- Open access
- Published: 03 February 2020
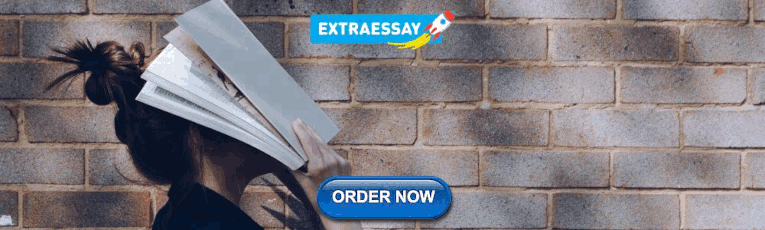
Effectiveness of wastewater treatment systems in removing microbial agents: a systematic review
- Zahra Aghalari 1 ,
- Hans-Uwe Dahms 2 , 3 , 4 ,
- Mika Sillanpää 5 ,
- Juan Eduardo Sosa-Hernandez 6 &
- Roberto Parra-Saldívar 6
Globalization and Health volume 16 , Article number: 13 ( 2020 ) Cite this article
47k Accesses
75 Citations
2 Altmetric
Metrics details
Due to unrestricted entry of wastewater into the environment and the transportation of microbial contaminants to humans and organisms, environmental protection requires the use of appropriate purification systems with high removal efficiency for microbial agents are needed. The purpose of this study was to determine the efficacy of current wastewater treatment systems in removing microbes and their contaminants.
A systematic review was conducted for all articles published in 5 Iranian environmental health journals in 11 years. The data were collected according to the inclusion and exclusion criteria and by searching the relevant keywords in the articles published during the years (2008–2018), with emphasis on the efficacy of wastewater treatment systems in removing microbial agents. Qualitative data were collected using a preferred reporting items for systematic reviews and meta-analyzes (PRISMA) standard checklist. After confirming the quality of the articles, information such as the name of the first author and the year of publication of the research, the type of study, the number of samples, the type of purification, the type of microbial agents and the rate of removal of microbial agents were entered into the checklist. Also the removal rates of the microbial agents mentioned in the studies were compared with united states environmental protection agency (US-EPA) standards.
In this study, 1468 articles retrieved from 118 issues of 5 environmental health journals were reviewed. After reviewing the quality of the articles in accordance with the research objectives, 14 articles were included in the study that were published between 2010 and 2018. In most studies, two main indicators Total coliforms and Fecal coliforms in wastewater were investigated. Removing fungi and viral contamination from wastewater was not found in any of the 14 studies. Different systems (activated sludge, stabilization ponds, wetlands, and low and medium pressure UV disinfection systems were used to remove microbial agents in these studies. Most articles used active sludge systems to remove Total coliforms and Fecal coliforms , which in some cases were not within the US-EPA standard. The removal of Cysts and Parasitic eggs was only reporte from stabilization pond systems (SPS) where removal efficiency was found in accordance with US-EPA standards.
Conclusions
Different types of activated sludge systems have higher efficacy to remove microbial agents and are more effective than other mentioned systems in removing the main indicators of sewage contamination including Total coliforms and Fecal coliforms . However, inappropriate operation, maintenance and inadequate handling of activated sludge can also reduce its efficiency and reduce the removal of microbial agents, which was reported in some studies. Therefore, it is recommended to conduct research on how to improve the operation, maintenance, and proper management of activated sludge systems to transfer knowledge to users of sludge systems and prevent further health issues related to microbial agents.
Introduction
Due to hazardous impacts of municipal, industrial and hospital wastewater on water, soil, air and agricultural products, wastewater treatment and the proper disposal of the sludge produced are indispensable from an environmental safety point of view [ 1 , 2 ]. Economically, effective wastewater treatment has important effects on saving water, and preventing unnecessary water losses [ 3 ]. In arid and semiarid countries such as Iran, the water demand has increased and annual rainfall is low also in regions of North Africa, Southern Europe, and in large countries such as Australia and the United States. Consequently, reuse of sewage is the most sustainable and long-term solution to the problem of water scarcity [ 4 , 5 ]. In the next 30 years, the world’s population will increase by more than double. Due to population growth, the amount of water available in 1960 was reduced to 3300 cubic meters and in 1995 it was reduced to 1250 cubic meters. This trend is projected to decrease to 650 cubic meters worldwide by 2025 [ 6 ]. Due to this water shortage crisis, water from wastewater treatment need to be reused increasingly in the near future [ 6 ]. Wastewater reuse requires treatment and application of appropriate wastewater treatment systems [ 7 ]. In recent years, increased research has been done on wastewater treatment using simple, low-cost, easy-to-use methods in developing countries [ 8 , 9 ]. Systems and processes such as activated sludge, aerated lagoons, stabilization ponds, natural and synthetic wetlands, trickling filters, rotating biological contactors (RBCs) have been used for wastewater treatment and removal of physical, chemical and biological contaminants [ 10 , 11 ]. Among different contaminants of wastewater, microbial agents becoming increasingly important and their removal efficiency should be reported in different wastewater treatment systems [ 12 , 13 ]. Biological contaminants in wastewater are different types of bacteria ( Fecal coliforms and Escherichia coli , Salmonella , Shigella , Vibrio cholerae ), diverse Parasite cysts and eggs , viruses and fungi. All of them can be hazardous to environmental and human health depending on the type and amount [ 14 , 15 ]. For example, bacteria in wastewater cause cholera, typhoid fever, and tuberculosis, viruses can cause hepatitis, and protozoa can cause dysentery [ 16 , 17 ]. Many microbial agents attached to suspended solids in wastewater if inadequately treated and wastewater discharge into the environment, such as river water, green space, and crops, put humans and aquatic organisms at risk [ 18 , 19 ]. Therefore, utilization of appropriate wastewater treatment systems tailored to a variety of microbial agents is essential to achieve as complete as possible elimination of biological agents. For example, in the study of Sharafi et al., (2015) with the aim of determining the removal efficiency of parasites from wastewater using a wetland system, the removal rates of protozoan cysts and Parasite eggs were 99.7 and 100%, respectively [ 20 ]. Okoh, et.al. (2010) reported that activated sludge processes, oxidation pools, activated carbon filtration, lime and chlorination coagulation eliminated removed 50–90% of wastewater viruses [ 21 ]. Wastewater from wastewater treatment plants, is used in Iran without restrictions and controls like in many other countries. Therefore, it is necessary to employ proper sewage treatment systems, before water can be publicly used such as for irrigation. This study is focusing on the efficacy of different wastewater treatment systems in removing microbial agents.
Study protocol
This systematic review study was carried out to determine the efficacy of wastewater treatment systems in the removal of microbial agents (bacteria, parasites, viruses, and fungi) by searching all articles published in 5 Iranian Journals of Environmental Health. The data were collected by referring to the specialized site of each journal, from the beginning of 2008 to the latest issue of 2018. Reviewed journals included; Iranian Journal of Health and Environment (IJHE), Journal of Environmental Health Engineering (JEHE), Journal of Research in Environmental Health (JREH), and two English-language journals, Environmental Health Engineering and Management Journal (EHEMJ), Journal of Environmental Health Science and Engineering (JEHSE).
Search strategy
Inquired information was collected by searching for keywords on the sites of Iranian specialty health journal. Key words included; ‘waste water’ OR ‘waste-water’ OR ‘wastewater treatment’ OR ‘effluent’ OR ‘sewage’ OR ‘sewage treatment’ OR ‘sewage disposal’ OR ‘wastewater disposal’ AND ‘treat’ OR ‘remove’ AND ‘microb’ AND ‘pathogen’ AND ‘bacteria’ AND ‘virus’ AND ‘parasite’ AND ‘FCs’ OR ‘Fecal coliforms ’ AND ‘Iran’.
A manual search was performed by checking all published articles. This way, the abstracts of all published articles were reviewed over the period of 11 years between 2008 and 2018.
Inclusion criteria
Inclusion criteria for this study included the year of publication, type of wastewater samples (municipal wastewater, domestic wastewater, hospital wastewater), number of samples (more than 5 wastewater samples), treatment procedures (different types), state the required and mention the type of purification (type of treatment, type of microbial agents, amount or percentage of microbial agents removed).
Exclusion criteria
Exclusion criteria for this study were: lack of access to the full article, inappropriate subject matter, inadequacy of the method of treatment and purification, lack of expression of the type of microbial agents removed, review studies, and letters to the editor.
Quality assessment articles
This study is based on standard checklist PRISMA (Preferred Reporting Items for Systematic Reviews and Meta-analyzes). The US-based National Institute of Health Quality Assessment Tool for Observational Cohort and Cross-Sectional Studies [ 22 ] for qualitative studies was used. This checklist is made based on the following criteria: Yes, No, cannot determine, Not applicable, and Not reported. It has eliminated the scoring problems. The checklist included 14 questions that were used for research purposes, samples, inclusion and exclusion criteria, findings, results and publication period of each of the 14 articles (Table 1 ).
Extract information from articles
In order to extract information, all articles were evaluated independently by two reviewers based on inclusion and exclusion criteria. Both reviewers eventually summarized the information and in cases where the information was inconsistent a third reviewer’s comments was used. The information extracted from the articles was included in the researcher’s checklist for qualitative approval and used in other prior author studies of this paper [ 23 , 24 , 25 ]. The checklist included the name of the first author, the year of publication of the research, the type of study, the number of samples, the type of purification, the type of microbial agents and the rate of microbial removal. Additionally, the removal rates of the microbial agents mentioned in the studies were compared with US-EPA standards [ 26 , 27 ] (Table 2 ).
Search results
In this study, 1468 articles related to 118 issues of 5 environmental health journals were reviewed. In the first phase of the search process, 216 articles on wastewater treatment were identified. Then, 196 inappropriate and irrelevant articles were excluded for the purpose of the study. Finally, after reviewing the information and quality of the articles, 14 articles were eligible for systematic review (Fig. 1 ).
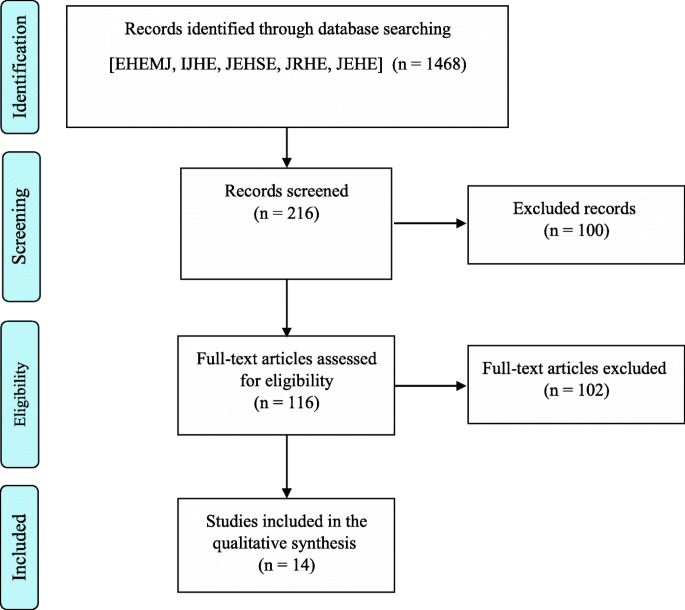
Flowchart describing the study design
Descriptive results of articles
Of the 14 articles reviewed, the largest number of articles (9 articles; 64.2%) were published between 2014 and 2018. Most of the experiments were carried out on wastewater samples in Tehran (28.58%). In total, studies were conducted in 10 cities of Iran (Fig. 2 ).
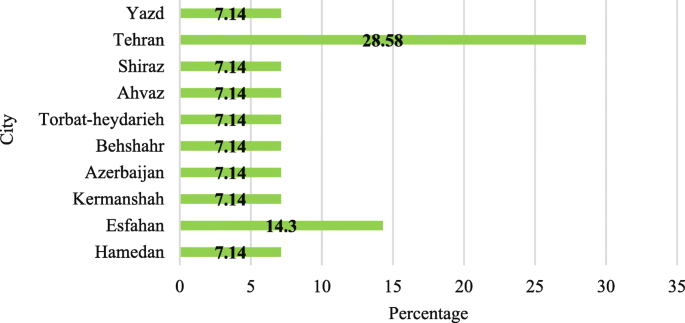
Cities selected for wastewater sampling in 14 articles
Concerning the type of microbial agents, it was found that a total of 14 articles have eliminated types of bacteria and parasites from municipal, hospital and industrial wastewater (Fig. 3 ). In 11 articles, two main microbial indices ( Total coliforms and Fecal coliforms ) were used as bioindicators to evaluate the efficacy of the wastewater treatment systems (Fig. 3 ).
