution (pp. 437–474).
Vallero, D. (2014c). Respiratory effects of air pollutants. In Fundamentals of air pollution (pp. 247–256).
Wang, Q. (2018). Urbanization and global health: The role of air pollution. Iranian Journal of Public Health, 47 (11), 1644–1652.
Wang, S., Gao, S., Li, S., & Feng, K. (2020). Strategizing the relation between urbanization and air pollution: Empirical evidence from global countries. Journal of Cleaner Production, 243 , 118615. https://doi.org/10.1016/j.jclepro.2019.118615
Wargocki, P., Wyon, D. P., Sundell, J., Clausen, G., & Fanger, O. (2000). The effects of outdoor air supply rate in an office on perceived air quality, sick building syndrome (SBS) symptoms and productivity. Indoor Air, 10 (4), 222–236. https://doi.org/10.1034/j.1600-0668.2000.010004222.x
Weisskopf, M. G., Kioumourtzoglou, M. A., & Roberts, A. L. (2015). Air pollution and autism spectrum disorders: Causal or confounded? Current Environmental Health Reports, 2 , 430–439.
Weyens, N., Thijs, S., Popek, R., Witters, N., & Przybysz, A. (2015). The role of plant – microbe interactions and their exploitation for phytoremediation of air pollutants (pp. 25576–25604). https://doi.org/10.3390/ijms161025576
Book Google Scholar
WHO global air quality guidelines: Particulate matter (PM2.5 and PM10), ozone, nitrogen dioxide, sulfur dioxide and carbon monoxide [Internet]. Geneva: World Health Organization; 2021. Table 3.26, Recommended 2021 AQG levels and 2005 air quality guidelines. Available from: https://www.ncbi.nlm.nih.gov/books/NBK574591/table/ch3.tab 26/
World Bank. (2022). https://www.worldbank.org/en/topic/urbandevelopment/overview
Download references
Conflict of Interest
The authors have no conflicts of interest.
Author information
Authors and affiliations.
Department of Environmental Sciences, University of Jammu, JKUT, Jammu, India
Shivali Gupta & Rakesh Kumar
You can also search for this author in PubMed Google Scholar
Editor information
Editors and affiliations.
Department of Ecology, Environment and Remote Sensing, Government of Jammu and Kashmir, Srinagar, Jammu and Kashmir, India
Fayma Mushtaq
Majid Farooq
LeadsConnect Services Pvt Ltd, Noida, Uttar Pradesh, India
Alok Bhushan Mukherjee
Birla Institute of Technology, Mesra, Ranchi, Jharkhand, India
Mili Ghosh Nee Lala
Rights and permissions
Reprints and permissions
Copyright information
© 2023 The Author(s), under exclusive license to Springer Nature Switzerland AG
About this chapter
Gupta, S., Kumar, R. (2023). Urban Areas and Air Pollution: Causes, Concerns, and Mitigation. In: Mushtaq, F., Farooq, M., Mukherjee, A.B., Ghosh Nee Lala, M. (eds) Geospatial Analytics for Environmental Pollution Modeling. Springer, Cham. https://doi.org/10.1007/978-3-031-45300-7_7
Download citation
DOI : https://doi.org/10.1007/978-3-031-45300-7_7
Published : 02 December 2023
Publisher Name : Springer, Cham
Print ISBN : 978-3-031-45299-4
Online ISBN : 978-3-031-45300-7
eBook Packages : Earth and Environmental Science Earth and Environmental Science (R0)
Share this chapter
Anyone you share the following link with will be able to read this content:
Sorry, a shareable link is not currently available for this article.
Provided by the Springer Nature SharedIt content-sharing initiative
- Publish with us
Policies and ethics
- Find a journal
- Track your research
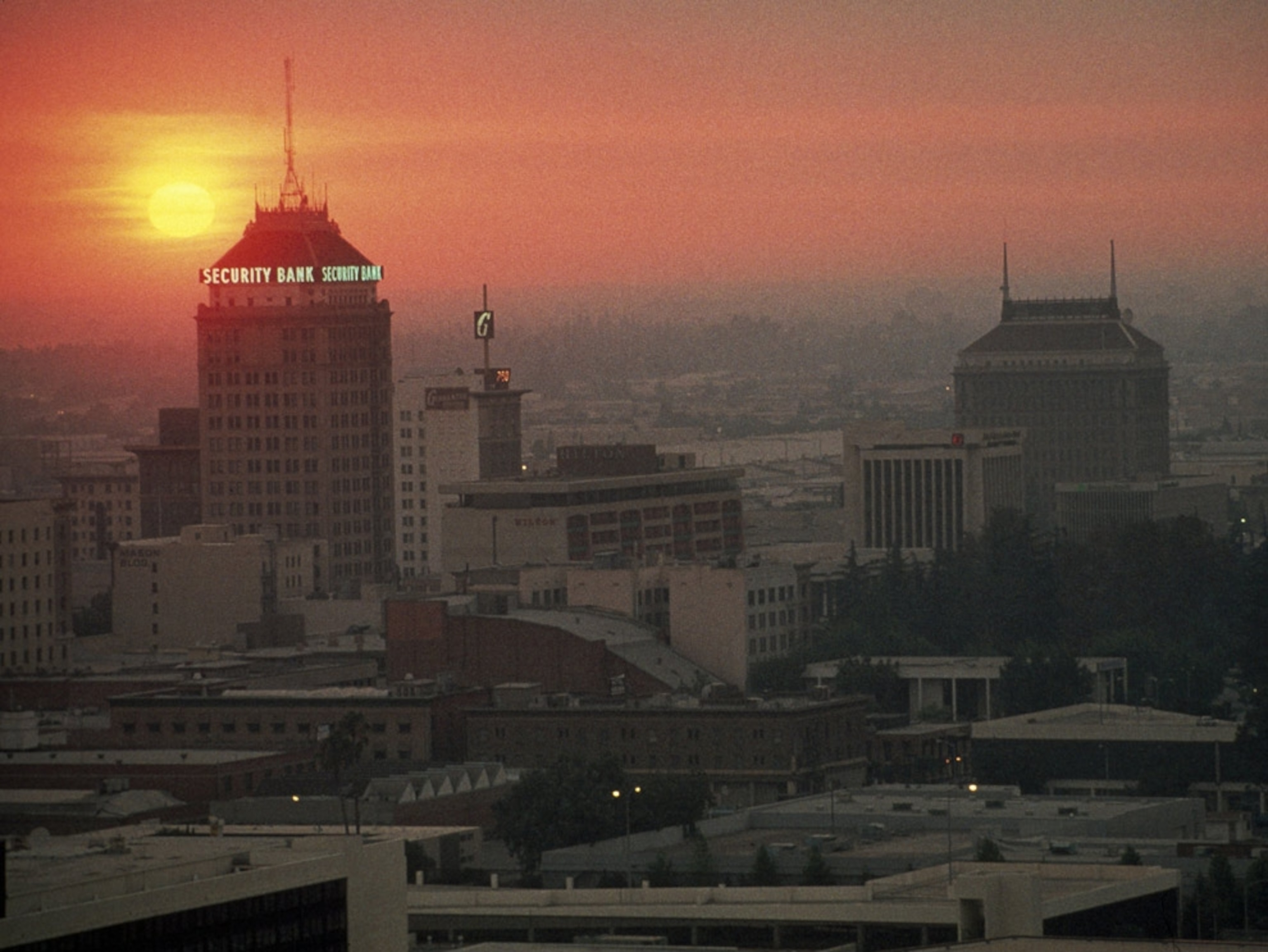
A cloak of smog gives Fresno, California, a hazy look. Smog, a hybrid of the words "smoke" and "fog," is caused when sunlight reacts with airborne pollution, including ash, dust, and ground-level ozone.
Urban Threats
Urbanization spurs a unique set of issues to both humans and animals.
The promise of jobs and prosperity, among other factors, pulls people to cities. Half of the global population already lives in cities, and by 2050 two-thirds of the world's people are expected to live in urban areas. But in cities two of the most pressing problems facing the world today also come together: poverty and environmental degradation.
Poor air and water quality, insufficient water availability, waste-disposal problems, and high energy consumption are exacerbated by the increasing population density and demands of urban environments. Strong city planning will be essential in managing these and other difficulties as the world's urban areas swell.
- Intensive urban growth can lead to greater poverty, with local governments unable to provide services for all people.
- Concentrated energy use leads to greater air pollution with significant impact on human health.
- Automobile exhaust produces elevated lead levels in urban air.
- Large volumes of uncollected waste create multiple health hazards.
- Urban development can magnify the risk of environmental hazards such as flash flooding .
- Pollution and physical barriers to root growth promote loss of urban tree cover.
- Animal populations are inhibited by toxic substances, vehicles, and the loss of habitat and food sources.
- Combat poverty by promoting economic development and job creation.
- Involve local community in local government.
- Reduce air pollution by upgrading energy use and alternative transport systems.
- Create private-public partnerships to provide services such as waste disposal and housing.
- Plant trees and incorporate the care of city green spaces as a key element in urban planning.
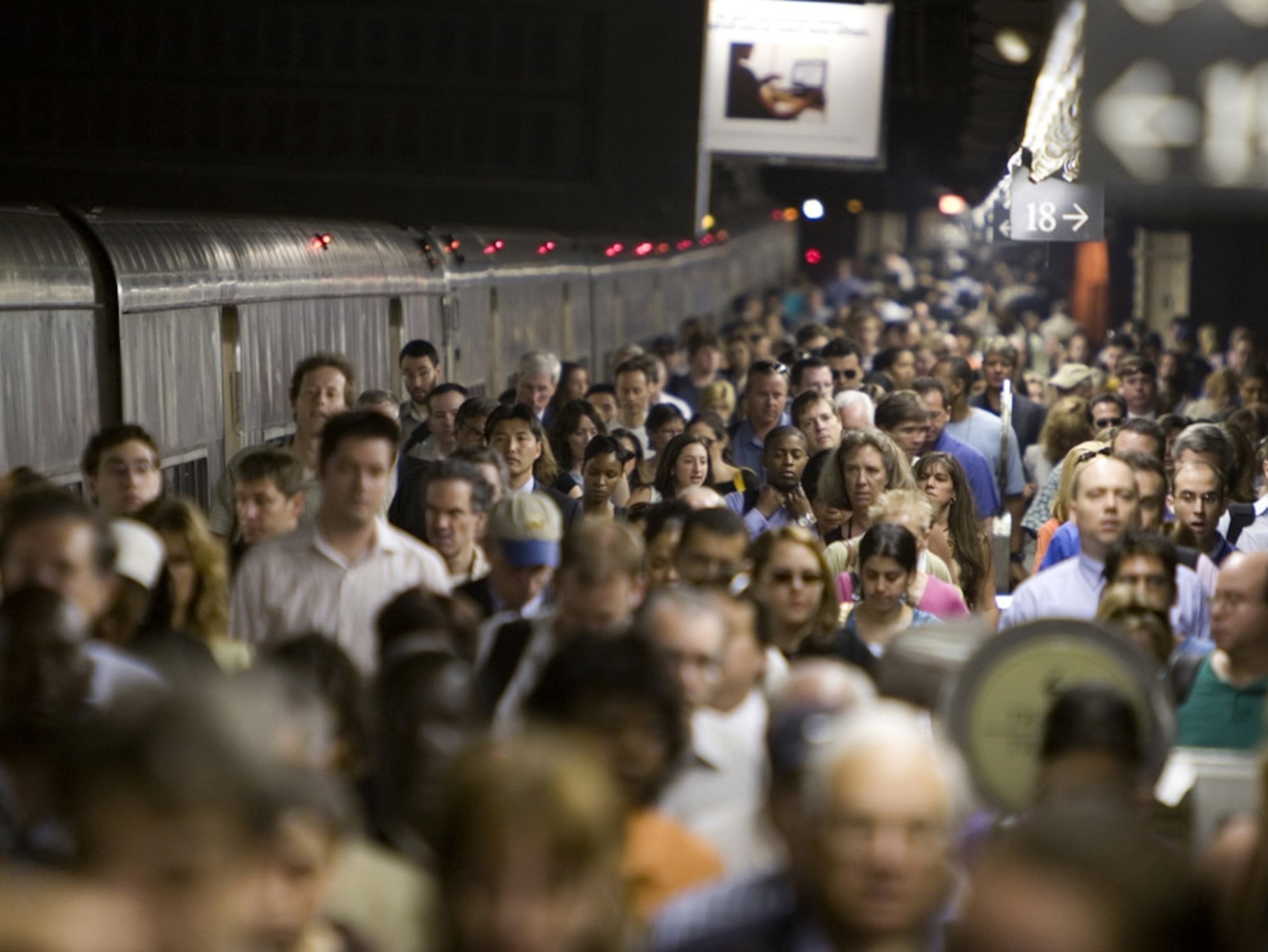
Related Topics
- ENVIRONMENT AND CONSERVATION
- AIR POLLUTION
- URBAN PLANNING
- WASTE MANAGEMENT
You May Also Like
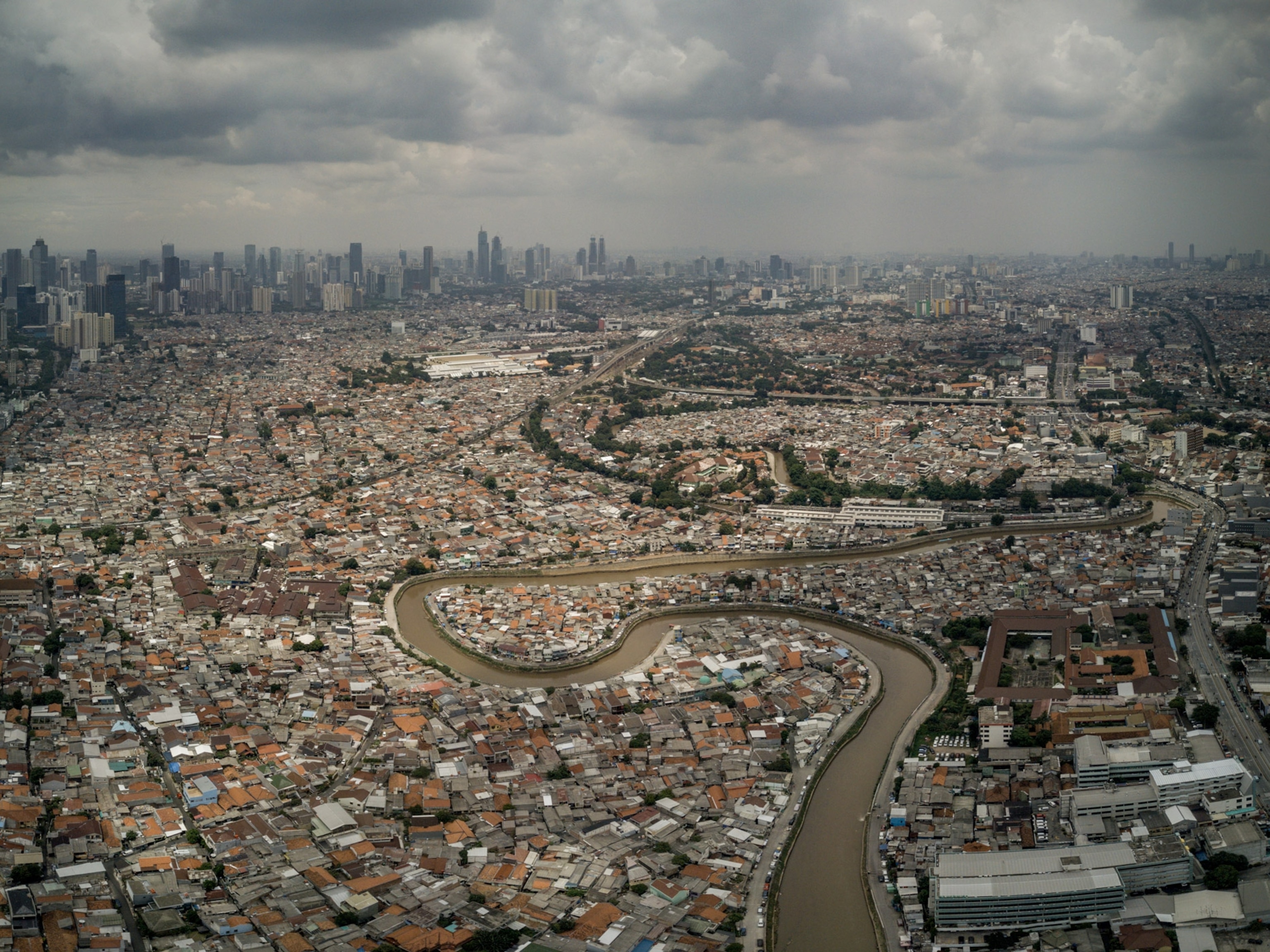
Indonesia's giant capital city is sinking. Can the government's plan save it?
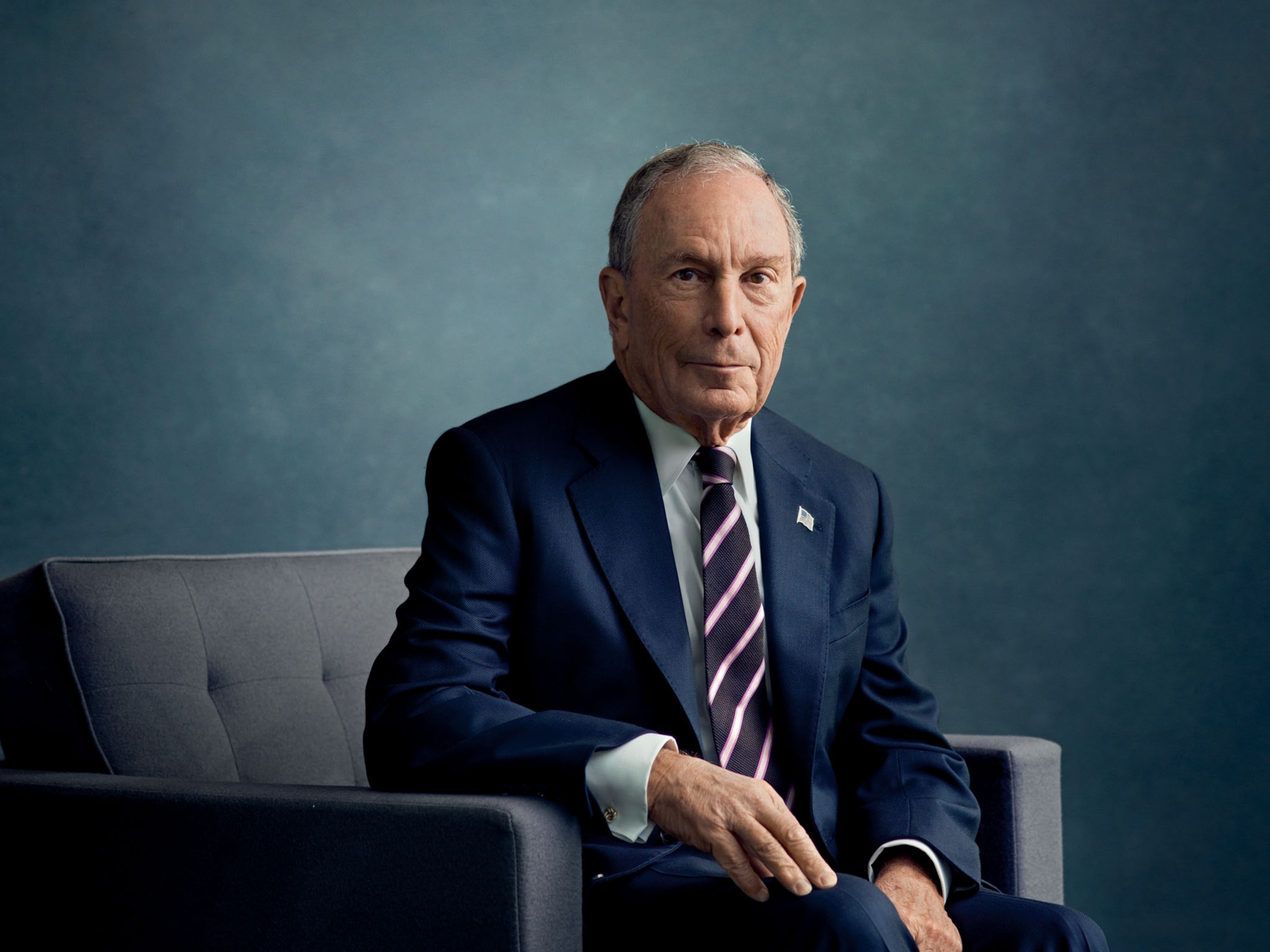
‘Cities, Businesses, and Citizens Can Save the Planet’
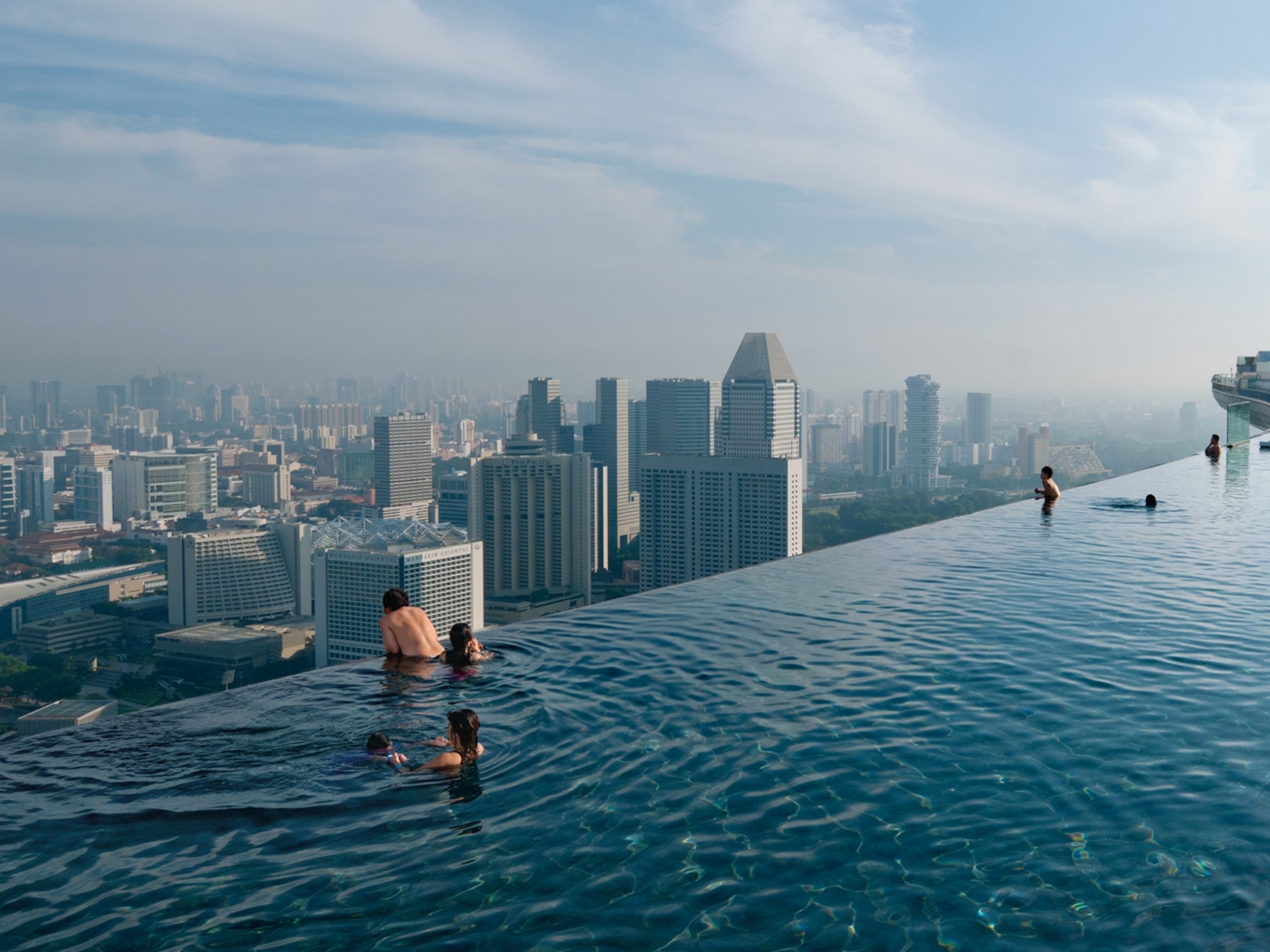
The City Solution
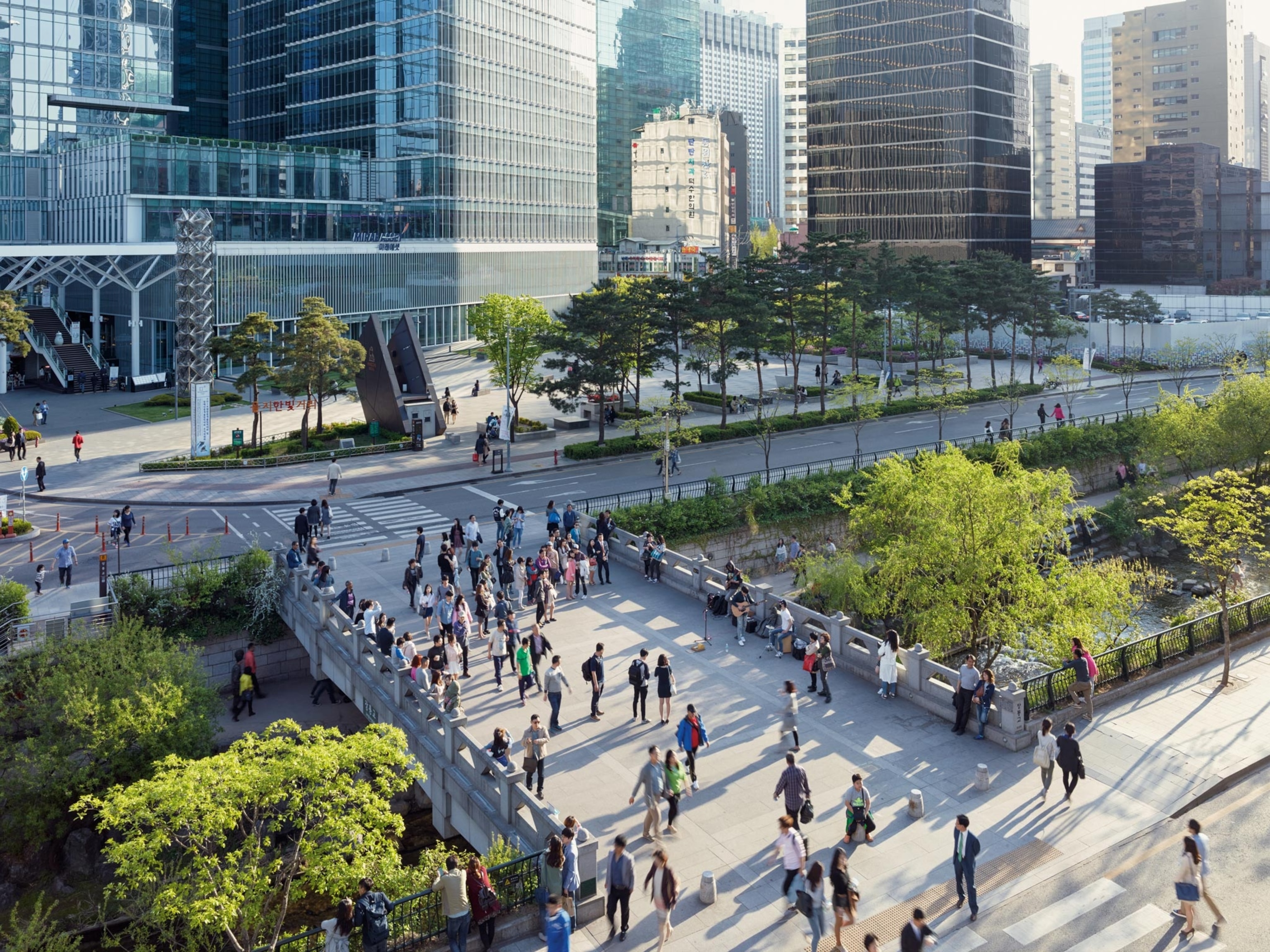
How Urban Parks Are Bringing Nature Close to Home
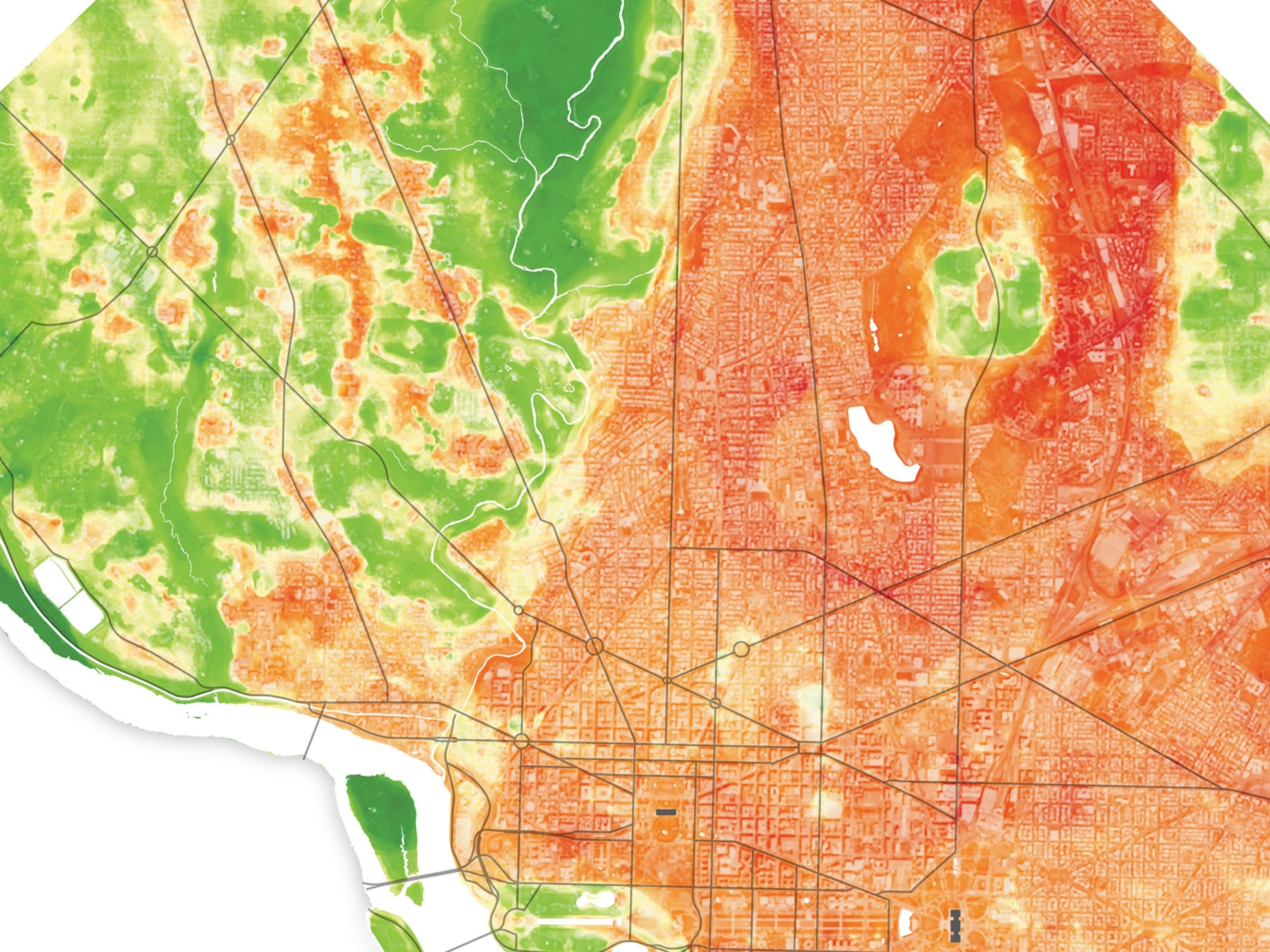
To prepare for rising temperatures, scientists map urban ‘hot spots’
- Terms of Use
- Privacy Policy
- Your US State Privacy Rights
- Children's Online Privacy Policy
- Interest-Based Ads
- About Nielsen Measurement
- Do Not Sell or Share My Personal Information
- Nat Geo Home
- Attend a Live Event
- Book a Trip
- Inspire Your Kids
- Shop Nat Geo
- Visit the D.C. Museum
- Learn About Our Impact
- Support Our Mission
- Advertise With Us
- Customer Service
- Renew Subscription
- Manage Your Subscription
- Work at Nat Geo
- Sign Up for Our Newsletters
- Contribute to Protect the Planet
Copyright © 1996-2015 National Geographic Society Copyright © 2015-2024 National Geographic Partners, LLC. All rights reserved
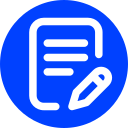
Essay Curve
Essay on Pollution Due To Urbanization

Essay on Pollution Due To Urbanization: Urbanization has brought about numerous benefits such as economic growth and improved living standards. However, it has also led to a significant increase in pollution levels in urban areas. This essay will explore the various forms of pollution that have arisen as a result of urbanization, including air, water, and noise pollution. It will also discuss the impacts of this pollution on human health and the environment, as well as potential solutions to mitigate its effects.
Table of Contents
Pollution Due To Urbanization Essay Writing Tips
1. Introduction: Start your essay by defining urbanization and its impact on the environment. Explain how rapid urbanization has led to increased pollution levels in cities.
2. Discuss the sources of pollution: Identify the main sources of pollution in urban areas such as vehicular emissions, industrial activities, construction work, and waste disposal. Explain how these activities contribute to air, water, and soil pollution.
3. Effects of pollution: Describe the harmful effects of pollution on human health and the environment. Discuss how air pollution can lead to respiratory diseases, water pollution can contaminate drinking water sources, and soil pollution can affect agricultural productivity.
4. Impact on wildlife: Explain how pollution due to urbanization can also have a negative impact on wildlife. Discuss how habitat destruction, chemical pollution, and noise pollution can disrupt ecosystems and threaten biodiversity.
5. Solutions to reduce pollution: Propose practical solutions to reduce pollution in urban areas. This can include promoting public transportation, implementing stricter emission standards for vehicles and industries, enforcing waste management regulations, and promoting green spaces in cities.
6. Role of government and individuals: Highlight the importance of government policies and regulations in addressing pollution due to urbanization. Discuss how individuals can also contribute to reducing pollution by adopting sustainable practices such as recycling, conserving energy, and using eco-friendly products.
7. Conclusion: Summarize the key points discussed in the essay and emphasize the need for collective action to combat pollution due to urbanization. Encourage readers to take responsibility for their actions and work towards creating a cleaner and healthier environment for future generations.
8. Proofread and revise: Before submitting your essay, make sure to proofread it for any grammatical or spelling errors. Revise the content to ensure that your arguments are clear and well-supported with evidence and examples.
By following these writing tips, you can effectively write an essay on pollution due to urbanization that is informative, engaging, and persuasive.
Essay on Pollution Due To Urbanization in 10 Lines – Examples
1. Urbanization leads to increased pollution as more people move to cities, resulting in higher levels of traffic, industrial activities, and waste generation. 2. The burning of fossil fuels for transportation and energy production in urban areas contributes to air pollution, leading to respiratory problems and other health issues. 3. Urbanization also leads to deforestation and loss of green spaces, which can result in soil erosion, water pollution, and habitat destruction. 4. The construction of buildings and infrastructure in urban areas can disrupt natural ecosystems and contribute to pollution through the release of construction materials and chemicals. 5. Urbanization increases the demand for water resources, leading to over-extraction of groundwater and contamination of water sources with pollutants. 6. The concentration of people in urban areas results in higher levels of waste generation, leading to problems with waste management and disposal, including littering and illegal dumping. 7. Urbanization can also lead to noise pollution from increased traffic, construction activities, and other sources, affecting the quality of life for residents. 8. The expansion of urban areas can result in the destruction of agricultural land and natural habitats, leading to loss of biodiversity and ecosystem services. 9. Urbanization can exacerbate the effects of climate change through increased greenhouse gas emissions, heat island effects, and other factors. 10. Addressing pollution due to urbanization requires sustainable urban planning, investment in green infrastructure, and policies to promote cleaner transportation and energy sources.
Sample Essay on Pollution Due To Urbanization in 100-180 Words
Urbanization has led to a significant increase in pollution levels in cities. The rapid growth of urban areas has resulted in higher levels of air, water, and noise pollution. The increase in vehicles, industries, and construction activities has contributed to the degradation of the environment.
Air pollution is a major concern in urban areas, with emissions from vehicles and industries leading to the formation of harmful pollutants such as carbon monoxide, sulfur dioxide, and particulate matter. Water pollution is also a problem, as urban runoff carries pollutants into rivers and lakes, contaminating water sources. Noise pollution from traffic and construction activities can have negative effects on human health and well-being.
To combat pollution due to urbanization, it is essential to implement strict regulations on emissions and waste disposal. Encouraging the use of public transportation, promoting green spaces, and investing in renewable energy sources can also help reduce pollution levels in urban areas. Ultimately, it is crucial to prioritize sustainable development practices to mitigate the harmful effects of urbanization on the environment.