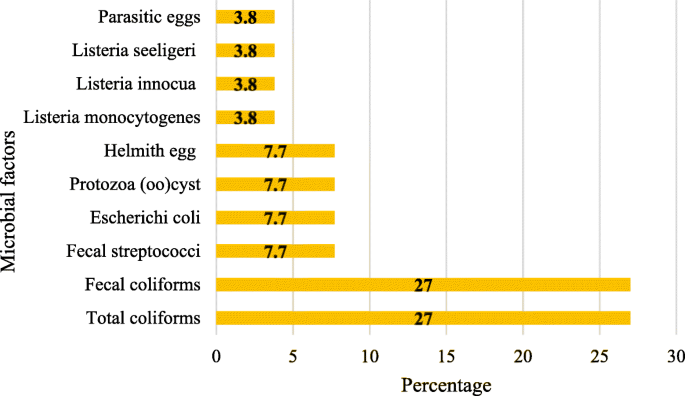
Types of microbial agents removed in wastewater based on the articles
Quality assessment of articles
The qualitative results of the articles showed that most of the studies were of good quality but in many articles the method of determination of sample size (Q5) was not specified. In the articles, participation rate of eligible persons, inclusion and exclusion criteria, exposure (s) were evaluated more than once, and blinding of participant exposure status was not relevant and not applicable (Q10, Q4, Q3 and Q12) (Table 3 ).
Article features
Articles on the efficacy of a variety of purification systems for the removal of microbial agents were published between 2010 and 2018. All studies don in the laboratory. The largest sample size was related to Derayat et al., 2011 [ 30 ] in Kermanshah with 120 wastewater samples. Wastewater studies were carried out in different cities of North, East, West and Central Iran. Most studies have investigated bacterial factors in wastewater and the efficacy of removing fungi and viral contamination in wastewater was not found in any study (Table 4 ). In most articles, the type of sewage treatment system was activated sludge. For example were the removal rates of microbial agents in wastewater investigated in the study by Derayat et al., 2011 [ 30 ], Baghapour et al., 2013 [ 31 ] and Nahavandi et al., 2015 [ 37 ] on Conventional Activated Sludge, Ghoreishi et al., 2016 [ 38 ] on extended aeration activated sludge (Table 4 ).
Evaluation of the removal of microbial agents in accordance with US-EPA standards showed that in some articles the removal of Total coliforms and Fecal coliforms was not within acceptable ranges. For example, in the study of Ghoreishi et al., 2016 [ 38 ], although several different systems were used to remove Total coliforms, eimination efficiency never reached US-EPA standards. Moreover, the activated sludge process did not have the efficiency to remove Parasitic eggs as reported in the study by Nahavandi et al., 2015 [ 37 ] (Table 4 ).
Examination of microbial removal rates in the study of Ghoreishi et al., 2016 [ 38 ] that none of the Total Coliforms removal was US-EPA standard although both extended aeration activated sludge and conventional activated sludge systems were used to remove Total coliforms . The US-EPA standard for Total coliforms removal is 1000 MPN/100 mL, and wastewater showing this amount of Total coliforms is capable of being discharged into the receiving waters [ 26 , 27 ]. A study by Paiva et al., 2015 on domestic wastewater in tropical Brazil also showed that removal of Total coliforms through the use of activated sludge was not a desirable remediation method [ 42 ]. The reason for the poor performance of activated sludge to remove Total coliforms can be attributed to factors such as management problems and operation of the activated sludge system, which results in the production of bulk waste and sludge. This problem is one of the most important disadvantages of activated sludge systems and should be addressed once a month by experienced staff and monitoring experts to correct it. Overall, different activated sludge systems are the best choice for this type of wastewater due to the amount of municipal wastewater pollutants because of high purification efficiency to reduce biochemical oxygen demand (BOD 5 ) [ 43 , 44 ].
Removal of Cysts and Parasitic eggs in the study of Derayat et al., (2011), which used stabilization pond systems, was reported as being in accordance with US-EPA standards [ 30 ]. A study by Amahmid et al. (2002) aimed at the treatment of municipal wastewater with a stabilized pond system in Morocco showing that Cyst and Parasitic egg removal efficiency was 100% and that the pond system showed a proper performance [ 45 ]. A large number of stabilized pond systems were been constructed and used in countries such as the United States, New Zealand, India, Pakistan, Jordan and Thailand [ 3 ]. In Iran, a number of these systems were constructed for the treatment of wastewater in Arak, Gilan West and Isfahan [ 46 ]. Stabilization ponds have a high acceptability due to their simplicity of operation, and lack of mechanical and electrical equipment compared to other sewage treatment systems, their high efficiency in removing pathogenic organisms [ 47 ]. A major drawback for stabilization ponds is the need for extensive land, the low quality of effluents due to the presence of algae, and odor production that limits the use of this type of treatment system near habitated areas. To improve the quality of resulting effluents, chemical compounds need to be consolidated, such as by coagulation and the application of microstrainers, stabilization ponds and rock filters [ 47 , 48 ].
As for wetlands by Karimi et al. (2014) on Fecal coliforms , Escherichia coli and Fecal streptococci show that wetlands did not perform well to remove microbial agents (removal rate for Fecal coliforms 1.13 × 1014 MPN/100 mL and Escherichia coli 5.03 × 1012 MPN/100 mL) [ 34 ]. In a study by Decamp et al. (2000), the mean removal of Escherichia coli through the wetland was 41 to 72% at the in situ scale and 96.6 to 98.9% at the experimental scale [ 49 ]. In the study of Evanson et al. (2006), Fecal coliforms removal rate was 82.7 to 95.99% [ 50 ]. Removal of Total coliforms and Fecal coliforms in the wetlands is done by various biological factors such as nematodes, protozoa, bacterial activity, bacteriophage production, chemical factors, oxidation reactions, bacterial uptake and toxicity [ 51 ] and the interference in each of these (microbial communities) will affect the rate of removal of Total coliforms and other microbial agents. Removal of pathogens such as Escherichia coli and Cryptosporidium was also performed in wetlands but is often not in compliance with environmental standards [ 52 ]. In addition, although wetlands are economical and widely used in wastewater treatment systems because of easy to operate, maintain, and operate at a low price [ 53 , 54 , 55 ], but they don’t seem to be a good option for removing all of the microbial agents.
In a study by Hashemi, et.al. (2010) on UV disinfection system included low pressure (LP) and UV disinfection system including medium pressure (MP) to remove Total coliforms , Fecal coliforms and Fecal streptococci. All investigated microbial agents were completely eliminated [ 28 ]. However, it was reported that the direct disinfection of secondary effluents with LP and MP systems and even their integration due to high concentrations of suspended solids was not possible. Therefore, disinfection of wastewater with UV irradiation requires higher effluent quality through improved system utilization or application of an advanced treatment plant prior to disinfection [ 28 ]. In 1988, about 300 and in 2004 about 4300 sewage treatment plants in the United States, (that are more than 20% of filtration plants) used a UV system for wastewater disinfection. The number of wastewater treatment plants having UV systems has increased in the US, Europe and East Asia. This trend is expected to expand further in the coming decades. Although the use of UV radiation for wastewater disinfection has many potential advantages, it also has disadvantages in terms of cost, lamp deposition, and the possible reactivation of targeted pathogenic microorganisms after treatment [ 56 ]. Wastewater treatment professionals should therefore be aware of new replacement processes and perform pilot scale assessments prior to changing treatment processes.
One of the strengths of this study is addressing the efficacy of wastewater treatment systems by comparing the removal efficiency of various microbial agents that have received little attention as yet. In most studies, only one type of system for removing different physical, chemical and microbial contaminants in a single type of wastewater was investigated and it was not possible to compare the removal efficiency of microbial agents. One of the limitations of this study was the lack of reviewing published articles on wastewater treatment systems in other than the 5 Iranian journals. This limitation, however, might be negligible because the research on wastewater treatment was done by environmental health professionals. Therefore, most studies in this area are published in specialized environmental health journals.
Different types of activated sludge systems have better efficacy to remove microbial agents and are more effective than other systems in removing the main indicators of sewage contamination including Total coliforms and Fecal coliforms . However, inappropriate operation, maintenance and inadequate handling of activated sludge can also reduce the efficiency of microbial agent removal, which has been reported in some studies. Therefore, it is recommended to conduct research on how to increase the operation, maintenance and proper management of activated sludge systems and provide the results to utility personnel responsible to work with this system in order to correct the system quality output in a timely manner. In future research, it is recommended that employed treatment methods integrate two or more purification systems, which then could more effectively remove microbial agents. Additionally, the reports of removal efficiency should include each of the indicated microbes so that health and environmental professionals can make better decisions about using the systems or prevent future eventualities.
Availability of data and materials
Not applicable.
Abbreviations
Anaerobic baffled reactor
Biochemical Oxygen Demand
Environmental Health Engineering and Management Journal
Fluidized Bed Reactor
Iranian Journal of Health and Environment
Journal of Environmental Health Engineering
Journal of Environmental Health Science and Engineering
Journal of Research in Environmental Health
Low pressure
Medium pressure
Most Probable Number
Preferred Reporting Items for Systematic Reviews and Meta-analyzes
Rotating Biological Contactors
Stabilization Pond Systems
United States Environmental Protection Agency
Ultraviolet
Qu X, Zhao Y, Yu R, Li Y, Falzone C, Smith G, Ikehata K. Health effects associated with wastewater treatment, reuse, and disposal. Water Environ Res. 2016;88(10):1823–55. https://doi.org/10.2175/106143016X14696400495776 .
Article CAS PubMed Google Scholar
Choudri BS, Charabi Y, Ahmed M. Health effects associated with wastewater treatment, Reuse and Disposal. Water Environ Res. 2018;90(10):1759–76. https://doi.org/10.2175/106143018X15289915807425 .
Zhang QH, Yang WN, Ngo HH, Guo WS, Jin PK, Dzakpasu M, Yang SJ, Wang Q, Wang XC, Ao D. Current status of urban wastewater treatment plants in China. Environ Int. 2016;92-93:11–22. https://doi.org/10.1016/j.envint.2016.03.024 .
Nzila A, Razzak SA, Zhu J. Bioaugmentation: an emerging strategy of industrial wastewater treatment for reuse and discharge. Int J Environ Res Public Health. 2016;13(9):846. https://doi.org/10.3390/ijerph13090846 .
Article CAS PubMed Central Google Scholar
Norton-Brandao D, Scherrenberg SM, van Lier JB. Reclamation of used urban waters for irrigation purposes—a review of treatment technologies. J Environ Manag. 2013;122:85–98. https://doi.org/10.1016/j.jenvman.2013.03.012 .
Article CAS Google Scholar
Abdel-Raouf N, Al-Homaidan AA, Ibraheem IB. Microalgae and wastewater treatment. Saudi J Biol Sci. 2012;19(3):257–75. https://doi.org/10.1016/j.sjbs.2012.04.005 .
Article CAS PubMed PubMed Central Google Scholar
Jaffar Abdul Khaliq S, Ahmed M, Al-Wardy M, Al-Busaidi A, Choudri BS. Wastewater and sludge management and research in Oman: an overview. J Air Waste Manag Assoc. 2017;67(3):267–78. https://doi.org/10.1080/10962247.2016.1243595 .
Kelessidis A, Stasinakis AS. Comparative study of the methods used for treatment and final disposal of sewage sludge in European countries. Waste Manag. 2012;32(6):1186–95. https://doi.org/10.1016/j.wasman.2012.01.012 .
Masciandaro G, Iannelli R, Chiarugi M, Peruzzi E. Reed bed systems for sludge treatment: case studies in Italy. Water Sci Technol. 2015;72(7):1043–50. https://doi.org/10.2166/wst.2015.309 .