Short Essay on Pollution Due To Urbanization in 200-500 Words
Urbanization is the process of population concentration in urban areas, leading to the growth of cities and towns. This rapid urbanization has resulted in numerous environmental challenges, with pollution being one of the most significant issues. Pollution due to urbanization has become a major concern as cities continue to expand and develop.
One of the main sources of pollution in urban areas is air pollution. The increase in vehicular traffic, industrial activities, and construction projects in urban areas has led to a significant rise in air pollution levels. The emission of harmful gases and particulate matter from vehicles and industries has resulted in poor air quality, leading to respiratory problems and other health issues for the residents of these areas. Additionally, the burning of fossil fuels for energy production and heating further contributes to air pollution in urban areas.
Water pollution is another major consequence of urbanization. The discharge of untreated sewage and industrial waste into rivers and water bodies has contaminated water sources, making them unfit for consumption. The rapid urbanization has also led to the encroachment and destruction of wetlands and natural water bodies, further exacerbating the problem of water pollution. The presence of pollutants in water bodies not only affects aquatic life but also poses a serious health risk to humans who rely on these water sources for drinking and other purposes.
Noise pollution is another issue that arises due to urbanization. The increase in population density and urban activities has led to higher levels of noise pollution in cities. Traffic noise, construction activities, and industrial operations contribute to the overall noise levels in urban areas, leading to stress, sleep disturbances, and other health problems for residents. Noise pollution can also have a negative impact on wildlife, disrupting their natural habitats and communication patterns.
Urbanization also contributes to the generation of solid waste in cities. The increase in population and consumption patterns has led to a significant rise in the amount of waste generated in urban areas. Improper waste management practices, such as open dumping and burning of waste, further exacerbate the problem of solid waste pollution in cities. The accumulation of waste in landfills and open spaces not only degrades the environment but also poses a threat to public health.
In conclusion, pollution due to urbanization is a pressing issue that requires immediate attention and action. Sustainable urban planning and development practices, along with stringent environmental regulations, are essential to mitigate the adverse effects of pollution in urban areas. It is crucial for governments, policymakers, and residents to work together to address the challenges posed by urbanization and ensure a cleaner and healthier environment for future generations.
Essay on Pollution Due To Urbanization in 1000-1500 Words
Urbanization is the process of population concentration in cities and towns, leading to the growth of urban areas. It is a global phenomenon that has been occurring at an unprecedented rate in recent decades. While urbanization has brought about numerous benefits such as economic growth, improved infrastructure, and access to better services, it has also led to a range of environmental problems, with pollution being one of the most significant issues.
Pollution due to urbanization is a complex and multifaceted problem that affects both the natural environment and human health. The rapid expansion of cities and towns has resulted in increased levels of air, water, and soil pollution, as well as noise pollution. These pollutants have a range of negative impacts on the environment, including damage to ecosystems, loss of biodiversity, and climate change. In addition, pollution poses serious health risks to urban residents, leading to respiratory diseases, cardiovascular problems, and other health issues.
One of the main sources of pollution due to urbanization is air pollution. The burning of fossil fuels for energy production, transportation, and industrial activities releases a range of pollutants into the atmosphere, including carbon dioxide, nitrogen oxides, sulfur dioxide, and particulate matter. These pollutants can have a range of negative impacts on human health, including respiratory problems, cardiovascular diseases, and even premature death. In addition, air pollution can also damage ecosystems, reduce crop yields, and contribute to climate change.
Water pollution is another significant issue associated with urbanization. The discharge of untreated sewage, industrial effluents, and agricultural runoff into rivers, lakes, and oceans can contaminate water sources and degrade water quality. This pollution can have serious consequences for aquatic ecosystems, leading to the loss of biodiversity, fish kills, and the destruction of coral reefs. In addition, water pollution can also pose risks to human health, as contaminated water sources can transmit diseases such as cholera, typhoid, and hepatitis.
Soil pollution is also a concern in urban areas, as the disposal of solid waste, industrial chemicals, and agricultural pesticides can contaminate soil and degrade soil quality. This pollution can have negative impacts on agricultural productivity, as contaminated soil may not be suitable for growing crops. In addition, soil pollution can also pose risks to human health, as contaminated soil can transfer pollutants to food crops, leading to the ingestion of harmful chemicals.
Noise pollution is another issue associated with urbanization, as the growth of cities and towns has led to increased levels of noise from traffic, construction, and industrial activities. This noise pollution can have negative impacts on human health, leading to stress, sleep disturbances, and hearing loss. In addition, noise pollution can also disrupt wildlife habitats, leading to changes in animal behavior and population dynamics.
In addition to these specific forms of pollution, urbanization can also contribute to broader environmental problems such as deforestation, habitat destruction, and climate change. The expansion of cities and towns often involves the clearing of forests and other natural habitats, leading to the loss of biodiversity and ecosystem services. In addition, urban areas are major sources of greenhouse gas emissions, contributing to global warming and climate change.
Addressing pollution due to urbanization requires a range of strategies at the local, national, and global levels. One key approach is to promote sustainable urban planning and development practices that prioritize environmental protection and public health. This can include measures such as promoting public transportation, improving waste management systems, and implementing green building standards. In addition, governments can also regulate industrial emissions, enforce pollution control laws, and invest in clean energy technologies to reduce pollution levels.
Public awareness and education are also crucial in addressing pollution due to urbanization. By raising awareness about the impacts of pollution on the environment and human health, individuals can take steps to reduce their own environmental footprint and advocate for policies that promote sustainability. In addition, education programs can also help to build public support for pollution control measures and encourage behavior change towards more sustainable lifestyles.
In conclusion, pollution due to urbanization is a significant environmental problem that poses serious risks to the environment and human health. The rapid expansion of cities and towns has led to increased levels of air, water, and soil pollution, as well as noise pollution, with a range of negative impacts on ecosystems, biodiversity, and public health. Addressing pollution due to urbanization requires a range of strategies, including sustainable urban planning, pollution control measures, and public awareness campaigns. By taking action to reduce pollution levels and promote environmental protection, we can create healthier and more sustainable urban environments for current and future generations.
Related Essays
Essay on A Visit To A Fair – 10 Lines, 100 to 1500 Words
Value of Games And Sports – Essay in 10 Lines, 100 to 1500 Words
Essay on Importance of Teacher – 100, 200, 500, 1000 Words
Essay on A Visit To A Museum – 100, 200, 500, 1000 Words
Essay on Effect of Social Media On Youth
Essay on Shri Guru Nanak Dev Ji – Short & Long Essay Examples
Essay on Nuclear Family – Short Essay & Long Essay upto 1500 Words
Essay on Anudeep Durishetty – 10 Lines, 100 to 1500 Words
Essay on Non Violence – Samples, 10 Lines to 1500 Words
Covid 19 Responsive School – Essay in 10 Lines, 100 to 1500 Words
Leave a Comment Cancel reply
Save my name, email, and website in this browser for the next time I comment.
Thank you for visiting nature.com. You are using a browser version with limited support for CSS. To obtain the best experience, we recommend you use a more up to date browser (or turn off compatibility mode in Internet Explorer). In the meantime, to ensure continued support, we are displaying the site without styles and JavaScript.
- View all journals
- Explore content
- About the journal
- Publish with us
- Sign up for alerts
- Open access
- Published: 27 April 2021
Urbanization: an increasing source of multiple pollutants to rivers in the 21st century
- Maryna Strokal ORCID: orcid.org/0000-0002-8063-7743 1 ,
- Zhaohai Bai ORCID: orcid.org/0000-0001-7685-5441 2 ,
- Wietse Franssen 1 ,
- Nynke Hofstra 1 ,
- Albert A. Koelmans 3 ,
- Fulco Ludwig 1 ,
- Lin Ma ORCID: orcid.org/0000-0003-1761-0158 2 ,
- Peter van Puijenbroek ORCID: orcid.org/0000-0001-6370-2411 4 ,
- J. Emiel Spanier 1 ,
- Lucie C. Vermeulen ORCID: orcid.org/0000-0002-8403-2442 5 ,
- Michelle T. H. van Vliet ORCID: orcid.org/0000-0002-2597-8422 6 ,
- Jikke van Wijnen 7 &
- Carolien Kroeze 1
npj Urban Sustainability volume 1 , Article number: 24 ( 2021 ) Cite this article
21k Accesses
66 Altmetric
Metrics details
- Element cycles
- Science, technology and society
Most of the global population will live in urban areas in the 21st century. We study impacts of urbanization on future river pollution taking a multi-pollutant approach. We quantify combined point-source inputs of nutrients, microplastics, a chemical (triclosan) and a pathogen ( Cryptosporidium ) to 10,226 rivers in 2010, 2050 and 2100, and show how pollutants are related. Our scenarios consider socio-economic developments and varying rates of urbanization and wastewater treatment. Today, river pollution in Europe, South-East Asia and North America is severe. In the future, around 80% of the global population is projected to live in sub-basins with multi-pollutant problems in our high urbanization scenarios. In Africa, future river pollution is projected to be 11–18 times higher than in 2010, making it difficult to meet Sustainable Development Goals. Avoiding future pollution is technically possible with advanced wastewater treatment in many regions. In Africa, however, clean water availability is projected to remain challenging. Our multi-pollutant approach could support effective water pollution assessment in urban areas.
Similar content being viewed by others
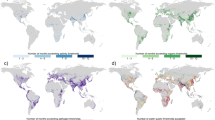
Current wastewater treatment targets are insufficient to protect surface water quality
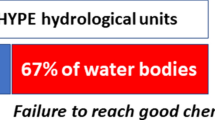
Chemical pollution imposes limitations to the ecological status of European surface waters
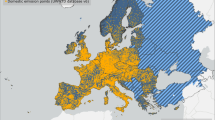
Domestic waste emissions to European waters in the 2010s
Introduction.
Urban areas currently accommodate more than half of the global population 1 and generate over two-thirds of the world gross domestic products (GDP) 2 , 3 . In 2050, more than two-thirds of the global population will live in cities 1 , 4 , 5 . Rapid urbanization creates opportunities for economic developments 6 , but may also increase the use of freshwater resources 4 , 6 , 7 , 8 , 9 . This will increase competition for water between cities and agriculture 4 . More urban waste is likely to result in contamination of water with multiple pollutants such as nutrients 10 and pathogens 11 , 12 from human excretion, plastics 13 , 14 , 15 , 16 , 17 , 18 , and chemicals 19 , 20 from personal care products. River pollution poses a threat to the availability of clean water in large parts of the world 7 , 21 , challenging the achievement of Sustainable Development Goal 6 (SDG, clean water for all) and 11 (sustainable cities). Recent studies on impacts of rapid urbanization on water stress or water scarcity worldwide exist 4 , but often ignore water quality 7 .
Previous global studies likely underestimate the impact of urbanization on water pollution because of their strong focus on single pollutants 10 , 16 , 20 , 22 , 23 , 24 (Fig. 1 ). Urbanization (e.g., sewer connections in cities) is, however, often a common, point source of multiple pollutants in rivers, contributing to multiple impacts. Examples are eutrophication problems caused by nitrogen (N) and phosphorus (P) in many world regions 25 , 26 , and diarrhea caused by pathogens (e.g., Cryptosporidium ) especially in developing countries 11 , 27 . A multi-pollutant approach is, thus, urgently needed to account for interactions between drivers of urbanization (e.g., population, economy) and pressures such as emissions of different pollutants 21 . This can help to identify effective solutions accounting for synergies and trade-offs in pollution control. Furthermore, reducing multiple pollutants in rivers from urban-related sources might be easier (e.g., improved wastewater treatment) than from diffuse sources such as agricultural runoff (e.g., delay effects of reduction options due to accumulation of substances in soils). This may have a positive effect on the overall water quality status depending on diffuse sources.
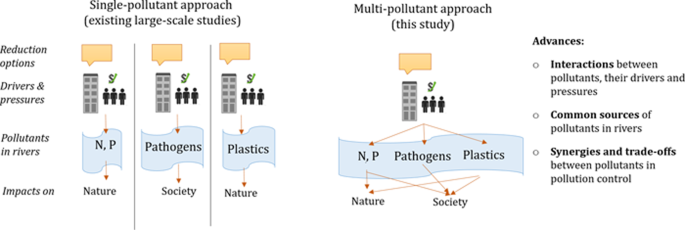
The figure shows a difference between single-pollutant approaches (most existing studies) and a multi-pollutant approach (this study) to assess the impacts of the rapid urbanization on future global river quality. We take N (nitrogen), P (phosphorus), pathogens and plastics as examples. Advances of the multi-pollutant approach are discussed in the main text.
In this paper, we study the impacts of urbanization on river pollution in the 21st century, taking a multi-pollutant perspective. We define multi-pollutant problems as increasing levels of more than one pollutant to rivers in future decades. We analyze, simultaneously, the following groups of pollutants: nutrients (N and P), pathogens (such as Cryptosporidium ), microplastics and chemicals (such as triclosan). These pollutants are selected because of their increasing pollution in many rivers worldwide 18 , 20 , 23 , 28 , 29 , 30 . Yet, these pollutants have common urban sources such as sewer systems (worldwide) and open defecation. We quantify point-source inputs of the pollutants to 10,226 rivers for 2010, 2050 and 2100 associated with urbanization: sewer systems and open defecation. For this, we use a global model of Strokal et al. 31 that takes the sub-basin scale modelling approach of Strokal et al. 32 for nutrients and integrates modelling approaches for other pollutants 18 , 20 , 23 (Supplementary Tables 1 , 2 and 3 ). We develop this model further for multiple-pollutants and future analyses based on evaluated, modelling approaches (see the “Methods” section).
To assess the impacts of urbanization, we develop five scenarios with different levels of urbanization and wastewater treatment rates (Fig. 2 ). The storylines are interpretations of the five Shared Socio-economic Pathways (SSPs) 33 , 34 , 35 , 36 (Supplementary Tables 4 , 5 and 6 ). These SSPs are five pathways with different levels of socio-economic challenges for mitigation and adaptation 33 , 34 , 35 , 36 . SSP1 is a Green Road pathway with low socio-economic challenges (e.g., low population growth), but with high economic and urbanization development. It is largely oriented towards achieving sustainable goals (see Supplementary Tables 4 , 5 and 6 ). SSP2 is a middle of the road pathway with medium challenges to mitigation and adaptation. Future trends will not be very different from historical trends. SSP3 is a Rocky Road pathway with high challenges to mitigation and adaptation. It is a world with difficulties to control the population growth and has low economic and urbanization development (see Supplementary Tables 4 , 5 and 6 ). SSP4 is a Road Divided pathway with high challenges to mitigation and low to adaptation. It has a large gap between urban and rural development with the high urbanization rates especially in urban areas. SSP5 is a taking the highway pathway with high challenges to mitigate, but low challenges to adapt. It is a word with priorities towards economy (see Supplementary Tables 4 , 5 and 6 ).
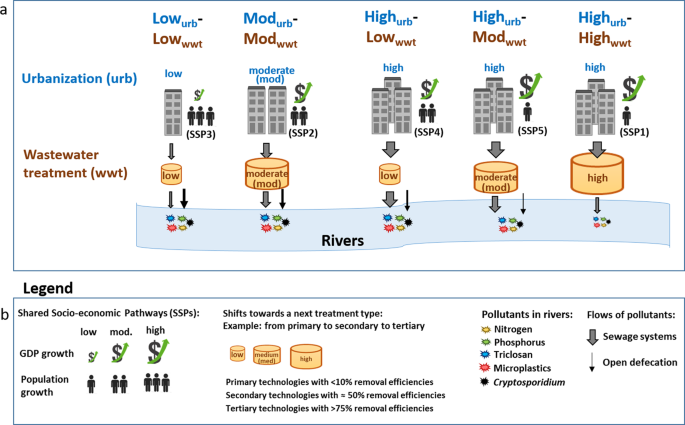
Low, moderate and high urbanization is defined here as the increasing number of urban people and total people with sewer connections (see a and b panels and Supplementary Tables 4 – 6 ). The number of people opens defecating directly to water is assumed to decrease with sewer connection. Higher sewer connections imply that more wastewater treatment plants will be constructed to maintain the increasing volumes of the waste (see the “Methods” section). Low, moderate and high wastewater treatment levels refer here to a shirt (low, moderate, high) towards a next treatment type: e.g., from primary to secondary to tertiary ( a , b , Supplementary Tables 4 – 6 ). This implies the low, moderate and high ambitions to improve wastewater treatment ( b ). Future years are 2050 and 2100. Supplementary Tables 1 – 6 give quantitative interpretations of the storylines for our multi-pollutant model (see also the “Methods” section). GDP is the gross domestic product. Sources for the technologies are in the main text and in Supplementary Table 3 .
Our five scenarios incorporate socio-economic pathways of SSPs, but with quantitative interpretations of aspects related to urbanization and wastewater treatment (see the “Methods” section). Our scenarios aim to show the impact of urbanization on multiple pollutants in rivers. Thus, the names of our five scenarios correspond to the different levels of urbanization and wastewater treatment: from low urbanization and low wastewater treatment rates towards high urbanization and high wastewater treatment rates. This results in the following scenarios: low urbanization and low wastewater treatment rates (Low urb –Low wwt , based on SSP3), moderate urbanization and moderate wastewater treatment rates (Mod urb –Mod wwt , based on SSP2), high urbanization and low wastewater treatment rates (High urb –Low wwt , based on SSP4), high urbanization and moderate wastewater treatment rates (High urb –Mod wwt , based on SSP5), and high urbanization and high wastewater treatment rates (High urb –High wwt , based on SSP1) (Fig. 2 ). The five scenarios consider interactions between global change (socio-economic pathways), urbanization, sanitation and wastewater treatment.
Low, moderate and high urbanization reflect different levels of increases in urban population, and, indirectly, people with sewer connections between 2010 and future years (see the “Methods” section). As a net effect, the number of people practicing open defecation (direct inputs of human waste to rivers) may decrease. Increasing sewer connections assume higher capacities of treatment plants to manage increasing volumes of the wastewater. Low, moderate and high rates of wastewater treatment are defined based on a shift towards a next treatment type: e.g., from primary (technologies with <10% removal rates 10 , 18 , 20 , low) to secondary (50% removal rates 10 , 18 , 20 , 37 , moderate) or to tertiary (>75% removal rates 10 , 38 , 39 , 40 , 41 , 42 , 43 , 44 , high, see the “Methods” section). The differences between the Low urb –Low wwt, and High urb –Low wwt scenarios indicate the impact of urbanization in terms of increasing numbers of people with sewer connections with low ambitions to improve the wastewater treatment under different socio-economic developments. The Mod urb –Mod wwt scenario could be considered business as usual. The differences between the High urb –Low wwt , High urb –Mod wwt and High urb –High wwt scenarios indicate the impact of improving the wastewater treatment in highly urbanized areas. Details are given in the “Methods” section on qualitative and quantitative descriptions of the five urbanization scenarios.