Chen HJ, Lin YZ, Fanjiang JM, Fan C. Microbial community and treatment ability investigation in AOAO process for the optoelectronic wastewater treatment using PCR-DGGE biotechnology. Biodegradation. 2013;24(2):227–43. https://doi.org/10.1007/s10532-012-9579-0 .
Zhang B, Yu Q, Yan G, Zhu H, Xu XY, Zhu L. Seasonal bacterial community succession in four typical wastewater treatment plants: correlations between core microbes and process performance. Sci Rep. 2018;8(1):4566. https://doi.org/10.1038/s41598-018-22683-1 .
Wang M, Shen W, Yan L, Wang XH, Xu H. Stepwise impact of urban wastewater treatment on the bacterial community structure, antibiotic contents, and prevalence of antimicrobial resistance. Environ Pollut. 2017;231(Pt 2):1578–85. https://doi.org/10.1016/j.envpol.2017.09.055 .
Park JH, Kim YJ, Binn- K, Seo KH. Spread of multidrug-resistant Escherichia coli harboring integron via swine farm waste water treatment plant. Ecotoxicol Environ Saf. 2018;149:36–42. https://doi.org/10.1016/j.ecoenv.2017.10.071 .
Grandclément C, Seyssiecq I, Piram A, Wong-Wah-Chung P, Vanot G, Tiliacos N, Roche N, Doumenq P. From the conventional biological wastewater treatment to hybrid processes, the evaluation of organic micropollutant removal: a review. Water Res. 2017;111:297–317. https://doi.org/10.1016/j.watres.2017.01.005 .
Osuolale O, Okoh A. Human enteric bacteria and viruses in five wastewater treatment plants in the Eastern Cape, South Africa. J Infect Public Health. 2017;10(5):541–7. https://doi.org/10.1016/j.jiph.2016.11.012 .
Article PubMed Google Scholar
Ajonina C, Buzie C, Rubiandini RH, Otterpohl R. Microbial pathogens in wastewater treatment plants (WWTP) in Hamburg. J Toxicol Environ Health A. 2015;78(6):381–7. https://doi.org/10.1080/15287394.2014.989626 .
Jaromin-Gleń K, Kłapeć T, Łagód G, Karamon J, Malicki J, Skowrońska A, Bieganowski A. Division of methods for counting helminths' eggs and the problem of efficiency of these methods. Ann Agric Environ Med. 2017;24(1):1–7. https://doi.org/10.5604/12321966.1233891 .
Naidoo S, Olaniran AO. Treated wastewater effluent as a source of microbial pollution of surface water resources. Int J Environ Res Public Health. 2013;11(1):249–70. https://doi.org/10.3390/ijerph110100249 .
Okeyo AN, Nontongana N, Fadare TO, Okoh AI. Vibrio species in wastewater final effluents and receiving watershed in South Africa: implications for public health. Int J Environ Res Public Health. 2018;15(6):1266. https://doi.org/10.3390/ijerph15061266 .
Sharafi K, Moradi M, Azari A, Sharafi H, Pirsaheb M. Comparative evaluation of parasitic removal in municipal wastewater using constructed wetland and extended aeration–activated sludge system in Kermanshah province, Iran. Inter J Health Life Sci. 2016;2(1):16–21.
Article Google Scholar
Okoh AI, Sibanda T, Gusha SS. Inadequately treated wastewater as a source of human enteric viruses in the environment. Int J Environ Res Public Health. 2010;7(6):2620–37. https://doi.org/10.3390/ijerph7062620 .
National heart, lung, and blood institute. Study Quality Assessment Tools. Available from: https://www.nhlbi.nih.gov/health-topics/study-quality-assessment-tools (Accessed 30 July 2019).
Tirgar A, Sajjadi SA, Aghalari Z. The status of international collaborations in compilation of Iranian scientific articles on environmental health engineering. Glob Health. 2019;15(1):17. https://doi.org/10.1186/s12992-019-0460-3 .
Tirgar A, Aghalari Z. Scientific achievements of medical journals in occupational accidents. HDQ. 2018;3(4):179–84. https://doi.org/10.32598/hdq.3.4.179 .
Aghalari Z, Tirgar A. Topics of disasters in scientific outputs of medical sciences: a cross-sectional study. HDQ. 2017;2(2):47–52. https://doi.org/10.18869/nrip.hdq.2.2.47 .
USEPA. Land application of sewage sludge: A guide for land appliers on the requirements of the federal standards for the use or disposal of sewage sludge, 40 CFR Part 503. Washington DC: Office of Enforcement and Compliance Assurance, United States Environmental Protection Agency; 1994. Report No.: EPA/831-B-93-002b
Google Scholar
EPA. Environmental guidelines: Use and disposal of biosolids products. Sydney: Waters & Catchments Policy Section, Environmental Policy Branch, Environment Protection Authority; 2000. Report No.: EPA 97/62
Hashemi H, Amin M, Bina B, Movahedian Attar H, Farrokhzadeh H. Survey on possibility of Disinfection of Isfahan North Wastewater Treatment Plant Effluent by Low and Medium Pressure Ultraviolet Systems in Pilot ScaleSystems in Pilot Scale. Iranian J Health Environ 2010; 3 (1):47–58. http://ijhe.tums.ac.ir/browse.php?a_id=134&sid=1&slc_lang=en
Banejad H, Yazdani V, Rahmani A, Mohajeri S, Olyaie E. Possibility of Using Moringa Peregrina Seeds Compared with Alum and Poly Aluminum Chloride in Sewage Treatment. Iranian J Health Environ. 2010;3(3):251–60 http://ijhe.tums.ac.ir/browse.php?a_id=106&sid=1&slc_lang=en .
Derayat J, Almasi A, Sharafi K, Meskini H, Dargahi A. The Efficiency Comparison of Conventional Activated Sludge and Stabilization Pond Systems in Removal of Cysts and Parasitic Eggs (A case Study: Kermanshah and Gilangharb Wastewater Treatment Plants). Iranian J Health Environ. 2011;4(2):181–8 http://ijhe.tums.ac.ir/browse.php?a_id=74&sid=1&slc_lang=en .
Baghapour MA, Nasseri S, Djahed B. Evaluation of shiraz wastewater treatment plant effluent quality for agricultural irrigation by Canadian water quality index (CWQI). Iranian J Environ Health Sci Eng. 2013;10(1):27. https://doi.org/10.1186/1735-2746-10-27 .
Safari GH, Yetilmezsoy K, Mahvi AH, Zarrabi M. Post-treatment of secondary wastewater treatment plant effluent using a two-stage fluidized bed bioreactor system. J Environ Health Sci Eng. 2013;11(1):10. https://doi.org/10.1186/2052-336X-11-10 .
Navidjouy N, Jalali M, Khorsandi H, Movahedian H. Study of Sludge Processing Units Efficiency in North IsfahanWastewater Treatment Plant to Remove Listeria Species. Iranian J Health Environ. 2014;7(1):65–72 http://ijhe.tums.ac.ir/browse.php?a_id=5295&sid=1&slc_lang=en .
Karimi B, Ehrampoush MH, Jabary H. Indicator pathogens, organic matter and LAS detergent removal from wastewater by constructed subsurface wetlands. J Environ Health Sci Eng. 2014;12(1):52. https://doi.org/10.1186/2052-336X-12-52 .
Article PubMed PubMed Central Google Scholar
Aslani H, Nabizadeh R, Alimohammadi M, et al. Disinfection of raw wastewater and activated sludge effluent using Fenton like reagent. J Environ Health Sci Eng. 2014;12(1):149. https://doi.org/10.1186/s40201-014-0149-8 .
Jamshidi S, Akbarzadeh A, Woo KS, Valipour A. Wastewater treatment using integrated anaerobic baffled reactor and Bio-rack wetland planted with Phragmites sp. and Typha sp. J Environ Health Sci Eng. 2014;12(1):131. https://doi.org/10.1186/s40201-014-0131-5 .
Hatam-Nahavandi K, Mahvi AH, Mohebali M, Keshavarz H, Mobedi I, Rezaeian M. Detection of parasitic particles in domestic and urban wastewaters and assessment of removal efficiency of treatment plants in Tehran, Iran. J Environ Health Sci Eng. 2015;13:4. https://doi.org/10.1186/s40201-015-0155-5 .
Ghoreishi B, Aslani H, Dolatkhah A, Abdoli Seilabi A, Mosaferi M. Evaluation of Microbial Quality in Biosolids Generated from Municipal Wastewater Treatment Plants. Iranian J Health Environ. 2016;9(1):81–90 http://ijhe.tums.ac.ir/browse.php?a_id=5584&slc_lang=en&sid=1&printcase=1&hbnr=1&hmb=1 .
Mollaie Tavani S, Dehghanifard E, Hajibagher Tehrani S, Ebrahimi U. Survey the performance of the Shohada of Behshahr the wastewater treatment plant hospital in 2015–2016. J Environ Health Eng. 2017;4(2):161–73 http://jehe.abzums.ac.ir/browse.php?a_id=335&sid=1&slc_lang=fa .
Sasani H, Mehrdadi N, Aminzadeh B, Takdastan A. Baffle and fixed media effects on coliform removal and bacterial die-off rate coefficient in waste stabilization ponds (a case study in Ahvaz). Environ Health Eng Manag. 2017;4(3):177–84. https://doi.org/10.15171/EHEM.2017.25 http://ehemj.com/browse.php?a_id=280&sid=1&slc_lang=en .
Choopan Y, Emami S. Evaluation of physical, chemical and biologic properties of Torbat-Heydarieh’s municipal wastewater treatment Plant for Agricultural Uses. Iranian J Res Environ Health. 2018;4(3):227–36 http://jreh.mums.ac.ir/article_12026.html .
Paiva MC, Ávila MP, Reis MP, Costa PS, Nardi RM, Nascimento AM. The Microbiota and Abundance of the Class 1 Integron-Integrase Gene in Tropical Sewage Treatment Plant Influent and Activated Sludge. PLoS One. 2015;10(6):e0131532. Published 2015 Jun 26. https://doi.org/10.1371/journal.pone.0131532 .
Mannino I, Franco D, Piccioni E, Favero L, Mattiuzzo E, Zanetto G. A cost-effectiveness analysis of seminatural wetlands and activated sludge wastewater-treatment systems. Environ Manag. 2008;41(1):118–29. https://doi.org/10.1007/s00267-007-9001-6 .
Uggetti E, Ferrer I, Molist J, García J. Technical, economic and environmental assessment of sludge treatment wetlands. Water Res. 2011;45(2):573–82. https://doi.org/10.1016/j.watres.2010.09.019 .
Amahmid O, Asmama S, Bouhoum K. Urban wastewater treatment in stabilization ponds: occurrence and removal of pathogens. Urban Water. 2002;4(3):252–62. https://doi.org/10.1016/S1462-0758(01)00071-1 .
Farzadkia M, Ehrampoush MH, Sadeghi S, Kermani M, Ghaneian MT, Ghelmani V et al . Performance evaluation of wastewater stabilization ponds in Yazd-Iran. Environ Health Eng Manag J. 2014;1(1):7-12. http://ehemj.com/article-1-37-en.html .
Fazelipour M, Takdastan A, Sekhavatjo M. Survey on chlorine application in sequencing batch reactor waste sludge in order to sludge minimization. Asian J Chem. 2011;23(6):2994–8 http://etrc.ajums.ac.ir/_hn-dez/documents/12-90.pdf .
CAS Google Scholar
Ho LT, Van Echelpoel W, Goethals PLM. Design of waste stabilization pond systems: a review. Water Res. 2017;123:236–48. https://doi.org/10.1016/j.watres.2017.06.071 .
Decamp O, Warren A. Investigation of Escherichia coli removal in various designs of subsurface flow wetlands used for wastewater treatment. Ecol Eng. 2000;14:293–9. https://doi.org/10.1016/S0925-8574(99)00007-5 .
Evanson M, Ambrose RF. Sources and growth dynamics of fecal indicator bacteria in a coastal wetland system and potential impacts to adjacent waters. Water Res. 2006;40:475–86. https://doi.org/10.1016/j.watres.2005.11.027 .