River pollution today
River pollution in Europe, South-East Asia and North America is already severe today. For these regions, we calculate high inputs of N (>50 kg km −2 year −1 ), P (>30 kg km −2 year −1 ), triclosan (>10 g km −2 year −1 ), microplastics (>5 kg km −2 year −1 ) and Cryptosporidium (>100 × 10 17 oocysts km −2 year −1 ) to many rivers in 2010 (Fig. 3 ). These regions experience severe water pollution problems 9 , 16 , 21 , 25 , 45 , contributing to negative impacts 21 such as eutrophication 45 and waterborne diseases (South-East Asian countries). For African sub-basins, pollution levels are not as high as in those regions (Fig. 3 ). However, some impacts of polluted water on children’s health are already indicated 21 . Globally, 9.5 Tg of N, 1.6 Tg of P, 0.45 Tg of microplastics, 0.72 kton of triclosan and 1.6 × 10 17 oocysts of Cryptosporidium entered rivers in 2010 (Fig. 4 , Supplementary Table 7 ). More than half of these inputs are to rivers in South-East Asia. Most of the pollutants in rivers are from sewer systems (see details in Supplementary Figs. from 1 to 29 ). Exceptions are some sub-basins in Africa and South-East Asia where open defecation contributes to over 20% of N, P and Cryptosporidium to their rivers. Existing assessments 9 , 10 , 13 , 20 , 23 reveal similar global estimates, but with diverse spatial scales. Our consistent spatial and temporal scales increase the robustness of our comparisons between multiple pollutants worldwide (e.g., Fig. 4 ).
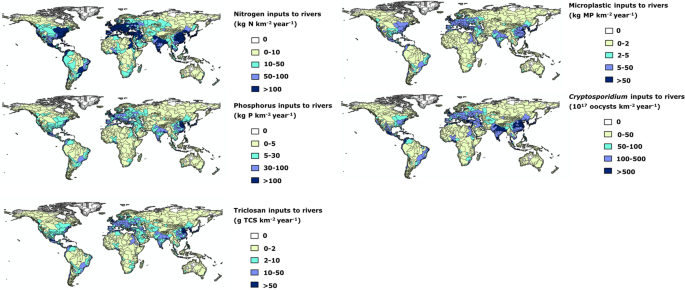
Units are kg km −2 of sub-basin area year −1 for nitrogen (N), phosphorus (P) and microplastics (MP), g km −2 of sub-basin area year −1 for triclosan (TCS) and 10 17 oocysts km −2 of sub-basin area year −1 for Cryptosporidium . Source: the global multi-pollutant model (model description is provided in the “Methods” section, and in Supplementary Tables 1 – 6 , model inputs are in Supplementary Figs. 1 – 14 ). Model uncertainties are discussed in the “Methods” section.
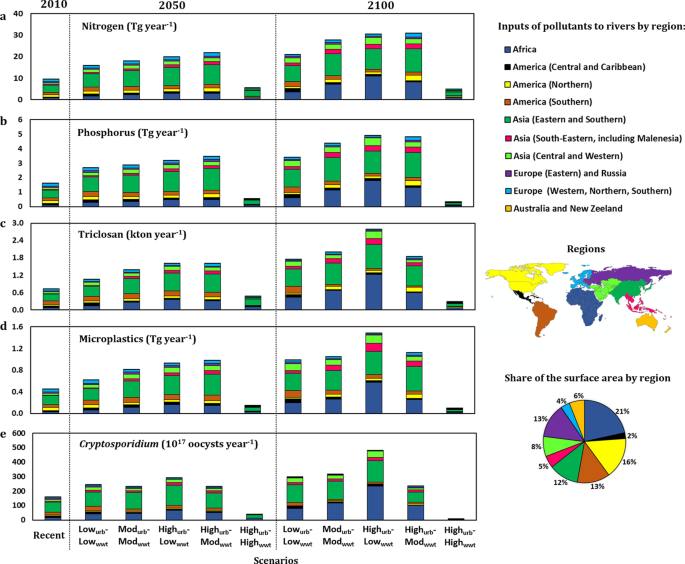
a – e Future trends for individual pollutants. Pies show the shares of the surface areas by region as % of the global surface area. Spatially explicit results are shown in Fig. 3 for 2010 and Fig. 5 for the future. The description of the scenarios is in Fig. 2 , in the “Methods” section and Supplementary Tables 1 – 6 . Source: the global multi-pollutant model (model description is provided in the “Methods” section, and in Supplementary Tables 1 – 6 , model inputs are in Supplementary Figs. 1 – 14 ). Model uncertainties are discussed in the “Methods” section.
High pollution levels result from the net effect of population densities, sewer connection rates (Supplementary Figs. 1 , 2 and 3 ), production of pollutants in human waste (Supplementary Figs. 4 , 5 , 6 , 7 and 8 for individual pollutants) and wastewater treatment efficiencies (Supplementary Figs. 9 , 10 , 11 , 12 and 13 for individual pollutants) in countries (Supplementary Figs. 14 and 15 ). For South-East Asia, high pollution levels are driven by high population densities (Supplementary Figs. 3 and 16 ). This region accommodates approximately half of the global population (3 billion people, Supplementary Fig. 1 ) on 12% of the global surface area (Fig. 4 ). For comparison, sub-basins of Europe (excluding Russia) and North America accommodate around 10% of the global population (0.8 billion people, Supplementary Fig. 1 ) on 20% of the global surface area (Fig. 4 ). Approximately 20% of the total population in 2010 was connected to sewer systems (Supplementary Fig. 1 ) with relatively low wastewater treatment efficiencies (removal levels <50% for most pollutants, Supplementary Figs. 9 – 13 ). For Europe and North America, the high pollution levels per km 2 of sub-basins are driven by high connection rates to sewer systems especially in urban areas. Here, over two-thirds of the population live in urban areas and are largely connected to sewer systems with removal efficiencies above 50% for the studied pollutants (Supplementary Figs. 9 – 13 ). Supplementary Fig. 17 shows the results of the sensitivity analysis indicating the importance of wastewater treatment and human development in river pollution (see the “Discussion” section).
Future river pollution globally
In the future, ~80% of the global population is projected to live in sub-basins with multi-pollutant problems (Figs. 5 and 6 ). These sub-basins cover over half of the global surface area (Fig. 6 ) for which inputs of more than one pollutant will increase at least 30% (Fig. 5 ) between 2010 and 2050 or 2100. This is for all scenarios, except for High urb –High wwt . In the scenario assuming low urbanization and low wastewater treatment (Low urb –Low wwt ), global inputs of most pollutants will less than double between 2010 and 2050 (Fig. 4 ). In this scenario, the population growth is high, and almost doubles between 2010 and 2100 (Supplementary Fig. 3 ). Approximately one-third of the total population globally will be connected to sewer systems. This number is much lower than in the other scenarios in 2100 (Supplementary Fig. 3 ). As a net effect of the low sewer connection (Supplementary Fig. 3 ) and low wastewater treatment (Supplementary Figs. 9 – 13 ), future inputs of pollutants to rivers from sewage are lower in the Low urb –Low wwt scenario than in the others (Fig. 3 ). However, as a trade-off, more nutrients and Cryptosporidium are projected to enter rivers from open defecation, mainly in developing countries (see Supplementary Figs. 14 and 15 ) compared to the other scenarios.
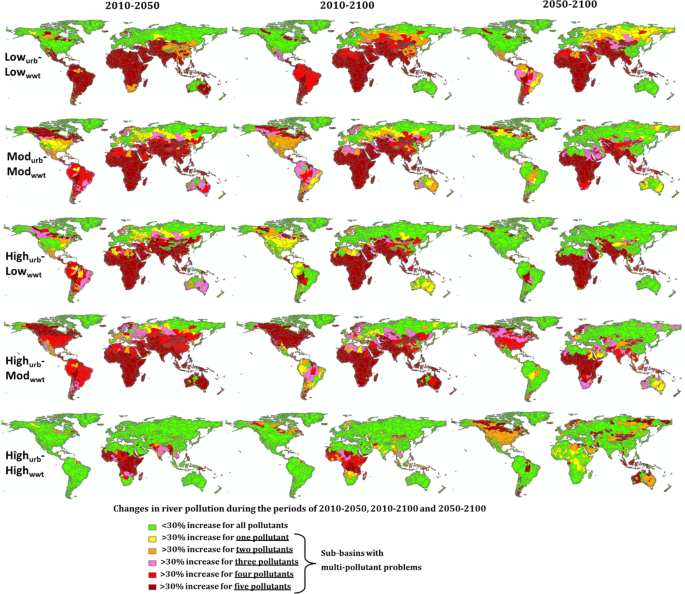
Maps show changes in inputs of pollutants to rivers during the periods of 2010–2050, 2010–2100 and 2050–2100 according to the five scenarios. We classify sub-basins based on the number of pollutants for which the increases are higher or lower than 30% (Note: 30% is arbitrary; see Supplementary Figs. 18 and 20 for results based on 10 and 50% thresholds). The pollutants include Cryptosporidium , microplastic, triclosan, nitrogen and phosphorus. More information is available in Supplementary Figs. 18 – 29 . The description of the five scenarios is in Fig. 2 , in the “Methods” section and Supplementary Tables 1 – 6 . Results for 2010 are in Fig. 3 . Source: the global multi-pollutant model (model description is provided in the “Methods” section, and in Supplementary Tables 1 – 6 , model inputs are in Supplementary Figs. 1 – 14 ). Model uncertainties are discussed in the “Methods” section.
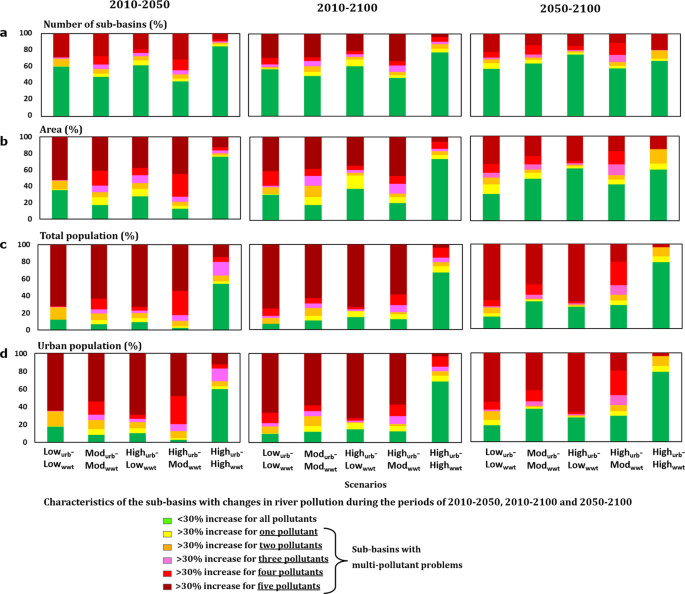
Sub-basins are classified based on the number of pollutants for which the increases are higher or lower than 30% during the periods of 2010–2050, 2010–2100 and 2050–2100 according to the five scenarios. Graphs show the number of sub-basins ( a ), sub-basin areas ( b ), total population ( c ) and urban population ( d ) for the sub-basins with the increases of higher or lower than 30% (Note: 30% is arbitrary; see Supplementary Figs. 19 and 21 for results based on 10% and 50% thresholds). More information is available in Supplementary Figs. 18 – 29 . See Fig. 5 for the changes in inputs of pollutants during the periods of 2010–2050, 2010–2100 and 2050–2100. The description of the scenarios is in Fig. 2 , in the “Methods” section and Supplementary Tables 1 – 6 . Results for 2010 are in Fig. 3 . Source: the global multi-pollutant model (model description is provided in the “Methods” section, and in Supplementary Tables 1 – 6 , model inputs are in Supplementary Figs. 1 – 14 ). Model uncertainties are discussed in the “Methods” section.
The future inputs of most pollutants to rivers are projected to be higher in the scenarios with moderate (Mod urb –Mod wwt ) and high urbanization (High urb –Low wwt , High urb –Mod wwt , Fig. 4 ). The population grows not as fast as in the Low urb –Low wwt scenario, but the rate of urbanization is much higher, especially in the High urb –Low wwt and High urb –Mod wwt scenarios (Supplementary Tables 4 – 6 ). As a result, over two-thirds of the global population is projected to be connected to sewer systems in 2100 (Supplementary Fig. 3 ). Wastewater treatment efficiency is slightly improved (Mod urb –Mod wwt , High urb –Mod wwt ) depending on the economic development (Supplementary Figs. 9 – 13 ). As a net effect, the High urb –Low wwt and High urb –Mod wwt scenarios project, generally, higher inputs of most pollutants to rivers than the Low urb –Low wwt and Mod urb –Mod wwt scenarios (Fig. 4 ).
Pollutants differ in their future trends. For example, High urb –Low wwt projects the highest inputs of Cryptosporidium , microplastics and triclosan globally in 2100 compared to the other pollutants and scenarios (Fig. 4 ). For N and P, High urb -Low wwt and High urb -Mod wwt project somewhat similar amounts globally (Fig. 4 ). All these differences between pollutants and scenarios are a net effect of three important factors: socio-economic development (e.g., population, GDP), urbanization rates (population connected to sewer systems) and treatment efficiencies. For example, higher GDP results generally in higher N and P excretion rates per capita because of changes towards protein-rich diets 31 , 46 (Supplementary Figs. 4 – 5 ). Developed countries (Human Developing Index, HDI > 0.785) have generally lower infection rates, leading to less per capita excretion of Cryptosporidium 23 (Supplementary Fig. 8 ), but may lead to higher production of microplastics from car tyres 31 (Supplementary Fig. 7 ) as a result of industrialization. All these interactions are considered together with different trends in the population growth (Supplementary Fig. 3 ), urbanization rates (Supplementary Figs. 1 and 2 ) and treatment levels (Supplementary Figs. 9 – 13 ) among scenarios and regions.
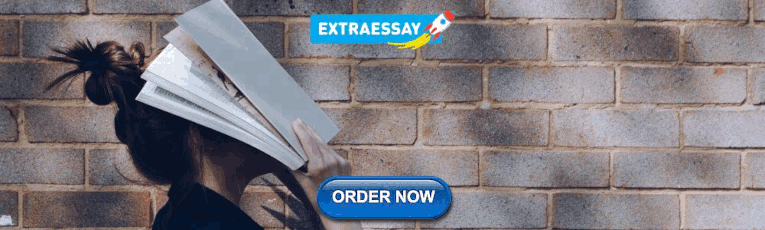
Future river pollution in Africa
Future river pollution is projected to be 11–18 times higher than in 2010 in the scenario with high urbanization and low wastewater improvements (High urb –Low wwt ). This range is for increasing inputs of the five pollutants by at least 30% during the period of 2010–2100 (Fig. 5 ). Africa may become a major contributor to river pollution in the world (Fig. 4 ). For example, by 2100, up to half of the global inputs of multiple pollutants are projected in Africa in High urb –Low wwt (Fig. 4 ). For comparison: in 2010 the contribution of African rivers to the global river pollution was <5% (Fig. 4 ). All scenarios project increasing river pollution in the future for Africa (Figs. 5 and 6 ). This is largely associated with the projected population growth and assumed wastewater treatment. The African population is projected to more than double in many sub-basins during 2010–2100 in all scenarios (Supplementary Fig. 3 ). Many people will live in urban areas (High urb –Low wwt and High urb –Mod wwt , Supplementary Figs. 1 – 3 ). More people will inevitably generate more waste, and this may not be treated effectively enough (e.g., High urb –Low wwt ). This all explains the large future increases in river pollution in Africa (Fig. 5 ). In the low urbanization scenario (Low urb –Low wwt ), less people will live in urban areas, and a lower percentage of people will be connected to sewer systems. Thus, open defecation may continue in Low urb –Low wwt especially by 2050. This is an important source of nutrients and Cryptosporidium to African rivers in this scenario. Supplementary Figs. 18 , 19 , 20 and 21 show results for increasing inputs of the five pollutants by at least 10% and 50% during the period of 2010–2100. Supplementary Figs. 22 , 23 , 24 , 25 and 26 show future trends in river pollution by individual pollutants. Supplementary Figs. 27 , 28 and 29 show scenarios and sub-basins where open defecation is an important source of P, N and Cryptosporidium in rivers.
Future river pollution in Asia
Future river pollution is projected to be 2–3 times higher than in 2010 in the scenario with high urbanization and low wastewater improvements (High urb –Low wwt ). This range is for at least 30% increases in inputs of the five pollutants for the period 2010–2100 (Fig. 5 ). Exceptions are rivers in sub-basins of China (Fig. 5 ). These rivers are projected to be cleaner in 2100 than in 2050, but inputs of the pollutants may still be higher in 2100 than in 2010 in the urbanized scenarios with the low (High urb –Low wwt and Low urb –Low wwt ) and moderate (Mod urb –Mod wwt and High urb –Mod wwt ) wastewater treatment improvements (Fig. 5 ). The Chinese population is projected to decrease in the future in all scenarios (Supplementary Fig. 3 ). However, with the rapid urbanization (Supplementary Figs. 1 – 2 ), the wastewater treatment (Supplementary Figs. 9 – 13 ) may not keep up with the pollution loads. This explains higher river pollution levels. This is different for some other Asian countries such as India and Pakistan. By 2050, the total population of India and Pakistan will have increased (Supplementary Fig. 3 ). By 2100, the total population will have decreased or increased depending on the socio-economic development in the scenarios (Supplementary Fig. 3 , Supplementary Tables 4 – 6 for the scenario description). However, the wastewater treatment is poorer or absent compared to the Chinese sub-basins (Supplementary Figs. 9 – 13 ), resulting in more pollutants in rivers (Fig. 5 , Supplementary Figs. 18 – 21 ).
Future river pollution in Europe and North America
Many rivers in Europe and North America may be cleaner in the future. European rivers (Western, Northern and Southern) may get cleaner in the future because of high removal efficiencies to treat wastewater (Supplementary Figs. 9 – 13 ). However, in the High urb –Mod wwt scenario, high wastewater treatment efficiencies (>50% for all pollutants) may not be enough to reduce future pollution to the level below 2010. For American rivers, future trends differ largely between South and North in the scenarios with the low (Low urb –Low wwt ) and high (High urb –Low wwt ) urbanization trends. In the Low urb –Low wwt scenario, lower increases (<30%) in inputs of pollutants are projected for many Northern rivers whereas higher increases (>30%) for most Southern rivers (Fig. 5 , Supplementary Figs. 18 – 21 ). This difference can be explained by the higher population growth (Supplementary Figs. 1 – 3 ) and less efficient wastewater treatment (Supplementary Figs. 9 – 13 ) in South America compared to North America. In the High urb –Low wwt scenario, higher increases in river pollution are projected for South America by 2050, but lower by 2100. This is associated with the decreased population (Supplementary Fig. 3 ) and with the increased efficiencies of wastewater treatment between 2050 and 2100 (Supplementary Figs. 9 – 13 ). Rivers in Australia may be more polluted in the future (Fig. 5 ). Exceptions are the Low urb –Low wwt and High urb –Low wwt scenarios with less pollution in 2100 than in 2050. This is largely associated with the decreasing population during 2050–2100 (Supplementary Figs. 1 – 13 , 18 – 21 ).
Reducing future river pollution
Advanced wastewater treatment can reduce future river pollution in many world regions, but not in Africa (High urb –High wwt ). In High urb –High wwt, all developed countries (HDI > 0.785) will shift completely towards tertiary treatment with enough capacities and high efficiencies to remove pollutants from the wastewater (>75% for all pollutants, Supplementary Figs. 1 – 14 ). Examples of such technologies are annomox 47 for N, calcium precipitation for P 48 , disinfection by Ultraviolet radiation for Cryptosporidium 42 , reverse osmosis for nutrients 41 and microplastics 49 . Developing countries (HDI < 0.785) will also shift towards tertiary technologies, but in combination with secondary technologies 10 , 46 (Supplementary Figs. 1 – 14 ). Open defecation will stop by 2100. Thus, High urb –High wwt shows the technical potential of advanced technologies with enough treatment capacities to reduce future pollution from highly urbanized areas.
It will be difficult to reduce future river pollution in Africa to the level of 2010, even with advanced technologies (High urb –High wwt , Fig. 5 ). Inputs of most pollutants to many African rivers are projected to increase by at least 30% during 2010–2100 in High urb –High wwt (Fig. 5 ). The main reason is an increase in the total population, which is much higher (>doubling) than in other world regions (Supplementary Fig. 3 ). As a result, implementing advanced technologies in 2100 may help to reduce inputs of most pollutants to the level of 2050, but not to the level of 2010. For many other world’s rivers, advanced technologies with enough treatment capacities are projected to lower future inputs of pollutants in High urb –High wwt (Fig. 5 , Supplementary Fig. 20 ). This may have a positive impact on the overall pollution status depending also on the contribution of diffuse sources from agriculture. However, for some rivers in Asia (e.g. India, Pakistan), inputs of most pollutants from point sources will still increase by 2050, but may be lower by 2100 in High urb –High wwt (Fig. 5 ). Some rivers in North America, Middle Asia and Australia are projected to have higher inputs of pollutants in 2100 than in 2050, but lower than in 2010 (Fig. 5 , Supplementary Fig. 20 ). These trends are the net effect of the population growth, urbanization and wastewater treatment in High urb –High wwt (Figs. 2 , 5 and 6 ).
Scenario analyses are widely used to explore possible futures 1 , 34 , 36 , 50 , 51 , 52 . Our five scenarios are a combination of possible trends in urbanization, socio-economic development (existing SSPs 1 , 36 , 53 ) and our assumptions on sanitation, wastewater treatment capacities and removal efficiencies of pollutants. Our assumptions may, however, seem ambitious (Supplementary Tables 5 and 6 ). For example, we assume the full implementation of advanced technologies with enough treatment capacities in High urb –High wwt for all developed countries. We did this to show the effects of sustainable practices in urban areas on increasing the availability of clean water for people and nature. This assumption, however, might be ambitious to achieve. In our scenarios, we reflect a relation between urbanization (e.g., more urban people) and sewer connections (see High urb –Low wwt, High urb –Med wwt ) with sustainable urbanization practices (see High urb –High wwt ). This relation may, however, not emerge everywhere in the world. On the other hand, we explore possible futures; we do not state how likely or desirable these futures are. Our scenarios aim to identify impacts of future urbanization (e.g., differences between Low urb –Low wwt and High urb –Low wwt ) and the technical potentials of proven wastewater treatment technologies to reduce future river pollution from point sources (e.g., differences between High urb –Low wwt and High urb –High wwt ). Our insights may contribute to the formulation of sustainable urbanization practices where wastewater treatment is effective enough to reduce pollutants in the urban waste (e.g., SDG11) and thus to increase the availability of clean water in the future (e.g., SDG6).
Our global multi-pollutant model quantifies, simultaneously, five pollutants in rivers with consistent datasets in space and time. However, uncertainties exist. The model is developed based on existing, evaluated models for pollutants 11 , 18 , 20 , 23 , 29 , 32 (e.g., comparisons with observed concentrations and sensitivity analyses). We further evaluate our combined model using five approaches 54 (see the “Methods” section). First, we compare our model outputs with existing studies (see the “Methods” section, Supplementary Table 7 ), showing a good agreement for the five pollutants. Second, we compare the spatial pattern of pollution problems with existing models 8 , 9 , 10 , 11 , 12 , 16 , 55 , 56 , indicating the river pollution in densely populated and highly urbanized areas (Figs. 3 – 5 , Supplementary Tables 7 and 8 ). However, existing studies did not focus on a simultaneous reduction of the five pollutants from urbanized activities in the 21st century, which is a multi-pollutant perspective of our study. Third, we performed a sensitivity analysis for pollution hotspots. We define multi-pollutant hotspots as places with >30% increases in two or more pollutants between 2010 and future years (Fig. 5 ). This is an elegant way to combine the five pollutants. We realize that the 30% threshold is arbitrary. The results should, therefore, be interpreted as warning signals of future river pollution. In the sensitivity analysis, we changed the 30% threshold to 10% (Supplementary Figs. 18 – 19 ) and 50% (Supplementary Figs. 20 – 21 ). The results confirm the robustness of our main messages about future multi-pollutant hotspots. Fourth, we performed a sensitivity analysis for all important model inputs underlying the calculations (Supplementary Tables 9 , 10 , 11 and 12 , Supplementary Fig. 17 ). In total, 25 model inputs are changed with ±10%, resulting in 50 model runs for 10,226 sub-basins and five pollutants. The results show that the model is not very sensitive to changes in most model inputs. For most sub-basins, the model outputs are relatively sensitive to changes in <5 model inputs. These inputs are related to HDI, wastewater treatment types and removal efficiencies. The 10% changes in these inputs, resulted in up to 5% change in model output for sub-basins covering over two-thirds of the global surface area (see details in the “Methods” section for all sub-basins). Fifth, we compare model inputs with independent datasets (Supplementary Table 8 , Supplementary Figs. 15 and 16 ). All this gives trust in the model performance (see the “Methods” section).
Our results are future oriented. We focus on trends in future hotspots of multi-pollutant problems in the world. We believe that not all model uncertainties affect our main messages about trends. We also realize that our results are relatively sensitive to the assumptions on future HDI and wastewater treatment (see Approach 4 in the “Methods” section and sensitivity analysis). For HDI, we assumed an increase of 0, 10 and 20% between 2010 and 2050 and further increase by 2100 depending on scenario (Supplementary Tables 5 – 6 ). For wastewater treatment rates, we assumed a shift towards a next treatment type between 2010 and future years (e.g., 0–50% shift depending on scenario). To increase trust in our assumptions for future trends, we compared our model inputs with other independent studies. We did this for our five scenarios (Supplementary Table 8 , Supplementary Fig. 15 ). For example, future trends in our HDI between 2010 and future years are strongly in line with an independent study 57 ( R 2 above 0.88, see Supplementary Fig. 15 ). Crespo Cuaresma and Lutz 57 took into account differences in human development and their socio-economic wealth in projecting future HDI. Our wastewater treatment types in 2050 are also well compared with an independent study 10 (Supplementary Table 8 ).
Another potential source of uncertainties relates to the local variation in pollution levels. For example, sewage overflows may happen under heavy rain events, causing local peaks in water pollution. Such events are time dependent and may also contribute to global pollution levels 58 . We do not account for such local events in our model. We, however, believe that such omissions of events do not affect our messages for the multi-pollutants worldwide. This is because we explore future trends in the multi-pollutant hotspots worldwide that are influenced by global change, urbanization and wastewater treatment. Local analyses should, however, account for the impact of local events on local water quality (e.g., cities).
Our study aims to analyze the impact of the socio-economic drivers (e.g., GDP) and urbanization on future inputs of pollutants to rivers from point sources worldwide. However, we do not consider the transport of pollutants to rivers from agricultural fields, nor the impact of climate change on future river pollution. Next steps could be to further develop our global multi-pollutant model by calculating inputs of pollutants from agricultural fields and associated river export of pollutants. This will allow to explicitly combine the impact of both climate change and of socio-economic developments.
A multi-pollutant approach supports the search for effective solutions. A multi-pollutant approach might be more effective in reducing river pollution than a single-pollutant approach (Fig. 1 ). For example, reducing one pollutant may reduce (synergies) or increase (trade-offs) another pollutant. Our study serves as an illustrative example for the five pollutants. For example, increasing sewer connections may increase inputs of the five pollutants to rivers, but decrease inputs of N, P and Cryptosporidium from open defecation (Low urb –Low wwt ; trade-off). Higher economic developments may lead to less excreted Cryptosporidium per capita because of lower infection risks in developed countries 11 , 23 (Supplementary Fig. 8 ), but may generate more N and P in human excreta (Supplementary Figs. 4 – 5 ) as a result of protein-rich food consumption 10 , 46 (trade-off). Synergies also exist. For example, increasing sewer connections with advanced technologies and sufficient wastewater treatment capacities is projected to decrease the inputs of all five pollutants to many rivers in the future (High urb –High wwt ). This is also associated with synergies in treatment technologies to remove multiple pollutants. Some technologies are developed to target specific pollutants (e.g., N 47 , P 48 , Cryptosporidium 42 ). This implies that implementing technologies for one pollutant may not strongly influence another pollutant. However, technologies exist to treat more than one pollutant (e.g., 10 , 38 , 39 , 40 , 42 , 59 ). For example, secondary treatment with removal efficiencies of around 40–50% (assumed in Mod urb –Mod wwt and High urb –Mod wwt ) converts organic N into inorganic and gas, removing N from the waste 10 . They can also facilitate the biodegradation of triclosan 59 . Microplastics can host microorganisms (e.g., Cryptosporidium ) and serve as vectors for chemicals 15 , 49 , 60 . As a result, biofilms and flocs can form in, for example, activated sludge ponds and then settle down 49 . Triclosan can sorb to large particles and also settle down with other pollutants 38 , 39 , 59 . Advanced technologies (assumed in High urb –High wwt ) such as efficient ultrafiltration methods can reduce Cryptosporidium 42 and microplastics 49 , and reverse osmosis can recover nutrients 41 and reduce microplastics 49 . Nature-based solutions such as stabilization ponds and constructed wetlands are largely effective to reduce Cryptosporidium 42 and nutrients 61 . Accounting for synergies and trade-offs is essential to identify effective solutions for multiple pollutants. This can support the achievement of SDG11 for sustainable cities and SDG6 for clean water.
Our results can support policy assessment of water pollution in urban areas, and form the basis for actionable and region-specific solutions. We identify hotspots of urban-related river pollution and show possible effects of future urbanization on river quality under global change. This could help to prioritize short-term actions to avoid river pollution in the 21st century. Improving wastewater treatment is important to avoid multi-pollutant problems in an urbanized world (Fig. 5 , differences between High urb –High wwt and High urb –Low wwt ). Our sensitivity analysis indicates where improved wastewater treatment could have a larger impact (Supplementary Fig. 17 ). Our model indicates that water pollution is related to human development (expressed as human development index). This is important to realize when reducing Cryptosporidium and microplastics. Some countries in the world already introduced policies such as a ban of detergents and triclosan in products. Combing such policies with improved wastewater treatment may contribute to synergetic solutions for achieving SDGs and reducing river pollution from urban waste. For Africa, improving wastewater treatment may not be enough. Controlling the African population growth to reduce waste production in the future may be needed in urban and water policy assessments.
Our study quantifies future trends in inputs of five pollutants to rivers for five scenarios. We argue that a multi-pollutant perspective is needed in quantitative analyses of future trends in global change, urbanization, sanitation and wastewater treatment. We analyzed multiple pollutants simultaneously in a consistent way. We did this for 10,226 sub-basins for 2010, 2050 and 2100. Our insights are in how future trends differ between pollutants, sub-basins and how hotspots of multi-pollutant problems change in the 21st century. Our study provides an example of multi-pollutant problems from urban point sources. We show that future inputs of pollutants are projected to increase with increasing urbanization. We also show that it is technically possible to avoid these increases with advanced proven technologies to treat wastewater, except in Africa. In Africa, clean water availability is projected to remain a challenge because of the fast increasing population. This will consequently challenge the achievement of SDGs 6 and 11 in Africa. Our model may serve as an example for multi-pollutant modelling of diffuse sources such as agricultural runoff and other pollutants, such as pesticides 62 , antibiotics 24 and antimicrobial resistance. Another opportunity is to analyze the economic (e.g., costs), societal, institutional and political feasibilities of future pollution reduction options. This is important to identify region-specific solutions. Our long-term projections can help to increase the awareness of society and decision makers about pollution hotspots in the 21st century. This can facilitate short-term actions in different regions to avoid pollution in the future and contribute to achieve SDGs 6 and 11.
Model description and inputs
We used a model of Strokal et al. 31 that takes the sub-basin scale modelling approach of Strokal et al. 32 for nutrients and integrates modelling approaches for other pollutants 18 , 20 , 23 . We developed it further for future analyses of point-source inputs of pollutants to rivers (Supplementary Table 1 ). Our model quantifies inputs of five pollutants to 10,226 rivers: nitrogen (N), phosphorus (P), microplastics, triclosan and Cryptosporidium for 2010, 2050 and 2100. The model of Strokal et al. 31 was developed for 2010 taking the sub-basin modelling approach of Strokal, et al. 32 for N 29 , 32 , P 29 , 32 and integrating the existing modelling approaches for microplastics 18 , triclsan 20 and Cryptosporidium 23 . We developed the model for the years 2050 and 2100 based on the urbanization storylines of the SSPs and our assumptions. Our multi-pollutant model quantifies simultaneously annual inputs of the five pollutants to rivers at the sub-basin scale using the consistent spatial and temporal dataset for model inputs for 2010, 2050 and 2100. The model quantifies inputs of the five pollutants from sewer systems and open defecation. These are the point sources of the pollutants in rivers. Sewer systems discharge five pollutants to rivers. Open defecation is a point source of N, P and Cryptosporidium in our model. Model evaluation is presented below after the scenario descriptions.
Inputs of the pollutants to rivers from open defecation are quantified as a function of the population that is open defecating and the excretion or consumption rates of pollutants per person per year (Supplementary Tables 1 and 2 ). Inputs of pollutants from sewer systems are quantified as a function of the population that is connected to sewer systems, the excretion or consumption rates of pollutants per person per year and removal efficiencies of pollutants during treatment. We quantified inputs of the pollutants at 0.5° grid and then aggregate the results to 10,226 river sub-basins (Supplementary Table 1 ). Model inputs for 2010 are directly from Strokal, et al. 31 . Model inputs for 2050 and 2100 are based on the SSPs with different trends in urbanization and wastewater treatment (see scenario descriptions below).
Below, we explain how model inputs were derived (Supplementary Tables 1 – 6 ). Population for 2010, 2050 and 2100 are aggregated to 0.5° grid from the global, 0.125 degree cell database of Jones and O’Neill 53 . The number of people with sewer connections and open defecation are quantified at 0.5° grid using the population map of 0.5° grid and the fraction of people with sewer connections or open defecation. For 2010, the fraction of urban and rural people with sewer systems and open defecation were available by country from the Joint Monitoring Program (see details in Strokal et al. 31 and Hofstra and Vermeulen 11 ). We assigned the national values to grids of 0.5° grid. Then, we multiplied the number of people per grid (aggregated from Jones and O’Neill 53 ) with the fraction of people connected to sewer systems or open defecating (based on Hofstra and Vermeulen 11 ). For 2050 and 2100, we made assumptions for the fractions of people connected to sewer systems and with open defecation. These assumptions were based on storylines of SSPs for economy, population and urbanization (Fig. 2 , Supplementary Tables 4 – 6 ). Our assumptions differ among urban and rural people, and among developing and developed countries (see scenario descriptions below).
Excretion or consumption rates of pollutants were largely derived based on existing, evaluated approaches and sources. Excretion rates of N and P in human waste per person are quantified as a function of GDP (gross domestic product) at purchasing power parity, following the approach of Van Drecht et al. 46 , but adjusted to the unit of 2005 (see details in Strokal et al. 31 , Supplementary Tables 1 – 6 ). For 2010, 2050 and 2100, GDP at 0.5° grid was derived from the global SSP database with the projections from the International Institute for Applied Systems Analysis (IIASA, 63 ). P in detergents was from Van Drecht et al. 46 for the world regions (Supplementary Tables 1 – 6 ).
Excretion rates of Cryptosporidium were quantified based on the infection rate in developed (5%) and developing (10%) countries and the excretion rate per ill person (10 9 oocysts) according to Hofstra et al. 23 . For 2010, developed and developing countries were defined based on the Human Development Index (HDI), following the approach of Hofstra et al. 23 : HDI > 0.785 (developed) and HDI < 0.785 (developing). For 2050 and 2100, we made assumptions for HDI for countries depending on SSP storylines for the economy, population growth and urbanization (see scenario descriptions below and Supplementary Tables 4 – 6 ).
Consumption rates of microplastics per person per year were derived directly from Siegfried et al. 18 , but with some modifications (details are in Strokal et al. 31 ). Microplastics in sewer systems result from car tyres, PCPs (personal care products), household dusts and laundry. For PCPs, dust and laundry, consumption rates are 0.071, 0.08 and 0.12 kg of microplastics per person per year according to Siegfried, et al. 18 . We assumed that these values do not change over time. For tyres, this is different. Strokal et al. 31 assumed that developed countries will contribute more microplastics to sewage from car tyres as a side-effect of economic and infrastructural developments. Thus, we assigned 0.18 kg of microplastics from tyres per person for developed countries (HDI > 0.785) and 0.018 kg of microplastics from tyres per person for developing countries (HDI < 0.785) according to Strokal et al. 31 . We assumed changes in HDI by country in the future based on the SSPs storylines (see scenario descriptions below and Supplementary Tables 1 – 6 ).
Consumption rates of triclosan per person in the world were directly taken van Wijnen et al. 20 (0.5 kg per person per year for 2010). We assumed that the consumption rate will not change largely in the future and thus will remain as in 2010.