Karimi B, Ehrampoush MH, Ebrahimi A, Mokhtari M. The study of leachate treatment by using three advanced oxidation process based wet air oxidation. Iran J Environ Health Sci Eng. 2013;10:1–7. https://doi.org/10.1186/1735-2746-10-1 .
Carty A, Scholz M, Heal K, Gouriveau F, Mustafa A. The universal design, operation and maintenance guidelines for farm constructed wetlands (FCW) in temperate climates. Bioresour Technol. 2008;99:6780–92. https://doi.org/10.1016/j.biortech.2008.01.045 .
Moore M, Cooper C, Smith S Jr, Cullum R, Knight S, Locke M, Bennett E. Mitigation of two pyrethroid insecticides in a Mississippi Delta constructed wetland. Environ Pollut. 2009;157:250–6. https://doi.org/10.1016/j.envpol.2008.07.025 .
Muga HE, Mihelcic JR. Sustainability of wastewater treatment technologies. J Environ Manag. 2008;88:437–47. https://doi.org/10.1016/j.jenvman.2007.03.008 .
Vymazal J. Removal of nutrients in various types of constructed wetlands. Sci Total Environ. 2007;380(1–3):48–65. https://doi.org/10.1016/j.scitotenv.2006.09.014 .
NewYork State Energy Research and Development Authority (NYSERDA). Evaluation of ultraviolet (UV) radiation disinfection. Enter Report. 2004.
Download references
Acknowledgements
Since this research is part of a research project approved at Gonabad University of Medical Sciences, it is hereby sponsored by Gonabad University of Medical Sciences Research and Technology, which supported the research (Project No. T/4/95) and the Code of Ethics. (IR.GMU.REC.1396.110), is appreciated.
Permission to collect data
We have a permission to collect the data from the source.
This research was funded by the Deputy of Research and Technology of Gonabad University of Medical Sciences. The funders did not have any role in the design of the study and collection, analysis, and interpretation of data and in writing the manuscript.
A grant from MOST to Tan Han Shih (Hans-Uwe Dahms) is gratefully acknowledged (MOST 107–2621-M-037-001 and MOST 108–2621-M-037-001 to T.H. Shih). A NSYSU/KMU collaboration is acknowledged (108-PO25).
Author information
Authors and affiliations.
Faculty of Public Health, Gonabad University of Medical Sciences, Gonabad, Iran
Zahra Aghalari
Department of Biomedical Science and Environmental Biology, Kaohsiung Medical University, Kaohsiung, 80708, Taiwan, Republic of China
Hans-Uwe Dahms
Research Center for Environmental Medicine, Kaohsiung Medical University, Kaohsiung, 80708, Taiwan, Republic of China
Department of Marine Biotechnology and Resources, National Sun Yat-sen University, Kaohsiung, 80424, Taiwan, Republic of China
Department of Civil and Environmental Engineering, Florida International University, Miami, FL, USA
Mika Sillanpää
Tecnologico de Monterrey, School of Engineering and Sciences, Campus Monterrey, Ave. Eugenio Garza Sada 2501, CP 64849, Monterrey, Nuevo Leon, Mexico
Juan Eduardo Sosa-Hernandez & Roberto Parra-Saldívar
You can also search for this author in PubMed Google Scholar
Contributions
ZA conceived the study, made final decisions on the inclusion of journal articles and extracted data from them, and wrote and revised the manuscript. HUD, MS, JESH and RPS wrote and revised the manuscript. All authors read and approved the final manuscript.
Corresponding author
Correspondence to Zahra Aghalari .
Ethics declarations
Ethics approval and consent to participate.
This study was approved and registered by the Code of Ethics (IR.GMU.REC.1396.110).
Consent for publication
Competing interests.
The authors declare that they have no competing interests.
Additional information
Publisher’s note.
Springer Nature remains neutral with regard to jurisdictional claims in published maps and institutional affiliations.
Rights and permissions
Open Access This article is distributed under the terms of the Creative Commons Attribution 4.0 International License ( http://creativecommons.org/licenses/by/4.0/ ), which permits unrestricted use, distribution, and reproduction in any medium, provided you give appropriate credit to the original author(s) and the source, provide a link to the Creative Commons license, and indicate if changes were made. The Creative Commons Public Domain Dedication waiver ( http://creativecommons.org/publicdomain/zero/1.0/ ) applies to the data made available in this article, unless otherwise stated.
Reprints and permissions
About this article
Cite this article.
Aghalari, Z., Dahms, HU., Sillanpää, M. et al. Effectiveness of wastewater treatment systems in removing microbial agents: a systematic review. Global Health 16 , 13 (2020). https://doi.org/10.1186/s12992-020-0546-y
Download citation
Received : 22 October 2019
Accepted : 28 January 2020
Published : 03 February 2020
DOI : https://doi.org/10.1186/s12992-020-0546-y
Share this article
Anyone you share the following link with will be able to read this content:
Sorry, a shareable link is not currently available for this article.
Provided by the Springer Nature SharedIt content-sharing initiative
- Systematic analysis
- Microbial agents
- Environmental health
Globalization and Health
ISSN: 1744-8603
- Submission enquiries: [email protected]
- General enquiries: [email protected]
Thank you for visiting nature.com. You are using a browser version with limited support for CSS. To obtain the best experience, we recommend you use a more up to date browser (or turn off compatibility mode in Internet Explorer). In the meantime, to ensure continued support, we are displaying the site without styles and JavaScript.
- View all journals
- My Account Login
- Explore content
- About the journal
- Publish with us
- Sign up for alerts
- Review Article
- Open access
- Published: 08 July 2021
Removal of heavy metal ions from wastewater: a comprehensive and critical review
- Naef A. A. Qasem ORCID: orcid.org/0000-0002-3641-3996 1 , 2 ,
- Ramy H. Mohammed ORCID: orcid.org/0000-0002-5103-1726 3 &
- Dahiru U. Lawal ORCID: orcid.org/0000-0002-2185-6575 2
npj Clean Water volume 4 , Article number: 36 ( 2021 ) Cite this article
160k Accesses
517 Citations
6 Altmetric
Metrics details
- Chemical engineering
- Pollution remediation
An Author Correction to this article was published on 07 December 2021
This article has been updated
Removal of heavy metal ions from wastewater is of prime importance for a clean environment and human health. Different reported methods were devoted to heavy metal ions removal from various wastewater sources. These methods could be classified into adsorption-, membrane-, chemical-, electric-, and photocatalytic-based treatments. This paper comprehensively and critically reviews and discusses these methods in terms of used agents/adsorbents, removal efficiency, operating conditions, and the pros and cons of each method. Besides, the key findings of the previous studies reported in the literature are summarized. Generally, it is noticed that most of the recent studies have focused on adsorption techniques. The major obstacles of the adsorption methods are the ability to remove different ion types concurrently, high retention time, and cycling stability of adsorbents. Even though the chemical and membrane methods are practical, the large-volume sludge formation and post-treatment requirements are vital issues that need to be solved for chemical techniques. Fouling and scaling inhibition could lead to further improvement in membrane separation. However, pre-treatment and periodic cleaning of membranes incur additional costs. Electrical-based methods were also reported to be efficient; however, industrial-scale separation is needed in addition to tackling the issue of large-volume sludge formation. Electric- and photocatalytic-based methods are still less mature. More attention should be drawn to using real wastewaters rather than synthetic ones when investigating heavy metals removal. Future research studies should focus on eco-friendly, cost-effective, and sustainable materials and methods.
Similar content being viewed by others
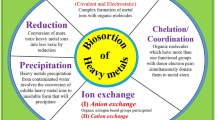
Toxic heavy metal ions contamination in water and their sustainable reduction by eco-friendly methods: isotherms, thermodynamics and kinetics study
Veer Singh, Ghufran Ahmed, … Ashish Kumar
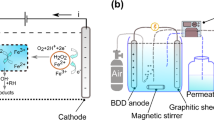
Recent developments in hazardous pollutants removal from wastewater and water reuse within a circular economy
Menatalla Ahmed, Musthafa O. Mavukkandy, … Shadi W. Hasan
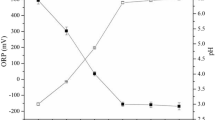
Removal behavior and chemical speciation distributions of heavy metals in sewage sludge during bioleaching and combined bioleaching/Fenton-like processes
Chunsheng Qiu, Shangyu Xie, … Shaopo Wang
Introduction
The presence of heavy metals in wastewater has been increasing with the growth of industry and human activities, e.g., plating and electroplating industry, batteries, pesticides, mining industry, rayon industry, metal rinse processes, tanning industry, fluidized bed bioreactors, textile industry, metal smelting, petrochemicals, paper manufacturing, and electrolysis applications. The heavy metal contaminated wastewater finds its way into the environment, threatening human health and the ecosystem. The heavy metals are non-biodegradable 1 and could be carcinogenic 2 , 3 , 4 , 5 , 6 ; thus, the presence of these metals in water by improper amounts could result in critical health issues to living organisms.
The most popular heavy metals are lead (Pb), zinc (Zn), mercury (Hg), nickel (Ni), cadmium (Cd), copper (Cu), chromium (Cr), and arsenic (As). Although these heavy metals can be detected in traces; however, they are still hazardous. Table 1 summarizes some heavy metals, focusing on their major sources, health effects, and the permitted quantity in drinking water. The aforementioned metals and others such as silver (Ag), iron (Fe), manganese (Mn), molybdenum (Mo), boron (B), calcium (Ca), antimony (Sb), cobalt (Co), etc. are commonly available in wastewater and need to be removed.
Recent studies have focused on a particular method for heavy metal ions removal, such as electrocoagulation (EC), adsorption using synthetic and natural adsorbents, magnetic field implementation, advanced oxidation processes, membranes, etc. These studies stood on the advantages and disadvantages of a specific method for wastewater treatment, including heavy metal removal. A complete picture of the heavy metals removal methods from wastewater resources has not been drawn yet. Therefore, the present review comprehensively and critically discusses the available technologies to expel heavy metal ions from wastewater efficiently. Moreover, it is essential to choose the most applicable method based on the removal efficiency, chemicals added/adsorbents, initial concentration, optimal treated pH value, and other operating conditions.
The methods discussed in this review are classified into adsorption-, membrane-, chemical-, electric-, and photocatalytic-based treatments. An assessment for each method is conducted. Additional details about the operating conditions, removal efficiency, and important remarks of each method are listed for the reported studies in the literature in the accompanying Supplementary Information file. The literature research is selected based on the availability of the operating and performance parameters for each method.
Adsorption-based separation
The adsorption mechanism is defined by the physicochemical properties of adsorbent and heavy metals and operating conditions (i.e., temperature, adsorbent amount, pH value, adsorption time, and initial concentration of metal ions). Generally, heavy metal ions can be adsorbed onto the adsorbent’s surface, as shown in Fig. 1a . This method was reported to have low operating costs, high removal capacity, easy implementation, and simple treatment by regenerating the adsorbed heavy metal ions 7 . Different types were developed for wastewater remediation, as discussed in the following sections.
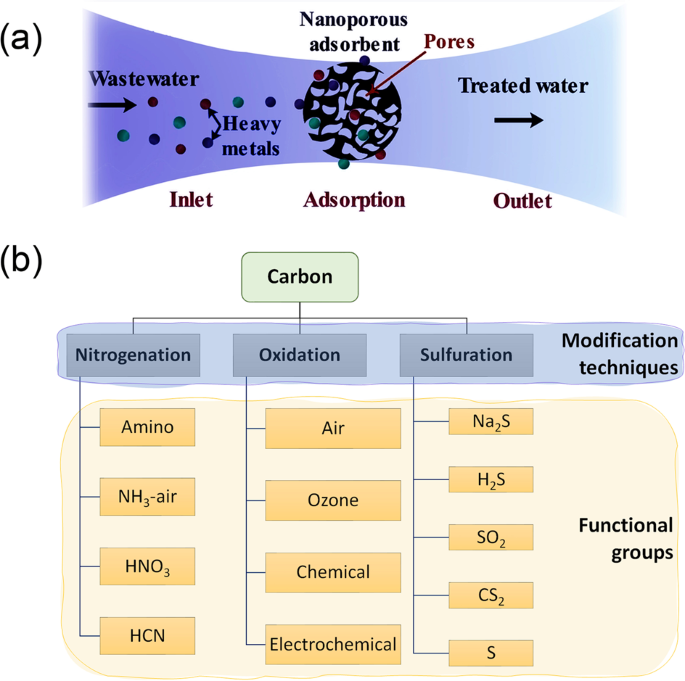
a Heavy metal ions adsorption process; the metal ions of wastewater adhere to the surface of nanoporous adsorbents, which has a high surface area due to its porosity. The adsorption process could be selective for one or more metals than others. The regeneration process could be achieved using a desorbing agent. b Various modification techniques (i.e., nitrogenation, oxidation, and sulfuration) are used to functionalize carbon with different functional groups. Functionalization enhances adsorption capacity and stability.
Carbon-based adsorbents
Carbon-based nanoporous adsorbents, especially activated carbons (ACs), carbon nanotubes (CNTs), and graphene (GN), are extensively used in the applications of heavy metal removal owing to their tremendous surface area (500–1500 m 2 /g) 8 . The carbon surface charges can be enhanced by surface functional groups (such as carboxyl, phenyl, and lactone groups, as shown in Fig. 1b ) to improve the heavy metal uptake 9 . Among various modification methods, nitrogenation, oxidation, and sulfuration are the most commonly employed techniques to enhance the specific surface area, pore structure, adsorption capacity, thermal stability, and mechanical strength 10 . However, they depend mainly on the adsorbent materials, which sometimes are very expensive 11 . Subsequently, adsorbent’s cost should be considered in choosing the most suitable adsorbents.
Surface modification often reduces its surface area and, in turn, increases the content of surface functional groups. Consequently, more metal ions can be adsorbed 12 . Supplementary Tables 1 and 2 summarize the removal capacity and characteristics of carbon-based adsorbents and composite adsorbents. The adsorption uptake increases by increasing the adsorbent surface area, adsorbent dose, initial concentration of metal ions, and contact time. Although the multi-wall carbon nanotubes (MWCNTs) have received particular interest for heavy metal removal 13 , they are highly hydrophobic and suffer from rapid aggregation in aqueous solution due to large Van der Waals forces, decreasing the adsorption potential.
There is a lack of literature in quantitative assessment of functional groups’ role in heavy metal ions sorption. Moreover, the current surface modification techniques demand high heat/pressure, strong acid/base, or intensive oxidation/reduction reactions. This complex preparation process makes the carbon-based adsorbents expensive, burdening their widespread use in industrial applications. Thus, researchers should propose innovative, low-cost, and environmentally friendly surface modification techniques.
Chitosan-based adsorbents
Chitosan (CS) is a natural adsorptive polymer that has an affinity toward pollutants in wastewaters because it has amino (–NH 2 ) and hydroxyl (–OH) groups 14 . Despite its unique features, it suffers from low mechanical strength and poor stability 15 , making the regeneration inefficient. Also, it is challenging to use CS in its powder or flake form because of its low porosity, low surface area, resistance to mass transfer, and high crystallinity 15 . Consequently, structural and chemical modifications have been proposed to overcome these drawbacks. Cross-linking chemical modification imparts strength to CS by bridging between polymer chains and the functional groups. However, this approach reduces the uptake 16 .
Grafting is another chemical modification method that involves the covalent bonding of functional groups (like amine and hydroxyl) on the backbone of CS, leading to a remarkable increase in the adsorption capacity 17 . Combining CS with other adsorbent materials has also been proposed to enhance CS’s adsorption capacity, mechanical strength, and thermal stability 18 . The ion-imprinting technique was followed to prepare adsorbents which high selectivity for target metal ions 19 .
Supplementary Table 3 lists the uptake of different CSs for heavy metal ions removal from wastewater. Generally, the uptake of CS depends mainly on the presence of protonation or non-protonation of amine (–NH 2 ) and phosphoric (H 3 PO 4 ) groups, which affect the pH value of the wastewater. In the absence of the modifications, CS-based shows low reusability. This behavior might be attributed to the strong bond (between the metal ions and adsorbent surface), low thermal/chemical stability, low mechanical strength, incomplete desorption, declination in the effective adsorbate-adsorbent interaction, and unavailability of adsorption sites 20 . So, alternative regeneration methods and modifications should be proposed to enhance the reusability of CSs.
Mineral adsorbents
Mineral adsorbents such as zeolite, silica, and clay are considered good candidates for water purification with low operating costs 21 . Clay has extraordinary cation exchange capacity (CEC), cation exchange selectivity, surface hydrophilicity, high swelling/expanding capacity, and surface electronegativity 22 . In addition, acid washing, thermal treatment, and pillar bearing could enlarge the pore size, pore volume, and specific surface area, leading to a remarkable increase in the adsorption efficiency 22 . Research studies (listed in Supplementary Table 4 ) showed that physical adsorption, chemical adsorption, and ion exchange are the most common mechanisms controlling wastewater treatment using mineral adsorbents. Besides the mentioned parameters, the pH, temperature, adsorption time, and adsorbent dosage are also considered vital parameters controlling the adsorption process. The adsorption removal efficiency increases when the pH increases and the initial concentration decreases 23 .
Using natural minerals could be cost-effective. However, the removal efficiency might decrease after a few cycles 24 . Therefore, different modification methods, such as calcination and impregnation, have been proposed to enhance the removal efficiency of such adsorbents 25 . However, these modifications incur additional costs to the process and release new chemical agents into the environment. Grafting functional groups could synthesize eco-friendly and multifunctional adsorbents suitable for treating various types of wastewaters. The preparation of two-dimensional nanosheets and one-dimensional nanotubes-based clay adsorbents might lead to innovative low-cost and high-performance adsorbents.
Magnetic adsorbents
Magnetic adsorbents are a specific material matrix that hosts iron particles (usually magnetic nanoparticles, such as Fe 3 O 4 ) 26 . The base material could be carbon, CS, polymers, starch, or biomass. As illustrated in Fig. 2 , the adsorption process is affected by the magnetic field, surface charge, and redox activity characteristics. They showed low-cost, easy-synthesis, extraordinary surface charge, and reusability. Many magnetic adsorbents were proposed in the literature, such as zero-valent iron nanoparticles (ZVI NPs), iron oxides (hematite (α-Fe 2 O 3 ), maghemite (γ-Fe 2 O 3 ), magnetite (Fe 3 O 4 )), and spinel ferrites. The mechanism and kinetics of the sorption process rely on several parameters, such as surface morphology and adsorbent magnetic behavior. They are also affected by experimental conditions such as pH, irradiation time, adsorbent concentration, wastewater temperature, and the initial dosage of pollutants 27 . The presence of iron particles in adsorbent is very efficient in metal ions removal from effluent 28 .
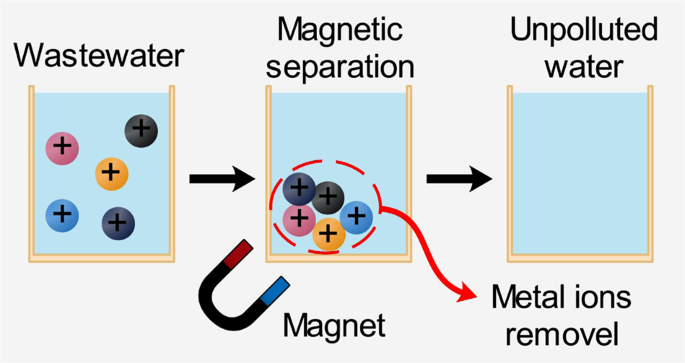
The magnetic adsorbent particles adsorb the metal ions and sequentially accumulated; thus, the wastewater is treated.
Some studies have focused on coating Fe 3 O 4 particles for removing heavy metal ions. Co-precipitation, high-gravity technology, and grafting are the most commonly used methods 29 . The grafting method was considered a preferable choice because it is flexible and straightforward. However, it strongly depends on the active hydroxyl on the surface of Fe 3 O 4 particles and the number of active functional groups. The produced adsorbents were not adequately cyclic stable, which is a barrier facing the commercialization of this method. Additional details about different magnetic adsorbents can be found in Supplementary Table 5 .
Biosorbents
The most recent research studies in using biosorption for wastewater treatment are listed in Supplementary Table 6 . The presence of numerous functional groups (i.e., carboxyl, amino, hydroxyl, phosphate, thiol, etc.) on the surface expedite the biosorption process 30 . Generally, the interaction between pollutants and the surface of biosorbent can occur through electrostatic interaction, aggregation, complexation/coordination, microprecipitation, ion exchange, reduction, or oxidation 31 . The solution pH affects the biosorbent surface charge density and ionization of functional groups located on the biosorbent surface 32 . When pH is low, cations are almost stable and can be bonded to the biosorbent surface. On the other hand, at higher pH values, the solubility of metal cations decreases with the possibility of a precipitation phenomenon.
The biosorbent amount is a vital factor affecting the removal efficiency due to offering more vacant biosorption sites. The biosorbent capacity could increase at higher temperatures due to decreased solution viscosity, reduction in Gibb’s free energy, and bond rupturing. These reasons increase the collision frequency (mobility and kinetic energy) between biosorbent and metal ions and enhance the biosorbent active sites, leading to a higher affinity 31 . In turn, the bonding force between biosorbent and pollutants could decline at higher temperatures, and thus the biosorbent sorption uptake reduces. It was elucidated that the removal efficiency increases as the mixing agitation rate increases 33 .
Metal-organic frameworks adsorbents
Metal-organic frameworks (MOFs) are generally synthesized via reticular synthesis in which metal ions are strongly bonded to organic linkers. Researchers proposed thousands of MOFs. It was noticed that most of the organic ligands used to form many MOFs are very expensive and toxic 34 . Zirconium-MOFs family (such as UiO-66) is promising nanostructure materials for sorption applications due to the easy incorporation of functional groups and hydrolytic-thermal stability such as amine, carboxylic, hydroxyl, and oxygen 35 or by using the cross-linking method 36 . Composite-based MOF adsorbents could obtain further enhancement in the adsorption capacity of MOFs. Supplementary Table 7 lists the uptake of different MOFs towards several heavy metal ions in wastewater.
Despite the exciting features of MOFs and their good capability to remove heavy metal ions, they have micropores (i.e., tiny pores) inaccessible for some target metals. Also, most of them have low stability in water. Mn, Fe, and Cu have been used to form MOFs, but most of them resulted in poor chemical stability 37 , 38 , 39 , 40 , 41 , 42 , 43 , 44 , 45 , 46 , 47 , 48 , 49 , 50 . Therefore, further research is still needed to tune the MOFs’ structure and scale up these materials to implement them into industrial wastewater applications. Moreover, different functionalization methods should be proposed and applied to enhance MOFs’ stability and sorption kinetics.
The reported maximum uptakes of heavy metal ions for a proper adsorbent are listed in Table 2 .
Membrane-based filtration and separation
Over the years, technological advancement in membrane development has led to an increase in the use of membranes for filtration and extraction of heavy metal ions from wastewater. A simplified schematics for different membrane-based filtration processes is illustrated in Fig. 3a–c , while Fig. 3d demonstrates various pollutants that can be separated by different membrane techniques 51 .