Removal efficiencies of pollutants during treatment were derived based on the existing studies. For N, P and Cryptosporidium , removal efficiencies were quantified by country using the national distribution of wastewater treatment types (primary, secondary, tertiary, no treatment) and their treatment efficiencies for pollutants, following the approaches of 11 , 23 , 46 (see Supplementary Tables 1 – 6 , Supplementary Figs. 1 – 14 ). The quantified national removal efficiencies were then assigned to corresponding grids of 0.5°. For 2010, national distributions of wastewater treatment types were derived from Hofstra and Vermeulen 11 with a few corrections for countries with missing data (details are in Strokal et al. 31 ). For 2050 and 2100, we assumed changes (low, moderate, high) in the distribution of the treatment types depending on the storylines of SSPs (see scenario descriptions below). These changes imply a shift towards a next treatment type: e.g., from primary to secondary to tertiary (Supplementary Tables 1 – 6 ). Removal efficiencies of pollutants for different treatment types were taken directly from literature (see Supplementary Table 3 ) and do not vary among years.
For triclosan and microplastics, removal efficiencies were quantified based on the approaches of van Wijnen et al. 20 and Siegfried et al. 18 (details are in Strokal et al. 31 ). We used the known removal rate of phosphorus to assume the removal of triclosan and microplastics. For our assumptions, we used data about the removal of triclosan and microplastics from literature 39 , 59 , 64 , 65 , 66 . Based on these data, we related average phosphorus removal in a watershed to triclosan removal. We formulated three classes of triclosan removal (0, 60 or 90%) and related these to known phosphorus removal in each sub-basin (details are in van Wijnen et al. 20 ). A similar approach was carried out for microplastics. We formulated four microplastics removal classes based on literature and related those to the known average phosphorus removal in each sub-basin 18 , 30 . These classes represent an average microplastics removal in each sub-basin. Microplastic removal depends on the size and density of the microplastics. Therefore, the removal at each individual WWTP will be dependent on these and other characteristics. In our study, on a global scale, we chose to assume average removal for each sub-basin.
Scenario description
Storylines of the five scenarios are summarized in Fig. 2 , Supplementary Tables 1 – 6 and Supplementary Figs. 1 – 14 . Our five scenarios are with low urbanization and low wastewater treatment rates (Low urb –Low wwt ), moderate urbanization and moderate wastewater treatment rates (Mod urb –Mod wwt ), high urbanization and low wastewater treatment rates (High urb –Low wwt ), high urbanization and moderate wastewater treatment rates (High urb –Mod wwt ), and high urbanization and high wastewater treatment rates (High urb –High wwt ) (Fig. 2 ). These scenarios follow future trends in the socio-economic development based on the existing SSPs 1 , 63 , combined with our assumptions for population with sewer connections, open defecation and for wastewater treatment capacities and technologies (Supplementary Tables 4 – 6 ). Below, we describe each scenario. Quantitative interpretations of the scenario assumptions are presented in Supplementary Tables 4 – 6 for 2050 and 2100, and inputs are given in Supplementary Figs. 1 – 14 .
The Low urb -Low wwt scenario is based on SSP3 projections for the socio-economic development (Fig. 2 , Supplementary Tables 4 – 6 ). The scenario assumes a fragmented world with difficulties to control population growth. In this world, It is projected a low economic development with low urbanization rates and high population growth. For example, a global population of approximately 12 billion people is projected for 2100, of which 58% will be urban (Supplementary Figs. 1 – 3 ). Low economic developments will not allow to develop technologies largely. For 2050, HDI is assumed to stay as in 2010 and increase by 10% between 2050 and 2100 on a county level (Supplementary Tables 4 – 6 ). The society will not focus on reducing or avoiding future river pollution. As a result, the fraction of the population with sewer connections (around one-third of the global population) and the treatment efficiencies of wastewater (e.g., 14–18% globally depending on pollutant) will remain in 2050 as in 2010 (Supplementary Figs. 3 , 9 – 13 ). The same holds for the wastewater treatment capacities. However, by 2100 more people may be connected to sewer systems (above one-third of the global population). This will result in higher capacities of the wastewater treatment plants with slightly improved treatment technologies (e.g., 21–24% of removal efficiencies globally depending on pollutant). However, future wastewater treatment efficiencies vary largely among world countries: e.g., 0–96% in 2100 depending on region and pollutant. In general, higher wastewater treatment efficiencies are projected for Europe, North America and Australia (Supplementary Figs. 9 – 13 ),
The Mod urb -Mod wwt scenario is based on SSP2 projections of the middle of the road for the socio-economic development (Fig. 2 , Supplementary Tables 4 – 6 ). The scenario assumes a moderate economic development, moderate urbanization rates and moderate population growth compared to the other scenarios. For example, 9 billion people are projected globally for 2100 and 80% will be urban (Supplementary Figs. 1 – 3 ). From 2010, HDI is assumed to increase by 10% by 2050 and further increase by 10% by 2100 on a county level (Supplementary Tables 4 – 6 ). Technological development follows the business as usual trends. As a result, more people will be connected to sewer systems than today (45% in 2050 and 68% in 2100 globally, Supplementary Fig. 3 ). A number of wastewater treatment plants will be constructed to maintain the increasing volume of the wastewater from connected population to sewer systems. The amount of waste that is collected will be treated with slightly improved wastewater treatment. For example, on average, 33–42% of removal efficiencies globally are projected for 2100. This range is for the five pollutants. The removal efficiencies vary largely among regions (0–97% depending on region and pollutant, Supplementary Figs. 9 – 13 ). The number of people connected to sewer systems will be larger for urban (over two-thirds) than for rural (less than one-third) population. Some people may still experience open defecation in 2050. By 2100, all people who opened defecated in 2050 will become connected to sewer systems.
The High urb -Low wwt scenario is based on SSP4 projections for the socio-economic development (Fig. 2 , Supplementary Tables 4 – 6 ). The scenario assumes a large gap between urban and rural developments. The economic development is projected to be moderate compared to the other scenarios. HDI is projected to increase as in the Mod urb –Mod wwt scenario (Supplementary Tables 1 – 3 , Supplementary Fig. 14 ). The population is projected to increase in the future, but not largely: e.g., around 30% between 2010 and 2100 globally. By 2100, the global population is projected to reach 9.3 billion people (Supplementary Fig. 3a ). However, the urban population will develop faster than the rural. Urbanization will be high: e.g., 76% and 90% of the global population will be urban in 2050 and 2100, respectively. As a result, the connection rate of the population to sewer systems will increase in the future for urban areas. For example, 80% of urban and 11% of rural population globally is projected to be connected to sewer systems in 2100 (Supplementary Figs. 1 – 3 ). Wastewater treatment capacities will be enough to maintain the waste from sewer systems and treatment will be improved as in the Mod urb -Mod wwt scenario. For rural areas, the fraction of people connected to sewer systems in 2050 may remain the same as in the Low urb -Low wwt scenario and will be improved by 2100 (Supplementary Tables 4 – 6 ). By 2050, some rural people may still open defecate. By 2100, all rural people who opened defecated in 2050 will become connected to sewer systems with better treatment.
The High urb –Mod wwt scenario is based on SSP5 projections for the socio-economic development (Supplementary Tables 4 – 6 , Fig. 2 ). The scenario assumes a high economic development with high urbanization and low population growth (Fig. 2 , Supplementary Table 4 ). For example, the total population globally is projected to increase by less than 10% between 2010 and 2100, reaching 7.4 billion people in 2100 (Supplementary Fig. 3a ). However, more than 90% of the global population will be urban in 2100. From 2010, HDI is assumed to increase by 20% by 2050 and further increase by 20% by 2100. The technological development is relatively high compared to the Mod urb -Mod wwt scenario. This will lead to a higher population with sewer connections. More than half of the global population will be connected to sewer systems in 2050. For 2100, this number is over two-thirds of the global population (Supplementary Figs. 1 – 3 ). The capacities of the wastewater treatment plants will be enough to manage the amount of waste from sewer systems. However, people will invest less in improving wastewater treatment. People will focus more on the economy rather than on reducing river pollution. As a result, wastewater treatment may follow the business as usual trends. For example, on average, 34–44% of the wastewater treatment efficiencies are projected globally for 2100. However, these efficiencies vary largely among regions (0–97% depending on area and pollutant, Supplementary Figs. 9 – 13 ). Furthermore, some people may still open defecate in nearby water systems in the future. By 2100, all people who opened defecated in 2050 will become connected to sewer systems.
The High urb –High wwt scenario is based on SSP1 projections for the socio-economic development (Fig. 2 , Supplementary Tables 4 – 6 ). The society will develop fast with high urbanization rates as comparable to the High urb –Mod wwt scenario. The global population is projected to reach 6.9 billion people in 2100 (Supplementary Fig. 3a ). The share of urban people globally is projected to be 77% in 2050 and 92% in 2100 (Supplementary Figs. 1 – 3 ). The share of the total connected people to sewer systems is projected to be 55% in 2050 and 82% in 2100. HDI is projected to increase in the same rate as in the High urb –Mod wwt scenario. However, in this world, a strong focus is on reducing or avoiding river pollution by using the best available advanced technologies in all areas. Technological development is high because of the high economic development. People will invest in improving technologies to treat wastewater with multiple pollutants. There will be opportunities to develop technologies for multiple pollutants and combine them with nature-based solutions. As a result, the wastewater treatment is assumed to be improved largely with high removal efficiencies (60–98% depending on year, area and pollutant, Supplementary Figs. 9 – 13 ).
Model evaluation
We evaluated the uncertainties in our model using four approaches following a building trust circle method 54 . This method has been applied in several water quality studies 32 , 67 , 68 . First, we compare model outputs with existing studies. Second, we compare the spatial pattern of the pollution problems with existing models for individual pollutants. Third, we perform a sensitivity analysis for pollution hotspots. Fourth, we perform a comprehensive sensitivity analysis for all important model inputs underlying the calculations. Fifth, we compare model inputs with independent datasets. Model validation against observed concentrations is, unfortunately, challenging. This is because our model does not quantify concentrations. Some of the existing global models calculate concentrations and were evaluated against observations (Supplementary Tables 7 – 8 ). Thus, we used those models to compare their results with ours for individual pollutants. Below, we elaborate on these five approaches. Details are in Supplementary Tables 7 – 12 and Supplementary Figs. 15 , 17 .
Approach 1: evaluating model outputs by comparing them with other models and studies for individual pollutants. This comparison is presented in Supplementary Table 7 . The results show that our model outputs for global inputs of nitrogen, phosphorus, microplastics, triclosan and Cryptosporidium are generally in line with other models and studies. For example, our model quantified 9.5 Tg of nitrogen to rivers from point sources in 2010. Other models quantified 6.4–10.4 Tg of nitrogen to rivers from points sources during 2000–2010 10 , 46 , 69 (Supplementary Table 7 ). For phosphorus, we quantified 1.6 Tg in 2010 whereas the other models quantified 1.0–1.5 Tg for the period of 2000–2010 10 , 46 , 69 . For 2050, we quantified 5.4–21.0 Tg of nitrogen and 0.6–3.5 Tg of phosphorus in 2050 (ranges for the five scenarios). van Puijenbroek et al. 10 quantified 13.5–17.9 Tg of nitrogen and 1.6–2.4 Tg of phosphorus in 2050 under the five SSPs. For Cryptosporidium , our model quantified 1.6 × 10 9 oocysts in 2010 which is 1.1–1.4 × 10 9 oocysts in another model in 2000–2010 11 , 23 (Supplementary Table 7 ). For 2050, our model quantified 0.4–2.9 × 10 9 oocysts (range for the five scenarios). For the Low urb -Low wwt scenario, this value is 2.44 × 10 9 oocysts, which is comparable with 2.28 × 10 9 oocysts from the other model 11 , 23 . To our knowledge, van Wijnen, et al. 20 is the only study quantifying triclosan export by rivers. Our estimates for Danube, Zhujiang and Ganges are comparable with estimates of van Wijnen et al. 20 (Supplementary Table 7 ). For microplastics, our model quantified 0.45 Tg entering rivers globally in 2010. Best 9 indicated loads of 0.41–4.00 Tg of plastics in 32 world’s rivers. This is higher than our estimate because Best 9 accounts for macro- and microplastics whereas we only consider microplastics. Avio et al. 13 indicated 0.27 Tg of plastics to oceans in some regions in the world. This is lower than our estimate because we quantify inputs of plastics to rivers and not to the oceans. The other reasons for the differences between our model and other studies are in data inputs and the spatial level of detail. We focus on sub-basin analyses with the consistent model inputs for multiple pollutants (Supplementary Table 7 , Supplementary Figs. 1 – 13 ).
Approach 2: evaluating model outputs by comparing the spatial variability in pollution hotspots with other studies. We reviewed the literature on pollution hotspots in the world for individual pollutants 8 , 9 , 10 , 11 , 12 , 16 , 55 , 56 , 70 . Our pollution hotspots for multiple pollutants are in line with the existing studies for individual pollutants. For example, most pollution often happens in densely populated and highly urbanized areas 8 , 9 , 10 , 11 , 12 , 16 , 55 , 56 . For example, Best 9 indicated over 80% of large transboundary rivers in the world with multiple pollutants. For many large cities in polluted regions, the demand for water already exceeds its availability. For example, water scarcity (ratio between the water demand and availability) has been already reported for cities in countries such as China (e.g., Shanghai, Beijing), India (e.g., Delhi, Kolkata, Bangalore, Hyderabad), Mexico, North America (e.g., Los Angeles) 70 . In the future, river pollution will further decrease the availability of clean water in many urban regions 4 , 7 , 8 , 71 . We show that it is technically possible to increase the availability of clean water with implementing advanced technologies (High urb _High wwt , Figs. 3 – 6 ). However, future analyses for multi-pollutant hotspots are lacking in the existing literature. A few global models performed future analysis for individual pollutants 10 , 11 , 18 , 20 where urbanization was taken into account by 2050. Their results indicate pollution hotspots where human activities are most intensive, which is in line with our study. However, studies exploring trends in multi-pollutant hotspots by 2100 do not exist. We explore trends in pollution hotspots for multi-pollutant problems covering the entire 21st century under the five scenarios with different socio-economic developments and levels of wastewater treatment.
Approach 3: evaluating model outputs for pollution hotspots by sensitivity analysis. In Fig. 5 , we showed multi-pollutant hotspots. These hotspots were defined as at least a 30% increase in inputs of more than one pollutant to rivers during 2010–2050, 2010–2100 and 2050–2100. This definition is modest and easier to understand and interpret. We checked if the pollution hotspots remain the same by changing a 30% increase to 10% (Supplementary Figs. 18 – 19 ) and 50% (Supplementary Fig. 20 – 21 ). Results of this sensitivity analysis indicate that our main messages stay the same: Africa will become a hotspot region with multiple pollutants in rivers in the 21st century and advanced technologies may help to reduce pollution in many rivers of the world.
Approach 4: evaluating model inputs by a sensitivity analysis. We performed a comprehensive sensitivity analysis for all important model inputs underlying the calculations. In total, there are 25 model input parameters included in this analysis. Every model input was changed by +10% and −10%. As a result, we did 50 runs of the model for the year 2010. We analyzed the results of the 50 runs for 10,226 sub-basins and five pollutants: Cryptosporidium , nitrogen, phosphorus, triclosan and microplastics. Details can be found in Supplementary Tables 9 – 12 and Supplementary Fig. 17 .
In general, increasing the model inputs (13 out of 25) that are responsible for excretion or consumption rates of pollutants in urban waste lead to more pollutants in rivers (Supplementary Tables 10 – 12 ). The opposite is observed when these model inputs are decreased. An exception is HDI for Cryptosporidium and microplastics. Model inputs that are responsible for wastewater treatments (6 out of 25) have the following effect on the model outputs: increases in these inputs lead to less pollutants in rivers and vice versa. Model inputs (6 out of 25) that are responsible for the number of people (urban and rural) connected to sewage systems have the following effect on the model outputs: increases in these inputs lead to more pollutants in rivers and vice versa (Supplementary Tables 10 – 12 ).
We find that model outputs are most sensitive to changes in 2–5 out of the 25 model inputs. The sensitivities vary among sub-basins and pollutants. These model inputs are HDI (sensitive for Cryptosporidium and microplastics), the fractions of secondary (sensitive for triclosan and microplastics) and tertiary (sensitive for all five pollutants) treatment, and the removal efficiencies of secondary (sensitive for triclosan and microplastics) and tertiary (sensitive for all five pollutants) treatment. We analyze model outputs for 10,226 sub-basins that are sensitive to changes in those 2–5 model inputs. Supplementary Tables 11 – 12 show the percentages of the sub-basin areas where model outputs for the five pollutants change by: <5%, 5–10%, 10–50% and >50%. Supplementary Fig. 17 shows the location of the sub-basins for which model outputs are sensitive to one or more model inputs.
The model results for sub-basins covering over two-thirds of the global surface area changed by less than 5% (Supplementary Tables 11 – 12 ). For ≤13% of the global surface area the model outputs changed between 5–10%. This is for all pollutants. For ≤8% of the global area, the changes are between 10–50% in the model outputs. Exceptions are Cryptosporidium and microplastics, which are relatively sensitive for HDI. In one-third of the sub-basin area the model output for Cryptosporidium changed 10–50% as a result of changes in HDI. For microplastic, the changes may be even higher. However, the number of basins with changes above 50% is small. These results show that HDI is an important model input for Cryptosporidium and microplastics (see Supplementary Tables 1 , 9 – 12 ).
Approach 5: evaluating model inputs by comparing them with independent datasets. We provide this comparison in Supplementary Table 8 , Supplementary Figs. 15 and 16 . Comparison results build trust in our model inputs. We compared the following important model inputs for 2010 and 2050 scenarios: total population, population with sewer connections, distribution of treatment types, removal efficiencies of pollutants, nutrients in human excretion (Supplementary Table 8 ). We compared these inputs with van Puijenbroek et al. 10 who recently published global analyses of nutrient inputs to rivers from point sources. We also compared our population from Jones and O’Neill 53 with another global dataset from Kc and Lutz 34 (Supplementary Fig. 16 ). Our model inputs are well compared with the mentioned studies. Furthermore, we compared our HDI index for 2010 and 2050 with the HDI index from Crespo Cuaresma and Lutz 57 (Supplementary Fig. 15 ). HDI is an important input in our model to quantify the excretion of Cryptosporidium . HDI influences the treatment developments and consumption of microplastics associated with the use of car tyres. Our values for HDI under the five scenarios are well compared with the values of Crespo Cuaresma and Lutz 57 ( R 2 > 0.88 for the five scenarios).