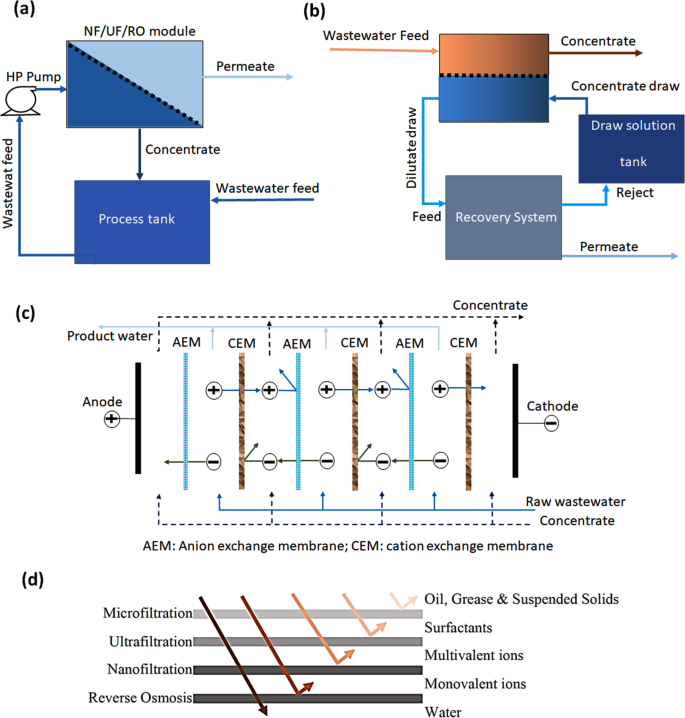
a nanofiltration, ultrafiltration, or reverse osmosis method, b forward osmosis process, c electrodialysis method in which alternative charged positive and negative membranes take place, and d the separation capabilities of different membranes against different pollutants.
Ultrafiltration
Ultrafiltration (UF) is used at low transmembrane operating pressure (TMP). Because UF membrane pores may be larger than the heavy metal ions, additives may be bonded to metal ions to enlarge the size of the metal ions. Therefore, micellar enhanced ultrafiltration (MEUF) and polymer enhanced ultrafiltration (PEUF) are proposed.
MEUF is formed by bonding UF and surfactant. MEUF has high flux and high selectivity, leading to low-energy consumption, high removal efficiency, and less space demand 52 . MEUF is most suitable for wastewater whose heavy metals are in low concentrations 53 . In MEUF, a surfactant is mixed with wastewater in a concentration above the critical micellar concentration (CMC). Beyond CMC, surfactant monomers assemble and increase the creation of some micelles in the solution. The surfactant contains a hydrophobic tail and a hydrophilic head. The inner hydrophobic core of the micelles could solubilize organic matters (having low molecular weight) as a solubilizate, while the surface adsorbs counter metal ions on their surface due to electrostatic interactions 54 . Surfactants, whose electric charge is the opposite of the metal ions, usually attain the highest retentions 55 , 56 , 57 . In this regard, polyelectrolytes (PE), cationic surfactants, and anionic surfactants (e.g., sodium dodecyl sulfate (SDS)) are used for effective heavy metals extraction 55 .
A summary of different studies on the MEUF process is given in Supplementary Table 8 . The performance of MEUF depends on several factors, including the added solutes, type of surfactant, operating conditions, and kind of membrane used.
PEUF is formed through the integration of UF and binding polymers. The functional groups of the bonding polymers could be sulfonate, phosphonic, carboxylated, or amine, and they are bonded via chelating or ionic bonds 58 . PEUF are also known as polymer-supported, complexation, polymer-assisted, size enhanced, and complexation enhanced ultra-filtrations. While permitting water and un-complexed components to permeate the membrane pores, the PEUF process blocks and extracts polymer-bonded metal ions 59 .
A summary of studies conducted on PEUF is presented in Supplementary Table 9 . PEUF shows effective polymer bonding, effective extraction, ability to recover and reuse complexation polymer of retentate, low-energy demands, and low-cost operation 51 , 55 . However, the choice of appropriate water-soluble polymer macro-ligands remains the main challenge of developing this technology.
Nanofiltration
Nanofiltration (NF) is used to concentrate constituents whose molecular weight is >1000 Da and remove solutes whose size of 0.0005–0.007 μm with molecular weights >200 Da 60 . Thus, the operating range of NF is between UF and reverse osmosis (RO) processes 55 . The NF membranes are composed of polymer composites of multiple-layer thin-film of negatively charged chemical groups. Anti-fouling NF membranes containing CeO 2 /Ce 7 O 12 and PES were synthesized through phase inversion and used to extract Fe 3+ , Al 3+ , Co 2+ , Cd 2+ , Cu 2+ , and humic acid from wastewater and reached extraction efficiency between 94 and 98% 61 . Other studies are presented in Supplementary Table 10 .
Microfiltration
Microfiltration (MF) employs a microporous membrane to remove micron-sized particles, bacteria, viruses, protozoa, contaminants, pollutants, etc., from a solvent/fluid/solution 60 . MF process is also a low pressure-driven membrane process, whose membrane pores are in the range of 0.1–10 μm 60 . Some of the MF membranes are made of silica, ceramics, zirconia, alumina, PVC, polysulfone, PTFE, polypropylene, PVDF, polyamides, polycarbonate, cellulose acetate, cellulose esters, or composite materials. The commercial application of MF is widely found in pharmaceutical and biological industries. However, the application of the MF system may be found in particle removal of the rinse water in the semiconductor industry, sterilization of beer and wine, other juices and cider clarification, and wastewater treatment 60 . The application of MF in heavy metal removal has not drawn enough attention because of its low removal ability. However, it has been used by modifying membrane or chemical pre-treatment of the feed solution. Depending on the mode of application, the MF process is available in two main configurations: crossflow and dead-end. Some studies on MF are summarized in Supplementary Table 11 .
Reverse osmosis
RO is a pressure-driven separation process that employs a semi-permeable membrane (pore size 0.5–1.5 nm) to allow only smaller molecules to pass. RO process reverses the normal osmosis process by applying pressure (20–70 bar) >the osmotic pressure of the feed solution. The molecular size of the solutes blocked is usually in the range of 0.00025–0.003 μm 60 . RO process could extract 95–99% of inorganic salts and charged organics 60 . RO process is compact and attained high rejection efficiency. However, membrane fouling and degrading are the major drawback of RO systems 60 . The RO separation process was used to extract heavy metal ions, including Ni 2+ , Cr 6+ , and Cu 2+ from electroplating wastewater, with a removal efficiency of >98.75 62 . Recently, RO has been used to purify industrial wastewaters from coster-field mining operations located in Victoria-Australia with mean extraction efficiency of 10%, 48%, 82%, 66%, and 95% for Fe 3+ , Zn 2+ , Ni 2+ , As 3+ , and Sb 3+ respectively 63 . Other studies on RO heavy metals removal are summarized in Supplementary Table 12 .
Forward osmosis
Forward osmosis (FO) is an osmosis process that requires a membrane to balance selectivity and permeated water flux 51 . In FO, a semi-permeable membrane separates a feed solution from the draw solution, as shown in Fig. 3b . The draw solution is usually at a higher osmotic pressure compared to the feed solution. Due to the osmotic pressure difference between the feed and draw solutions, water transports from the feed solution to the draw solution, thereby keeping the rejected solutes on the feed side and treated water on the draw solution 64 . FO does not require hydraulic pressure; thus, it is energy-saving. FO process is also environmentally friendly, easy cleaning, and low fouling; therefore, it is widely used in wastewater treatment 64 . Nevertheless, FO has limitations, such as draw solution re-concentration, membrane selection challenges, internal and external concentration polarization 65 . Supplementary Table 13 summarizes the use of FO, including thin-film membranes.
Electrodialysis
Electrodialysis (ED) is used to separates ions at the expense of electric potential difference. ED uses a series of cation exchange membranes (CEM) and anion exchange membranes (AEM), alternatively arranged in parallel, to separate ionic solutes 51 . In the ED process, the anions pass through AEM, while cations pass through CEM. In such a case, the treated stream (diluate) is produced from half of the ED stack channels, while the concentrated stream is expelled from the other half, as shown in Fig. 3c . ED offers high water recovery, no phase change, no reaction, or chemical involvement 66 , and can operate over a wide range of pH values. However, ED also exhibits membrane fouling, high cost of membranes, and demand for electric potential.
ED has been used to separate Ni 2+ , Pb 2+, and K + from synthetic solution through a novel ED heterogeneous CEM (consisting of 2-acrylamido-2-methyl propane sulfonic acid-based hydrogel and PVC) to attain extraction efficiency of 96.9%, 99.9%, and 99.9% for Ni 2+ , Pb 2+, and K + , respectively 67 . A batch ED process was employed to recover Pb 2+ and reached a maximum separation efficiency of ~100% 68 . A pilot-scale ED system has also been used to extract Cu 2+ , Ni 2+ , and traces of Cd 2+ , Fe 3+ , Cr 6+, and Zn 2+ , and exceeded 90% removal rate 69 . As 3+ and As 5+ were removed from metallurgical effluent by ED and attained a removal efficiency of 91.38% 70 .
Other membrane-based methods
Membrane distillation (MD) and liquid membrane (LM) are also used for wastewater treatment. MD is a hybrid thermally driven membrane separation process that consists of cold and hot compartments separated by a microporous hydrophobic membrane. MD allows only vapor to permeate its pores while blocking other molecules. MD exists in four configurations: direct contact MD, air gap MD, sweeping gas MD, and vacuum MD. MD process has been reported to achieve over 96% removal of Ca 2+ , Mg 2+ , Fe 3+ , and Fe 2+ 71 , and more than 99% for As 3+ and As 5+ 72 .
On the other side, LM is made of a liquid phase or thin-layer organic phase, which acts as a barrier between two aqueous phases. LM is immiscible to the feed solution and retentate solution 51 , and combined stripping and extraction processes in a single stage 73 . LM is highly selective, relatively efficient, and can achieve specific molecular recognition. However, the membrane’s long-term stability is poor 74 . LM process exists as a supported liquid membrane (SLM), emulsion liquid membrane (ELM), bulk liquid membrane (BLM), and polymer inclusion membrane (PIM) 51 . Among these types, the SLM process is an attractive alternative to traditional solvent extraction for heavy metal removal 73 . SLM achieved a removal efficiency of 89% for Zn 2+ , Cd 2+ , Cu 2+ , and Fe 3+ 75 .
Chemical-based separation
Chemical methods for removing heavy metals from wastewater are mature and used early. In this section, the chemical-based methods will be discussed, including precipitation, coagulation-flocculation, and flotation.
Precipitation
Chemical precipitation (the so-called coagulation precipitation) is broadly used in industries and is considered one of the most effective and mature methods. It changes the form of dissolved metal ions into solid particles to facilitate their sedimentation. The reagent coagulation (coagulant) precipitates metal ions by changing pH, electro-oxidizing potential, or co-precipitation 76 . It is usually followed by the removal of sediments. A simple schematic of the chemical precipitation process is depicted in Fig. 4 .
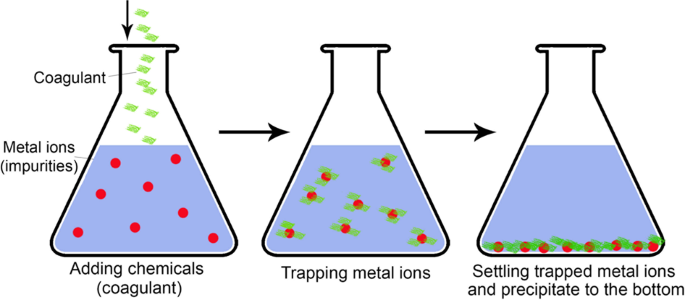
The coagulant is added to wastewater and stirred to trapping metal ions that settle and precipitate to the bottom of the container.
Hydroxide precipitation is broadly used due to its relatively inexpensive, simple, and tunable pH 77 . It is implemented by adding a hydroxide to the stirred wastewater to form insoluble metal hydroxide precipitates. For example, a metal ion could react with calcium hydroxide (lime) to produce metal hydroxide precipitates and calcium ions as:
It was found that pH values of 9–11 improved this process efficiency 78 . However, a high pH value is considered a disadvantage of this method since it requires a large dosage of precipitates. One of the most effective hydroxide precipitates for treating inorganic effluents of heavy metal concentration of 1000 mg/L is lime (CaO or Ca(OH) 2 ) 79 . A summary of some hydroxide precipitation studies is presented in Supplementary Table 14 . It can be seen that the majority of metals removed by this method are Zn 2+ , Cu 2+ , Ni 2+ , Pb 2+ , and Cr 3+ . In addition to the need for the high dosage to get optimal pH, there are some drawbacks, such as relatively large volumes of sludge leading to dewatering, disposal issues, amphoteric, and the inhabitation of metal hydroxide precipitation with the presence of complexing agents.