Results of these five approaches give us trust in using our multi-pollutant model to explore future trends in inputs of multiple pollutants to rivers from urbanization activities. All data are available in Strokal et al. 72 and Strokal et al. 73 .
Reporting summary
Further information on research design is available in the Nature Research Reporting Summary linked to this article.
Data availability
All the datasets generated and analysed during this study are publicly available in the Data Archiving and Networked Services (DANS Easy) repository: https://doi.org/10.17026/dans-zyx-jce3 73 . The data will be available for download from 01–04–2021. The data supporting the findings of this study are described in the following metadata record: https://doi.org/10.6084/m9.figshare.13333796 72 .
Code availability
All equations to the model are provided in the supplementary information files of this study and in the Data Archiving and Networked Services (DANS Easy) repository: https://doi.org/10.17026/dans-zyx-jce3 . The data will be available for download from 01–04–2021.
Jiang, L. & O’Neill, B. C. Global urbanization projections for the Shared Socioeconomic Pathways. Glob. Environ. Change 42 , 193–199 (2017).
Article Google Scholar
Acuto, M., Parnell, S. & Seto, K. C. Building a global urban science. Nat. Sustain. 1 , 2–4 (2018).
Seto, K. C., Golden, J. S., Alberti, M. & Turner, B. L. Sustainability in an urbanizing planet. Proc. Natl Acad. Sci. USA 114 , 8935–8938 (2017).
Article CAS Google Scholar
Flörke, M., Schneider, C. & McDonald, R. I. Water competition between cities and agriculture driven by climate change and urban growth. Nat. Sustain. 1 , 51–58 (2018).
Li, X., Zhou, Y., Eom, J., Yu, S. & Asrar, G. R. Projecting global urban area growth through 2100 based on historical time‐series data and future Shared Socioeconomic Pathways. Earth’s Future 7 , 351–362 (2019).
Keeler, B. L. et al. Social-ecological and technological factors moderate the value of urban nature. Nat. Sustain. 2 , 29–38 (2019).
Van Vliet, M. T. H., Florke, M. & Wada, Y. Quality matters for water scarcity. Nat. Geosci. 10 , 800–802 (2017).
Nagendra, H., Bai, X., Brondizio, E. S. & Lwasa, S. The urban south and the predicament of global sustainability. Nat. Sustain. 1 , 341–349 (2018).
Best, J. Anthropogenic stresses on the world’s big rivers. Nat. Geosci. 12 , 7–21 (2018).
Van Puijenbroek, P. J. T. M., Beusen, A. H. W. & Bouwman, A. F. Global nitrogen and phosphorus in urban waste water based on the Shared Socio-economic Pathways. J. Environ. Manage. 231 , 446–456 (2019).
Hofstra, N. & Vermeulen, L. C. Impacts of population growth, urbanisation and sanitation changes on global human Cryptosporidium emissions to surface water. Int. Hyg. Envir. Heal. 219 , 599–605 (2016).
Vermeulen, L. C., de Kraker, J., Hofstra, N., Kroeze, C. & Medema, G. Modelling the impact of sanitation, population growth and urbanization on human emissions of Cryptosporidium to surface waters—A case study for Bangladesh and India. Environ. Res. Lett. 10 , 094017 (2015).
Avio, C. G., Gorbi, S. & Regoli, F. Plastics and microplastics in the oceans: from emerging pollutants to emerged threat. Mar. Environ. Res. 128 , 2–11 (2017).
Boucher, J., Friot, D. & Boucher, J. Primary Microplastics in the Oceans: A Global Evaluation of Sources . (IUCN Gland, Switzerland, 2017).
Koelmans, A., Bakir, A., Burton, G. & Janssen, C. Microplastic as a vector for chemicals in the aquatic environment: critical review and model-supported reinterpretation of empirical studies. Environ. Sci. Technol. 50 , 3315–3326 (2016).
Lebreton, L. C. et al. River plastic emissions to the world’s oceans. Nat. Commun. 8 , 15611 (2017).
Schmidt, C., Krauth, T. & Wagner, S. Export of Plastic Debris by Rivers into the Sea. Environ. Sci. Technol. 51 , 12246–12253 (2017).
Siegfried, M., Koelmans, A. A., Besseling, E. & Kroeze, C. Export of microplastics from land to sea. A modelling approach. Water Res. 127 , 249–257 (2017).
Diamond, J. et al. Use of prospective and retrospective risk assessment methods that simplify chemical mixtures associated with treated domestic wastewater discharges. Environ. Toxicol. Chem. 37 , 690–702 (2018).
Van Wijnen, J., Ragas, A. & Kroeze, C. River export of triclosan from land to sea: a global modelling approach. Sci. Total Environ. 621 , 1280–1288 (2017).
Damania, R., Desbureaux, S., Rodella, A.-S., Russ, J. & Zaveri, E. Quality Unknown: The Invisible Water Crisis. Washington, DC: World Bank. © World Bank, https://openknowledge.worldbank.org/handle/10986/32245 License: CC BY 3.0 IGO. http://hdl.handle.net/10986/32245 , 142 pp., (2019).
Seitzinger, S. P. et al. Global river nutrient export: a scenario analysis of past and future trends. Glob. Biogeochem. Cycles 24 , GB0A08 (2010).
Hofstra, N., Bouwman, A. F., Beusen, A. H. W. & Medema, G. J. Exploring global Cryptosporidium emissions to surface water. Sci. Total Environ. 442 , 10–19 (2013).
Font, C., Bregoli, F., Acuña, V., Sabater, S. & Marcé, R. GLOBAL-FATE: A GIS-based model for assessing contaminants fate in the global river network. Geosci. Model Dev. Discuss. 2019 , 1–30 (2019).
Google Scholar
Breitburg, D. et al. Declining oxygen in the global ocean and coastal waters. Science 359 , eaam7240 (2018).
Diaz, R. J. Overview of hypoxia around the world. J. Environ. Qual. 30 , 275–281 (2001).
Kiulia, N. M. et al. Global occurrence and emission of rotaviruses to surface waters. Pathogens 4 , 229–255 (2015).
Beusen, A., Bouwman, A., Van Beek, L., Mogollón, J. & Middelburg, J. Global riverine N and P transport to ocean increased during the twentieth century despite increased retention along the aquatic continuum. Biogeosci. Discuss. 12 , 20123–20148 (2015).
Mayorga, E. et al. Global Nutrient Export from WaterSheds 2 (NEWS 2): model development and implementation. Environ. Modell. Softw. 25 , 837–853 (2010).
Van Wijnen, J., Ragas, A. M. J. & Kroeze, C. Modelling global river export of microplastics to the marine environment: Sources and future trends. Sci. Total Environ. 673 , 392–401 (2019).
Strokal, M. et al. Global multi-pollutant modelling of water quality: scientific challenges and future directions. Curr. Opin. Env. Sust. 36 , 116–125 (2019).
Strokal, M., Kroeze, C., Wang, M., Bai, Z. & Ma, L. The MARINA model (Model to Assess River Inputs of Nutrients to seAs): model description and results for China. Sci. Total Environ. 562 , 869–888 (2016).
Dellink, R., Chateau, J., Lanzi, E. & Magné, B. Long-term economic growth projections in the Shared Socioeconomic Pathways. Glob. Environ. Change 42 , 200–214 (2017).
Kc, S. & Lutz, W. The human core of the shared socioeconomic pathways: population scenarios by age, sex and level of education for all countries to 2100. Glob. Environ. Change 42 , 181–192 (2017).
Leimbach, M., Kriegler, E., Roming, N. & Schwanitz, J. Future growth patterns of world regions – A GDP scenario approach. Glob. Environ. Change 42 , 215–225 (2017).
O’Neill, B. C. et al. The roads ahead: narratives for shared socioeconomic pathways describing world futures in the 21st century. Glob. Environ. Change 42 , 169–180 (2017).
Zhao, Y. & Xu, L. Analysis of nitrogen and phosphorus removal operation in biological systems in sewage treatment plant (in Chinese). Environ. Ecol. Three Gorges 2 , 29–31 (2009).
Halden, R. U. & Paull, D. H. Co-occurrence of triclocarban and triclosan in US water resources. Environ. Sci. Technol. 39 , 1420–1426 (2005).
Thompson, A., Griffin, P., Stuetz, R. & Cartmell, E. The fate and removal of triclosan during wastewater treatment. Water Envion. Res. 77 , 63–67 (2005).
Chen, X. et al. Ozonation products of triclosan in advanced wastewater treatment. Water Res. 46 , 2247–2256 (2012).
Mondor, M., Masse, L., Ippersiel, D., Lamarche, F. & Masse, D. Use of electrodialysis and reverse osmosis for the recovery and concentration of ammonia from swine manure. Bioresour. Technol. 99 , 7363–7368 (2008).
Nasser, A. M. Removal of Cryptosporidium by wastewater treatment processes: a review. J. Water Health 14 , 1–13 (2016).
Liao, P., Chen, A. & Lo, K. Removal of nitrogen from swine manure wastewaters by ammonia stripping. Bioresour. Technol. 54 , 17–20 (1995).
Liu, Y., Kwag, J.-H., Kim, J.-H. & Ra, C. Recovery of nitrogen and phosphorus by struvite crystallization from swine wastewater. Desalination 277 , 364–369 (2011).
Selman, M., Greenhalgh, S., Diaz, R. & Sugg, Z. Eutrophication and hypoxia in coastal areas: a global assessment of the state of knowledge. World Resources Institute 284 , 1–6 (2008).
Van Drecht, G., Bouwman, A. F., Harrison, J. & Knoop, J. M. Global nitrogen and phosphate in urban wastewater for the period 1970 to 2050. Glob. Biogeochem. Cycles 23 , GB0A03 (2009).
Kartal, B., Kuenen, J. & Van Loosdrecht, M. Sewage treatment with anammox. Science 328 , 702–703 (2010).
Jaffer, Y., Clark, T. A., Pearce, P. & Parsons, S. A. Potential phosphorus recovery by struvite formation. Water Res. 36 , 1834–1842 (2002).
Sun, J., Dai, X., Wang, Q., van Loosdrecht, M. C. & Ni, B.-J. Microplastics in wastewater treatment plants: detection, occurrence and removal. Water Res. 152 , 21–37 (2019).
Kok, K., Pedde, S., Gramberger, M., Harrison, P. A. & Holman, I. P. New European socio-economic scenarios for climate change research: operationalising concepts to extend the Shared Socio-economic Pathways. Reg. Environ. Change 19 , 643–654 (2019).
Ma, L. et al. Exploring future food provision scenarios for China. Environ. Sci. Technol. 53 , 1385–1393 (2019).
Pedde, S. et al. Bridging uncertainty concepts across narratives and simulations in environmental scenarios. Reg. Environ. Change 19 , 655–666 (2019).
Jones, B. & O’Neill, B. Spatially explicit global population scenarios consistent with the Shared Socioeconomic Pathways. Environ. Res. Lett. 11 , 084003 (2016).
Strokal, M. River Export of Nutrients to the Coastal Waters of China: The Marina Model to Assess Sources, Effects and Solutions (PhD thesis). Wageningen University, Wageningen, The Netherlands 235 pp, (2016).
UNEP. A Snapshot of the World’s Water Quality: Towards a Global Assessment . 162pp (United Nations Environment Programme, Nairobi, Kenya, 2016).
Vörösmarty, C. J. et al. Global threats to human water security and river biodiversity. Nature 467 , 555–561 (2010).
Crespo Cuaresma, J. & Lutz, W. The demography of human development and climate change vulnerability: a projection exercise. Vienna Yearb Popul. Res. 1 , 241–261 (2016).
McClain, M. E. et al. Biogeochemical hot spots and hot moments at the interface of terrestrial and aquatic ecosystems. Ecosystems 6 , 301–312 (2003).
Butler, E., Whelan, M. J., Sakrabani, R. & van Egmond, R. Fate of triclosan in field soils receiving sewage sludge. Environ. Pollut. 167 , 101–109 (2012).
Kooi, M., Van Nes, E. H., Scheffer, M. & Koelmans, A. A. Ups and downs in the ocean: effects of biofouling on the vertical transport of microplastics. Environ. Sci. Technol. 51 , 7963–7971 (2017).
Vymazal, J. Removal of nutrients in various types of constructed wetlands. Sci. Total Environ. 380 , 48–65 (2007).
Ippolito, A. et al. Modeling global distribution of agricultural insecticides in surface waters. Environ. Pollut. 198 , 54–60 (2015).
Crespo Cuaresma, J. Income projections for climate change research: a framework based on human capital dynamics. Glob. Environ. Change 42 , 226–236 (2017).
Heidler, J. & Halden, R. U. Mass balance assessment of triclosan removal during conventional sewage treatment. Chemosphere 66 , 362–369 (2007).
Von der Ohe, P. C. et al. A new risk assessment approach for the prioritization of 500 classical and emerging organic microcontaminants as potential river basin specific pollutants under the European Water Framework Directive. Sci. Total Environ. 409 , 2064–2077 (2011).
Dann, A. B. & Hontela, A. Triclosan: environmental exposure, toxicity and mechanisms of action. J. Appl. Toxicol. 31 , 285–311 (2011).
Wang, M. et al. Excess nutrient loads to Lake Taihu: Opportunities for nutrient reduction. Sci. Total Environ. 664 , 865–873 (2019).
Wang, M., Kroeze, C., Strokal, M., van Vliet, M. T. & Ma, L. Global change can make coastal eutrophication control in China more difficult. Earth’s Future 8 , 1–19 (2020).
Morée, A., Beusen, A., Bouwman, A. & Willems, W. Exploring global nitrogen and phosphorus flows in urban wastes during the twentieth century. Glob. Biogeochem. Cycles 27 , 1–11 (2013).
McDonald, R. I. et al. Water on an urban planet: Urbanization and the reach of urban water infrastructure. Glob. Environ. Change 27 , 96–105 (2014).
West, P. C. et al. Leverage points for improving global food security and the environment. Science 345 , 325–328 (2014).
Strokal, Maryna; et al. Metadata supporting the article: Urbanization: an increasing source of multiple pollutants to rivers in the 21st century. figshare https://doi.org/10.6084/m9.figshare.13333796 (2020).
Strokal, M. et al. Urbanization: an increasing source of multiple pollutants to rivers in the 21st century. Wageningen University & Research https://doi.org/10.17026/dans-zyx-jce3 (2021).
Download references
Acknowledgements
M.S. (the corresponding author) was financially supported by a Veni-grant (0.16.Veni.198.001) and a KNAW-MOST SURE + project (5160957392).
Author information
Authors and affiliations.
Water Systems and Global Change group, Wageningen University & Research, Wageningen, the Netherlands
Maryna Strokal, Wietse Franssen, Nynke Hofstra, Fulco Ludwig, J. Emiel Spanier & Carolien Kroeze
Key Laboratory of Agricultural Water Resources, Hebei Key Laboratory of Soil Ecology, Center for Agricultural Resources Research, Institute of Genetic and Developmental Biology, The Chinese Academy of Sciences, Hebei, China
Zhaohai Bai & Lin Ma
Aquatic Ecology and Water Quality Management group, Wageningen University & Research, Wageningen, the Netherlands
Albert A. Koelmans
PBL Netherlands Environmental Assessment Agency, the Hague, the Netherlands
Peter van Puijenbroek
Centre for Infectious Disease Control, National Institute for Public Health and the Environment (RIVM), Bilthoven, the Netherlands
Lucie C. Vermeulen
Department of Physical Geography, Utrecht University, Utrecht, the Netherlands
Michelle T. H. van Vliet
Open University, Faculty of Science - Environmental Sciences Dept, Heerlen, the Netherlands
Jikke van Wijnen
You can also search for this author in PubMed Google Scholar
Contributions
M.S. led this manuscript. M.S. was responsible for designing the manuscript, developing a multi-pollutant model, and analyzing and writing the manuscript. C.K. substantially assisted in designing the manuscript, developing the model and analyzing the results. Z.B., W.F., N.H., A.A.K., L.V., M.T.H.V., J.E.S. and J.W., contributed largely in developing the global multi-pollutant model that was used in the manuscript for future analyses of the impact of urbanization on river pollution. They and other authors provided information to the manuscript and advised on the analyses. All authors assisted the interpretations of the Shared Socio-economic Pathways. These pathways are used in the manuscript for multiple pollutants. All authors read and commented on the text. All authors approved the final version and were involved in the accountability for all aspects of the manuscript.
Corresponding author
Correspondence to Maryna Strokal .
Ethics declarations
Competing interests.
The authors declare no competing interests.
Additional information
Publisher’s note Springer Nature remains neutral with regard to jurisdictional claims in published maps and institutional affiliations.
Supplementary information
Supplementary information, reporting summary, rights and permissions.
Open Access This article is licensed under a Creative Commons Attribution 4.0 International License, which permits use, sharing, adaptation, distribution and reproduction in any medium or format, as long as you give appropriate credit to the original author(s) and the source, provide a link to the Creative Commons license, and indicate if changes were made. The images or other third party material in this article are included in the article’s Creative Commons license, unless indicated otherwise in a credit line to the material. If material is not included in the article’s Creative Commons license and your intended use is not permitted by statutory regulation or exceeds the permitted use, you will need to obtain permission directly from the copyright holder. To view a copy of this license, visit http://creativecommons.org/licenses/by/4.0/ .
Reprints and permissions
About this article
Cite this article.
Strokal, M., Bai, Z., Franssen, W. et al. Urbanization: an increasing source of multiple pollutants to rivers in the 21st century. npj Urban Sustain 1 , 24 (2021). https://doi.org/10.1038/s42949-021-00026-w
Download citation
Received : 04 December 2019
Accepted : 20 August 2020
Published : 27 April 2021
DOI : https://doi.org/10.1038/s42949-021-00026-w
Share this article
Anyone you share the following link with will be able to read this content:
Sorry, a shareable link is not currently available for this article.
Provided by the Springer Nature SharedIt content-sharing initiative
Quick links
- Explore articles by subject
- Guide to authors
- Editorial policies
Sign up for the Nature Briefing newsletter — what matters in science, free to your inbox daily.


Essay on Pollution in Cities
Students are often asked to write an essay on Pollution in Cities in their schools and colleges. And if you’re also looking for the same, we have created 100-word, 250-word, and 500-word essays on the topic.
Let’s take a look…
100 Words Essay on Pollution in Cities
Introduction.
Pollution in cities is a major concern worldwide. It affects our health and environment, making city life challenging.
Types of Pollution
Cities face various types of pollution like air, water, noise, and land. Cars, factories, and waste contribute to this problem.
Effects of Pollution
Pollution harms our health, causing diseases like asthma. It also affects plants, animals, and our climate.
We can reduce pollution by using public transport, recycling, and planting trees. Governments should also enforce strict pollution control laws.
250 Words Essay on Pollution in Cities
Pollution in cities has emerged as a significant concern, causing detrimental effects on both human health and the environment. Rapid urbanization, industrialization, and overpopulation have exacerbated the issue, making it a pressing challenge for the modern world.
The Root Causes
The primary sources of urban pollution consist of vehicular emissions, industrial waste, and improper waste disposal. The rising number of vehicles in cities contributes significantly to air pollution, releasing harmful gases such as carbon monoxide and nitrogen oxides. Industrial units, on the other hand, discharge toxic effluents into water bodies, causing water pollution. In addition, the lack of effective waste management systems results in the accumulation of solid waste, leading to soil pollution.
Health and Environmental Impacts
The adverse health effects of urban pollution are alarming. Exposure to polluted air can lead to respiratory disorders, cardiovascular diseases, and even cancer. Water pollution, meanwhile, can cause waterborne diseases and disrupt aquatic ecosystems. Soil pollution affects agricultural productivity and can lead to food contamination.
In conclusion, pollution in cities is a multifaceted issue that requires comprehensive strategies for mitigation. This includes promoting sustainable transportation, implementing stringent regulations on industrial waste, and improving waste management systems. Collective efforts from governments, industries, and individuals are crucial to combat this urban menace and ensure a healthier and safer environment for future generations.
500 Words Essay on Pollution in Cities
Urban pollution is a critical issue that has been escalating at an alarming rate. Cities, being the epicenters of human civilization, industrialization, and modernization, are grappling with numerous pollution-related challenges. The severity of pollution in cities is a result of various factors, including rapid urbanization, increased industrial activities, and population growth.
The Nature of Urban Pollution
Water pollution in cities is often due to the improper disposal of industrial waste into water bodies, leading to their contamination. This pollution not only affects aquatic life but also poses a significant risk to human health when contaminated water is used for consumption or irrigation. Land pollution, on the other hand, is mainly due to improper waste management, leading to the accumulation of solid waste, which can contaminate the soil and groundwater.
The Impact of Urban Pollution
The impacts of pollution in cities are far-reaching and multifaceted. On a health level, exposure to pollutants can lead to a myriad of diseases and health complications, ranging from respiratory problems to cardiovascular diseases. Economically, pollution can lead to decreased productivity due to health-related work absences and increased healthcare costs.
Addressing Urban Pollution
Addressing urban pollution necessitates a multi-pronged approach. Regulatory measures, such as enforcing strict emission standards for industries and vehicles, can help reduce air pollution. Improved waste management systems can help mitigate land pollution, while stricter regulations on waste disposal can help curb water pollution.
Public awareness and education are also crucial in combatting urban pollution. People need to understand the impacts of their actions on the environment and be encouraged to adopt greener lifestyles. Technological innovations, such as renewable energy sources, electric vehicles, and sustainable waste management solutions, can also play a vital role in mitigating urban pollution.
If you’re looking for more, here are essays on other interesting topics:
Apart from these, you can look at all the essays by clicking here .
Leave a Reply Cancel reply
Save my name, email, and website in this browser for the next time I comment.
Talk to our experts
1800-120-456-456
- Pollution Due to Urbanisation Essay
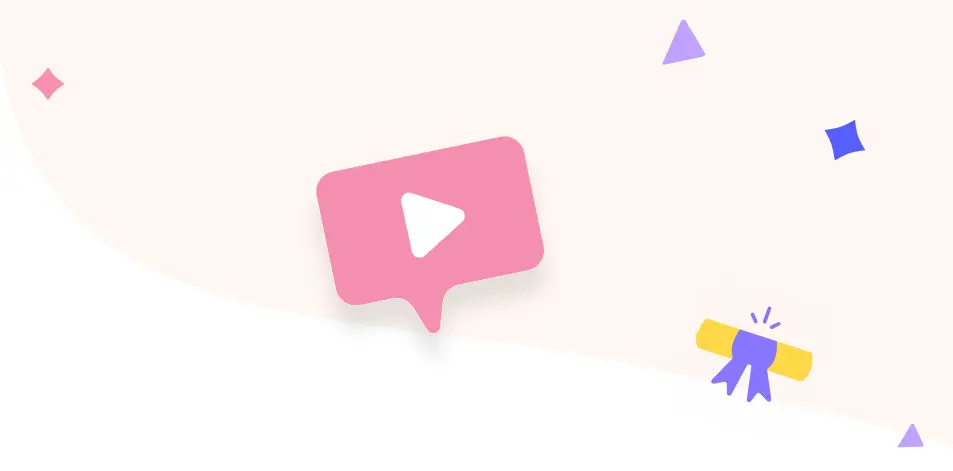
Essay on Pollution Due to Urbanisation
Below, you will find an essay on pollution due to urbanisation (long) and also a short essay on pollution due to urbanisation. While urbanisation has its positives, it is imperative to look at every object according to its pros and cons. Here are two essays on pollution due to urbanisation of 400-500 words and 100-200 words, respectively. We will discuss the importance of urbanisation for countries, and how urbanisation is polluting the world.
Long Essay on Pollution Due to Urbanisation
Urbanisation is a great concept which is required to develop any country. It refers to the concept of urbanising remote areas by building infrastructure which then brings about development. Infrastructure refers to all the buildings and institutions which are necessary for economic development to take place in an area. For example, educational institutions like schools, colleges, vocational learning centres are part of the infrastructure. Healthcare facilities such as hospitals and clinics, employment opportunities, food security, etc. are also part of the infrastructure of a country.
It is seen very often that a big corporation sets up shop in a rural area, and around this, infrastructure is built, and development and urbanisation take place. Jamshedpur is an example of such a place, where Tata Industries set up shop many years ago and made the area highly developed. Thus, urbanisation definitely encourages the people of a place to have a better life by giving them more opportunities to achieve good life through education, jobs, etc.
On the other hand, it must be duly noted that urbanisation is one of the leading causes of pollution in today’s world. There are several different kinds of pollution, such as air pollution, water pollution, soil pollution and noise pollution. The facets of urbanisation contribute to each one of these types of pollution in one way or another. Factories and mines contribute to air pollution through the fumes that each of them emits into the air. The damage done to the water and soil around factories because of their flowing septic is harmful to both humans as well as aquatic life. Additionally, the noises that come from mines, the whirring of machinery in factories, etc. contribute to noise pollution.
Additionally, it is not only big industries that contribute to pollution due to urbanisation. Part of urbanisation is also the development of roads, which means more cars, buses, two-wheelers, three-wheelers, trucks, etc. on the road. These all contribute to noise pollution because of the incessant honking, and also to air pollution, because of the fumes that all motor vehicles emit. Even when we are stuck in traffic in an auto, it becomes difficult to breathe because of the fumes which surround us on the roads. If we are finding it difficult to breathe, imagine what so many fumes are doing to our planet.
Short Essay on Pollution Due to Urbanisation
150 Words Paragraph On Pollution Due to Urbanisation
Pollution takes place when air, water or soil becomes contaminated with unwanted substances. Air pollution takes place because of the fumes of factories and motor vehicles on th e road. Soil pollution and water pollution take place due to the septic waste being released into soil or water that surrounds a factory. Even oil spills are a major reason for water pollution, and all kinds of pollution can be very dangerous for living beings. Another type of pollution is noise pollution, which comes from the honking of cars, loud sounds in factories, the passing of aeroplanes and trains, etc.
Urbanisation is a result of the need to achieve economic development. It refers to when a relatively rural or remote area is made more urban by constructing roads, hospitals, schools, offices, etc. In this way, development is a result of urbanisation, which is extremely good for all countries.
However, all the great factors that urbanisation brings in, such as factories to work in, motor vehicles to drive, and so much more, all of these contribute to pollution more and more. Even though urbanisation is very important for a country, it is important to address all the kinds of pollution
Pollution is one of the most pressing concerns confronting our civilization today. When their environment deteriorates on a daily basis, humans face major challenges. The mixing of any toxic element or contaminants in our natural environment is referred to as pollution. Many contaminants are introduced into the natural environment as a result of human activities, contaminating it too dangerous proportions. Pollution is caused by a variety of factors, one of which is urbanisation.
The negative aspect of urbanisation is the manufacturers, which emit a great deal of pollution. Their equipment emits smoke into the environment, pollutes water streams and the surrounding land, and makes a lot of noise. As a result, there is a lot of pollution as a result of urbanisation, and it is extremely destructive to the environment when it first begins.
The majority of the pollution in our environment is due to urbanisation. It's because factories are springing up all over the place, there are a lot more cars on the road now, and so on.
Pollution Due to Urbanisation
Our mother planet is choking, and we are unable to do anything about it. Today, we confront several issues, one of which is pollution. Pollution occurs when a contaminating substance is introduced into our environment and pollutes our natural resources. There are numerous causes of pollution, most of which are caused by humans. Natural resources and habitats have been depleted as a result of our activities.
Urbanisation is one of the primary causes of human pollution. Pollution levels began to rise when humans began to construct cities and industrialization developed. Human needs continue to expand, and we loot our mother planet to meet them. As a result of development, many beautiful valleys, mountains, hilltop stations, and woods have become pollution carriers. Trees have been felled, rivers and lakes have been poisoned, and natural reserves have been exploited.
As a result, we now live in severely polluted cities where daily life has become increasingly challenging. As a result of urban pollution, we are experiencing a variety of health issues, the worst part of which is that we are fully unconscious of it. It is past time for us to take steps to reduce pollution and make the world a better place for future generations.
Urbanisation is a really great step forward for any country, and it is and should be the main aim of all countries. All people around the world should have access to proper healthcare, education, sanitation, nourishment and safety, and urbanisation is how we can help achieve this goal. However, in the process of meeting this goal, we cannot forget that pollution due to urbanisation does take place, and is very dangerous for the planet and, therefore, all species living on earth in the long run.
FAQs on Pollution Due to Urbanisation Essay
1. What are the pros and cons of urbanisation according to the essay on pollution due to urbanisation?
The essay on pollution due to urbanisation says that urbanisation is good and is vital for a country, but can also be harmful for the environment. Urbanisation brings in better education, better healthcare facilities, better roads, and better infrastructure in general. However, it improves the lifestyles of human beings at the cost of hurting the environment by putting more contaminants into air, water and soil in the form of toxic fumes and septic waste. Thus, urbanisation is important, but it has to be brought about in a more sustainable manner.
2. How can we reduce pollution due to urbanisation?
At the individual level, there are some very simple ways to reduce pollution due to urbanisation. To reduce air pollution, we can choose to walk, carpool, or use public transport instead of taking a taxi. Garbage should not be thrown on roads and in water bodies, in order for us to stop soil and water pollution. We should also not honk on roads unnecessarily, to curb noise pollution. Unless the big companies and industries do not decide to take a stand and do what’s good for the environment, we will have to keep relying only on individual measures.
3. What are the different types of pollution and their causes?
Pollution in Cities: Types and Causes
Air Pollution: The air in metropolitan places is constantly polluted with harmful compounds, making breathing increasingly dangerous. The air in cities is suffocating. The air is polluted by smoke from autos, factories, and power plants. There are also other contaminants in the air, such as chemical spills and other harmful substances.
Water Pollution: Natural water supplies are becoming increasingly scarce in metropolitan areas, and those that do exist are becoming progressively contaminated. There is a lot of waste dumping in lakes and rivers, such as residential and industrial waste. A lot of trash is washed into the rivers when it rains.
Soil Pollution: Toxic mixtures in the soil are causing ecosystem disruption.
Noise Pollution: Cities are among the noisiest places on the planet. Noise pollution is caused by a variety of sources, including traffic noises, loudspeakers, and other undesirable noises, which cause a variety of health problems.
Radioactive Pollution: Nuclear power facilities' unintentional leaks represent a serious concern.
Visual Pollution: Signs, billboards, screens, high-intensity lights, and other forms of overexposure to sights in cities can also be highly unsettling.
There is also ' Thermal pollution ,' which is created by an excess of heat trapped in the earth's atmosphere.
4. How can pollution due to urbanisation be controlled?
One can implement the following methods to reduce pollution caused by urbanisation:
Conserve Energy: People in urban areas always use more energy than people in rural areas. The use of energy results in numerous types of pollution. One of the most effective strategies to reduce pollution is to conserve energy wherever possible. When you are not using an electrical appliance, turn it off. This tiny step can make a tremendous difference.
Reduce water waste: We waste a lot of water on a daily basis, which might have negative implications. We must make every effort to utilize as little water as possible.
Plant more trees: Urban areas are the ones with the least amount of greenery. It's a good idea to have a kitchen garden and a little lawn near your house.
Green belts: The government can assist by declaring specific sections in each city as green belts, allowing trees and other plants to flourish freely.
Use fewer loudspeakers: Using fewer loudspeakers can significantly minimise noise pollution. It's also a good idea to turn down the music level at functions after a specific amount of time has passed.
Indoors: In cities, home interiors are likewise heavily contaminated. We must also have some plants inside our homes to filter the polluted indoor air.
Industrial trash: Factory owners must make every effort to avoid dumping industrial waste in lakes or rivers. The government can also enact legislation in this regard.
5. What problems are caused due to Urbanization?
The necessity for open space to develop roads, buildings, and bridges, among other things, resulted in widespread deforestation. To accommodate the ever-increasing population, trees were cut down, fields were cleared, and built new space. It goes without saying that tree cutting is a major source of pollution. The high population density resulted in a scarcity of everything, including space and natural resources such as water and coal.
A number of serious challenges have arisen as a result of the interaction of the urban population with the environment. The spending habits and lifestyles of the urban people had a significant impact on the environment. Consumption of food, energy, and water is all higher in cities. Cities have much more filthy air than rural areas. This is mainly due to the increased use of automobiles and the expansion of industries and factories that pollute the air. We utilise electricity to power almost all of our equipment.
6. What is urbanisation, and how is it caused?
The population shift from rural to urban regions, the resulting decline in the number of people living in rural areas, and the methods in which societies adjust to this transition are all referred to as urbanisation. It is basically the process by which towns and cities evolve and grow as more people choose to live and work in central locations.
Individual, community and state activity result in either organic or planned urbanisation. Living in a city can be culturally and economically advantageous since it can provide more options for access to the labour market, better education, housing, and safety conditions, as well as lower commute and transit time and costs. A healthy urban environment is characterised by density, proximity, diversity, and marketplace rivalry. However, there are also negative social consequences associated with urban living, such as alienation, stress, higher living costs, and mass marginalisation. Suburbanization, which is occurring in the greatest developing countries' cities, can be seen as an attempt to balance these negative aspects of city living while still giving access to a huge number of shared resources.
7. What is the Impact of Urbanisation in Indian Cities?
The following are the main effects of urbanisation on environmental quality in Indian cities:
According to the entire slum population in India in 1991, 41 per cent of the overall slum population lived in cities with populations of one million or more, which account for 27 percent of the country's total population.
According to the current situation of municipal solid trash creation and collection situation in Indian metropolitan cities, Maharashtra creates the most municipal solid garbage (11,000 tonnes per day), followed by Delhi (8700 tonnes per day) in 2019, both of which are expected to rise in the near future.
In India and other Metropolitan Cities, the number of automobiles on the road is increasing.
In India and other metropolitan cities, the number of automobiles on the road has increased. The usage of vehicles has increased by 10% or more on average, posing a significant threat to air pollution.
Water resources are dwindling day by day as a result of rising population, wasteful usage, and a lack of conservation. Huge amounts of wastewater enter rivers as cities and industries grow, contaminating river streams that are used for drinking and other reasons.
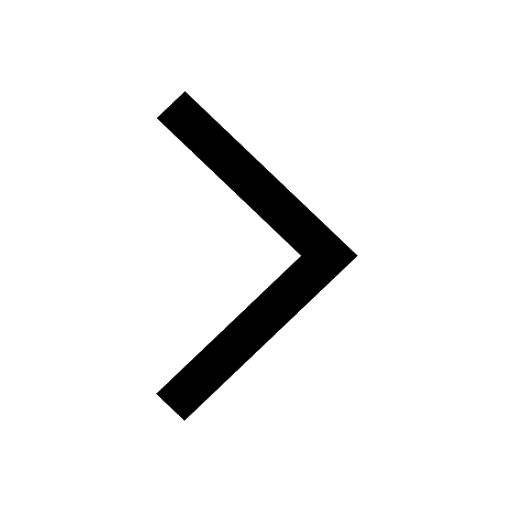
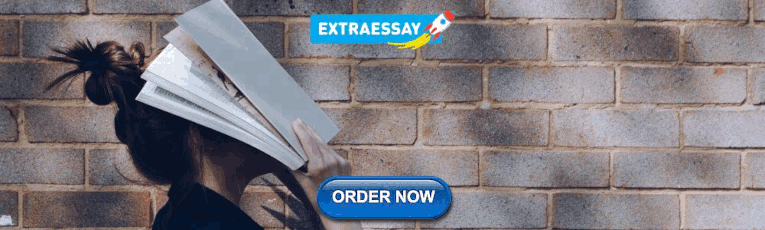
IMAGES
VIDEO
COMMENTS
Among all types of ecosystems, urban produce roughly 78% of carbon emissions and substantial airborne pollutants that adversely affect over 50% of the world’s population living in them 5, 10 ...
Pollution is an important determinant of urban quality of life. Households have flocked to cities over the last centuries and decades, attracted by agglomeration economies, such as higher productivity and wages.
Understanding the perplexing relationship between air pollution and urban forms and tackling air pollution in conjunction with climate and public health issues can result in substantial progress towards sustainable urbanization.
Urban development can magnify the risk of environmental hazards such as flash flooding. Pollution and physical barriers to root growth promote loss of urban tree cover.
I. Air Pollution in Urban Areas. One of the most visible and alarming consequences of urbanization is air pollution. The concentration of industries, transportation, and residential areas in cities has led to increased emissions of pollutants into the atmosphere.
Essay on Pollution Due To Urbanization: Urbanization has brought about numerous benefits such as economic growth and improved living standards. However, it has also led to a significant increase in pollution levels in urban areas.
Most of the global population will live in urban areas in the 21st century. We study impacts of urbanization on future river pollution taking a multi-pollutant approach.
For the first time, we provide both observation-based and model-based trends with a concrete estimation of the magnitude per country at global scale. In this study, we hypothesized that short-term changes over 20-year time-series are attributed to emissions changes.
Pollution in cities has emerged as a significant concern, causing detrimental effects on both human health and the environment. Rapid urbanization, industrialization, and overpopulation have exacerbated the issue, making it a pressing challenge for the modern world.
Here are two essays on pollution due to urbanisation of 400-500 words and 100-200 words, respectively. We will discuss the importance of urbanisation for countries, and how urbanisation is polluting the world.