The sulfide participation method distinguishes itself by higher removal efficiency and lesser dissolved solids increment than the hydroxide method. This method was reported to treat toxic heavy metal ions 80 . Lower sulfide results in a higher zinc concentration in the effluent, while higher sulfide leads to a malodor problem due to high residual sulfide. Also, it could produce hydrogen sulfide gas which is malodorous and toxic. For these reasons, the sulfide precipitation is recommended to be executed at a neutral pH 81 . The metal sulfide precipitations could follow Eq. ( 2 ) reaction,
Supplementary Table 15 summarizes the metal ions removal using sulfite precipitations. The toxicity of sulfide and its high cost are the most shortcomings.
As an alternative method to hydroxide precipitation, carbonate precipitation shows good effectiveness and optimum precipitation at lower pH values 82 . It could be achieved using sodium carbonate or calcium carbonate. The classical carbonates can be formed based on Eq. ( 3 and 4 ) 83 :
It could have less sludge volume, but it could release CO 2 bubbles and needs higher reagents for efficient precipitation 83 . Supplementary Table 16 lists some studies concerning carbonate precipitation. It can be seen that copper and manganese are the major metals removed by this technology. Zinc and lead could also be removed efficiently.
Fenton reaction is usually used to improve the removal efficiency of the chemical precipitation methods. The Fenton or Fenton-like oxidation is used for the de-complexation of heavy metal complexes. However, the pH is adjusted by the chemical precipitation mechanism (e.g., NaOH). Fenton chemistry is not straightforward, and it is performed through numerous reactions, depending upon various active intermediates, such as [Fe IV O] 2+ and hydroxyl radicals 84 , 85 . The classical Fenton reaction is 86 :
Fe 3+ -H 2 O 2 (Fenton-like 87 ) and Fe 0 -H 2 O 2 (advanced Fenton 88 ) are also represented as an alternative for Fe 2+ -H 2 O 2 . Additional detailed examples of using the Fenton-integrated chemical precipitation method are summarized in Supplementary Table 17 .
Some metals are represented in small volumes in bulk wastewater, such as radionuclides. The removal of radioactive metals is listed in Supplementary Table 18 .
Coagulation and flocculation
Coagulation is the destabilization of colloids by neutralizing the forces that keep them parted, while flocculation is the agglomeration of destabilized particles 89 . Traditional coagulants are aluminum, ferrous sulfate, and ferric chloride, using to neutralization of ion charges. Flocculation bonds the particles forming large agglomerates with the help of a flocculant, such as polyaluminum chloride (PAC), polyferric sulfate (PFS), polyacrylamide (PAM), and other macromolecule flocculants 90 . The PE were reported as one of the most practical flocculations, but the produced sludge might be toxic 89 . The flocculants are generally not natural and non-biodegradable 91 .
The process is illustrated in Fig. 5 , including the sedimentation. Some weaknesses are toxicity and health hazardousness of inorganic coagulants, a large volume of sludge, selective for some metals and inefficient in emerging contaminants, increasingly effluent color, inefficient when using natural coagulants, and complex of scaling up 92 . Supplementary Table 19 summarizes some of the studies conducted on coagulation-flocculation for heavy metal removal from different wastewater sources. The typical heavy metals removed by this method include Cu 2+ , Pb 2+ , and Ni 2+ . Other metals such as As 2+ , Se 2+ , Cr 2+ , Sb 3+ , Sb 5+ , Ag 2+ could also be efficiently removed.
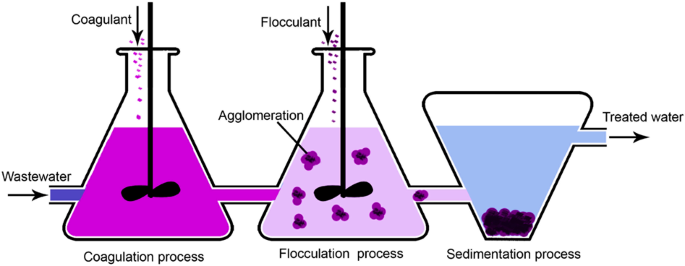
Coagulation and flocculation could be either two sequential processes or one process. The sedimentation process could be replaced with filtration or another method.
Flotation is used to remove various metal ions. The general schematic of the flotation process is shown in Fig. 6 . Dissolved air flotation, ion flotation, and precipitation flotation were extensively studied. In the dissolved air flotation, air (or gas) is fed to wastewater to generate micro-bubbles that could attach the metal ions, developing lower density agglomerates, leading to raising the flocs through the wastewater. The accumulated slug at the top surface can easily be removed 93 .
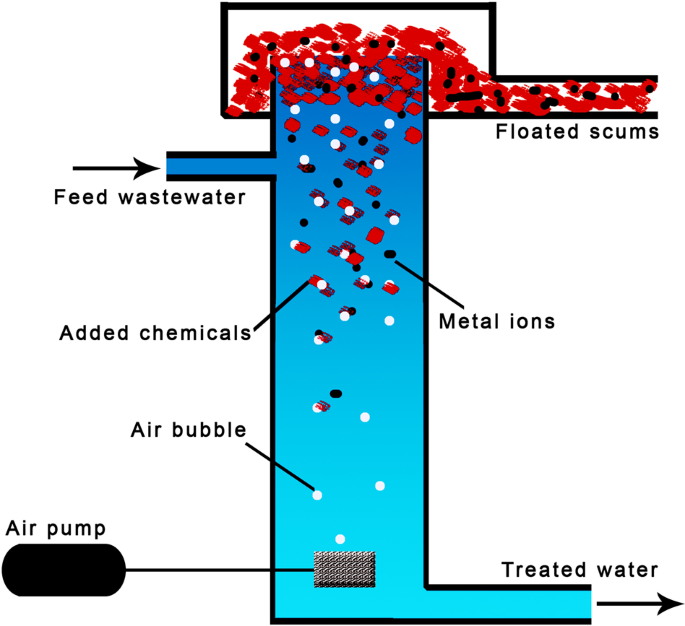
Chemicals such as collectors are added to be attached with metal ions and microbubbles, leading to lower density agglomerates floated and removed from the top of the treatment column.
The ion flotation process relies on the increase of hydrophobicity of metal species by using surfactants; therefore, the hydrophobic species are removed by air bubbles. The added surfactant facilities as collectors, while frothers control the indexes of ion flotation 94 . When the concentration of metal ions is low within a large quantity of wastewater, the ion flotation appears inefficient 95 . The ion flotation process showed low-energy consumption, limited volume demands, reduced sludge volumes, and selective treatment 96 . The precipitation flotation process is basically a chemical precipitate process that implements micro-bubbles. That precipitation flotation takes a short time to complete efficiently 97 . Generally, the flotation processes have advantages such as rapid operation, compact process, and a moderate cost.
Significant attention has recently been paid to ion flotation among all flotation processes, as observed from Supplementary Table 20 . Since ion flotation depends on surfactants as collectors, efficient and non-toxic surfactants are required. Chemical synthetic surfactants have been introduced to have strong collection ability, good selectivity, and easy construction. However, the cost and toxicity issues limit them. On the other side, bio-surfactants sound more environmentally friendly, but they exhibited low removal rates, large dosage quantity, and longtime process 94 . In this regard, nanoparticles have been proposed as new collectors that showed both benefits of synthetic- and bio-surfactants 94 .
Electric-based separation
In this section, different electrochemical methods (i.e., electrochemical reduction (ER), EC, electroflotation (EF), and electrooxidation (EO)), and ion exchange method are discussed.
Electrochemical treatment
In an electrochemical system, oxidation is performed at the anode (positive side), where electrons transfer to the cathode (negative side), at which the reduction process occurs. These two chemical reactions are called redox (reduction-oxidation), leading to water purification through metal removal. For example:
Selection of the anode and cathode mainly decides the type of the electrochemical method and influences the removal efficiency towards specific metal ions. Figure 7a shows different types of electrode arrangements, namely: monopolar electrodes in series (MP-S) arrangement, monopolar electrodes in parallel (MP-P) arrangement, and bipolar electrodes in series (BP-S) arrangement 98 . MP-S and MP-P are preferable from the economic point of view and also yield high products.
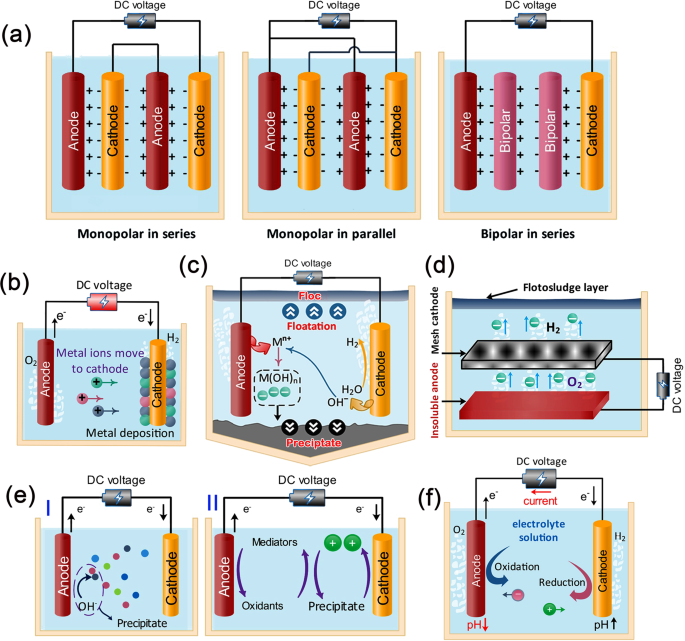
a Different arrangements of electrodes in electrolysis cell (i.e., monopolar electrodes in series (MP-S), monopolar electrodes in parallel (MP-P), and bipolar electrodes in series (BP-S)), b mechanism of the electrochemical reduction method in which positive metal ions are deposited over the cathode, c electrocoagulation process showing the flotation and precipitation of metal ions, d basic electroflotation methods—anions are released from anode to combine with cations (metal ions) and then floating over the water, e electrochemical oxidation (EO) process ((I) direct EO in which the ions react with OH and precipitate or (II) indirect EO in which mediators are used to forming oxidants that interact with the ions to be removed), and d electro-Fenton process in which OH − is released at anode and reacts with metal ions, and hence wastewater is purified due to precipitation.
The electrochemical processes are primarily classified into ER, EC, EF, and EO processes. In the ER method, also known as electrodeposition and electroplating, targeted atoms or molecules are deposited on the surface of the cathode, as shown in Fig. 7b . Sludge, which requires further treatment, is not formed in this treatment. It is worth noting that cathodes with high over-potential toward hydrogen evolution are more efficient in pollutant removal/reduction 99 . Supplementary Table 21 lists the most recent research studies of heavy metals removal using ER and its conditions. The cathodes made of carbon-based or sulfur mixture with different ratios in acidic conditions are suitable for removing Hg 2+ , Cd 2+ , Pb 2+ , and Cu 2+ from wastewater 100 . Iridium oxide-coated titanium anode was found as a perfect material for Cd 2+ removal with an efficiency of 100% regardless of its initial concentration 101 . More attention should be paid to the reactor design and the operating conditions to enhance the performance of the wastewater treatment 101 . Energy consumption is a barrier that should be solved to commercialize this type of treatment in industrial applications.
In the EC method, steel (iron) or aluminum electrodes, which are non-toxic and reliable, are mostly used 102 . The mechanism of the EC method is sequentially as; dissolving cations of anodic metal (Eq. ( 8 )), hydroxo complex formation (coagulants, Eq. ( 9 )), aggregate stability and phase separation, and precipitation and flotation.
Cations from anode destabilize colloidal particles and also form polymeric hydroxide complex metal ions (i.e., coagulants), which react with pollutants (negatively charged) present in wastewater as:
Therefore, metal hydroxide (larger particles) whose density is higher than water forms and precipitates (settle down), while floc (fine particles) floats, as illustrated in Fig. 7c . The coagulants are generated in situ due to anode oxidation, which is considered a further advantage of EC methods over other technologies. Supplementary Table 22 summarizes the most recent EC studies for heavy metal removal from wastewater. Generally, it was found that lower energy consumption and higher removal efficiency are achieved when the alternating current is used rather than the direct current. Also, the rise in temperature, voltage, and pH reduces the test duration.
EC drawbacks are the electrodes passivation and relatively high energy consumption 103 and the challenge of large-scale applications at lower energy consumption 104 . Many successful approaches were proposed to alleviate the passivation of the electrode, such as aggressive ion addition, alternating current operation, polarity reversal operation, ultrasonication, mechanical cleaning of electrodes, chemical cleaning of electrodes, hydrodynamic scouring. However, each route has drawbacks, such as generating hazardous byproducts, costly, additional treatment, and infrastructure, and increasing sludge production 105 . Accordingly, the EC process is still not fully mature applications.
The mechanism of EF is mainly based on carrying out water electrolysis on insoluble electrodes, while the flotation effect is introduced to facilitate the treatment process 106 , as sketched in Fig. 7d . So, the process efficiency depends on tiny (~ 0.15 mm) and uniform bubbles. Details of EF separation are listed in Supplementary Table 23 . It can be concluded that Ti-based anodes were widely used in the EF process 107 . They are insoluble, non-corrosive, and have high catalytic activity reactions with Cl 2 and O 2 in the electrolyte 108 . It was showed that the optimal pollutant concentration is in a range of 10–100 mg/L, while the maximum could be 200 mg/L 109 . The removal efficiency of the EF process toward heavy metal is limited due to low O 2 evolution overpotentials 107 . Therefore, hybridization between EF, membrane, and EC was a promising approach to improve the overall system performance to remove heavy metals 110 .
The mechanism of removing compounds from wastewater using EO is direct and indirect, as shown in Fig. 7e . The direct mechanism is simple. The performance of the indirect oxidation by chlorine depends on the NaCl concentration and is independent of current intensity 111 . The pollutants exchange electrons directly with the anode surface, and the polymeric layer is formed on the anode surface, leading to electrode deactivation and degrading efficiency. Oxidized contaminants appear in the solution when the organic pollutants interact with oxidants, as shown in Fig. 7e . The following equations describe the EO process:
Supplementary Table 24 lists the efficiency of the EO process at specific operating conditions using different anodes. Pt, Au, Mn, boron-doped diamond (PbO 2 ), and mixed metal oxide (MMO) (such as SnO 2 , PbO 2 , Ti/TiO 2 , IrO 2 , Sb 2 O 5 , RuO 2 ) are widely used as anodes 112 . The main advantages and disadvantages of several anode materials are listed in Table 3 . Highly efficient anode materials are expensive. So, other materials should be proposed to compromise efficiency and cost. Moreover, the presence of different types of metal ions in wastewater affects treatment efficiency. Hence, there is an urgent need to find efficient anode materials with high efficiency in diluted solutions. Future trends should investigate the effectiveness of integrating EO with other water technologies to overcome operational issues. Different electrochemical treatment processes are compared in Table 4 .
Ion exchange treatment
The ion exchange method is a reversible chemical reaction used to replace the undesirable metal ion with harmless and environmentally friendly ones 113 . A heavy metal ion is removed from a wastewater solution by attaching it to an immobile solid particle as a replacement with the solid particle cation, as shown in Fig. 8 . The material of solid ion-exchange particles could be either natural, e.g., inorganic zeolites, or synthetically produced, e.g., organic resins. The ion-exchange method can remove target (some or all) heavy metal ions, such as Pb 2+ , Hg 2+ , Cd 2+ , Ni 2+ , V 4+ , V 5+ , Cr 3+ , Cr 4+ , Cu 2+ , and Zn 2+ from wastewater 113 . The ion exchange mechanism for metal removals can be explained in the following reaction as the ion exchange particle having ion exchanger of M − EC + (M − is the fixed anion and EC + is the exchange cation; Na + and H + are frequently used as exchange cations) to exchange its cation (EC + ) with the wastewater cation (WC + ) 114 .
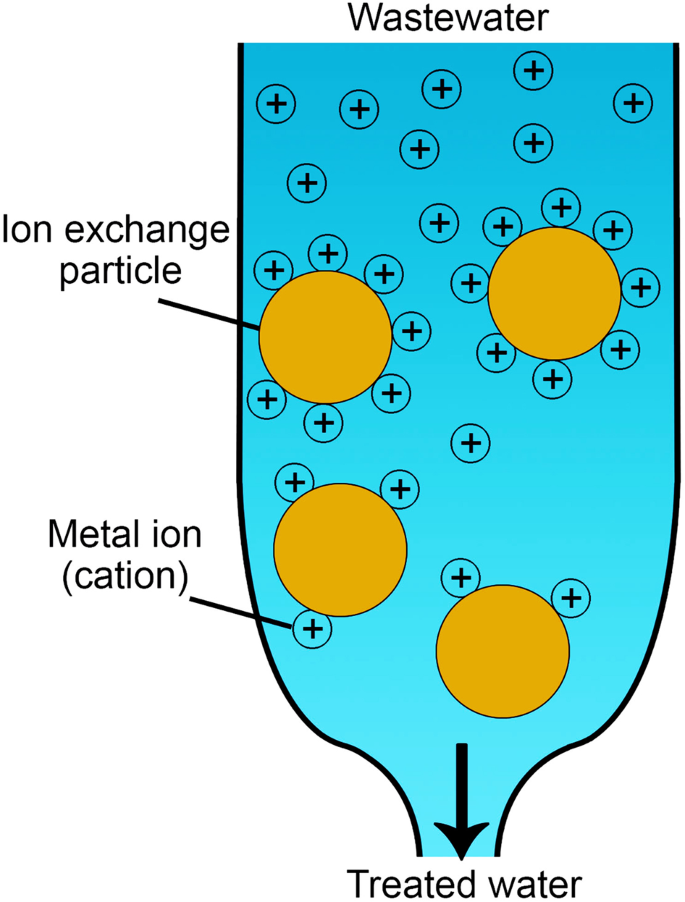
The metal ions (cations) of wastewater occur in the position of those in the ion exchange particles (such as H + and Na + ). Anions could also be removed by this method.
Different types, such as Amberlite 115 and Diaion CR11 116 , were investigated for cations removal. Zeolite has an excellent ion exchange capability owing to its negative charge resulting from Si 4+ , which resides in the middle of the tetrahedron and undergoes isomorphous replacement with Al 3+ cations. MOFs have recently been suggested as good candidates for the ion-exchange removal process 117 . Some reported MOFs used for ion-exchange reactions include AMOF-1 (for Cd 2+ , Pb 2+ , and Hg 2+ removal 118 ), ZIF-8 (for Cu 2+ removal 119 ), and ZIF-67 (for Cr 6+ removal 120 ). Supplementary Table 25 lists some studies that focused on the ion exchange method. Like adsorption, the ion exchange method needs more research on stability and reusability.
Photocatalytic-based separation
The photocatalytic process was reported as a simple process for wastewater treatment that uses light and semiconductors, such as titanium dioxide (TiO 2 ) 121 . Three key steps are taken in this process: charged carrier photogeneration, charged carrier separation and diffusion to the photocatalyst surface, and redox reaction on the photocatalyst surface 122 . The effluents of real soil washing wastewater were treated using an outdoor dual solar photocatalytic process of flat plate collector for the removal of 93.5% Cu 2+ , 99.6% Fe 3+ , and 99.4% Zn 2+ 123 . A simulated ultraviolet (UV)–solar TiO 2 photocatalysis has been used for the removal of ethylenediamine-N, N′-disuccinic acid (EDDS), and Cu 2+ from wastewater (0.2 mM EDDS and 0.2–1.4 mM CuSO 4 ) to obtain 100% conversion efficiency at 24% mineralization degree 124 . In another study, maximum removal efficiencies of 41% Cu 2+ , 100% Fe 3+ , 100% Zn 2+ and 100% EDDS were obtained from synthetic soil washing solution (3.6 × 10 −1 mM EDDS, 8.0 × 10 −2 mM Cu +2 , 1.0 × 10 −1 mM Fe +3 and 8.0 × 10 −2 mM Zn +2 ) 125 .
Using visible light irradiation, a synthesized rhodium/antimony co-doped TiO 2 nano-rod and titanate nanotube (RS-TONR/TNT) was used to extracts Pb 2+ , Cd 2+ , Cu 2+ , Zn 2+ , and organic pollutant from wastewater with 70 and 80% degradation efficiency for dye and Bisphenol A, respectively 126 . The photocatalytic process using CeO 2 /BiOIO 3 composites with Ce 4+ /Ce 3+ redox centers was used to attain 86.53% Hg 2+ removal efficiency under visible light absorption and photocatalytic activity 127 . In an aqueous solution containing As 5+ and Cr 6+ (concentration of 0.10 mg/L), a synthesized 3D-Fe 2 O 3 was used to achieve nearly 100% removal rates using solar light irradiation and photocatalytic activity 128 . A fabricated CH-GEL/ZSPNC hybrid nanocomposite ion exchanger achieved 90% Ni 2+ , 94.9% Zn 2+ , 95% Mg 2+ , 100% Pb 2+ , 90.3% Cd 2+ , 88.9% Cu 2+ and 84% Rhodamine-B (dye) extraction efficiencies using solar light 129 .
A fabricated CS/silver bio-nano-composites (CS/PVDC/Ag) was utilized in photocatalytic oxidation process for 97% Cu 2+ , 88% Pb 2+ , 89% Cd 2+ and 77% dye removal 130 . Although this technology shows the in site generation of reactive radicals, no chemicals used, no sludge production, it has some drawbacks. It is still on a laboratory scale, low throughput, dependent on pH, and inefficient when different metals are present 131 .
Remarks of the treatment methods and future perspectives
It could be figured out from the discussion above that each method used for metal ions removal is not thoroughly preferred and has its advantages and disadvantages. A general comparison between the typical techniques in terms of economic feasibility, technical maturity, less pollution to the environment, and operation control and automaticity is summarized in Fig. 9 .
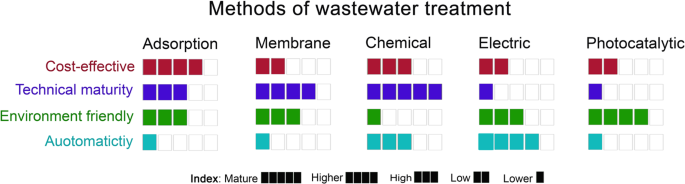
The compared methods are adsorption-, membrane-, chemical-, electric-, and photocatalytic-based in terms of cost-effective, technical maturity, environment friendly, and automaticity, based on the above discussion and the reported studies in the literature 51 , 113 , 131 , 133 , 134 , 135 , 136 , 137 . The electric- and chemical-based methods are represented here for chemical precipitation and electrochemical processes, respectively 133 .
Among all methods, adsorption is the most considered method in recent years. It showed easy operation, low-cost, and high-sorption capacity. Developing eco-friendly and cost-effective adsorbents from wastes is the current research trend. However, disposal of such adsorbents after the adsorption process is a big challenge to avoid environmental risks. Adsorption onto ACs was reported as a feasible method for industrial scale. Adsorption of metal ions from low traces and efficient regeneration processes need additional research. The economic feasibility of industrial applications is also essential.
Membrane methods play a significant role in wastewater treatment and have become a more promising option for wastewater treatment. They are already best-suited for some separation applications such as desalination. Membrane processes are characterized by high metal ions extraction efficiency. However, membrane fouling and biofouling, low recovery for the amount of feed wastewater, process complexity, pre-treatment, periodic membrane cleaning, and high cost are some of the shortcomings of this method. The development of novel membrane materials with enhanced thermal and chemical stability for industrial wastewater treatment is needed in the future to attain better anti-fouling properties and enhance membrane selectivity for the target metals. For both adsorption and membrane methods, the automatic operation of industrial plants needs further implementation and improvement.
Chemical-based separations have widely been used for heavy metal removal owing to their simplicity and low cost. Nevertheless, chemicals are consumed for tuning pH values and enhancing the accumulation of ions. A large-volume sludge is produced that needs further treatment. The electrochemical treatment has the merits of quick treatment, well-controlled, easier sludge removal, and fewer chemicals. However, the high cost of anodes and cathodes, low throughput, high-energy consumption are the main challenges facing this technique. Coupling between different types of electrochemical treatment methods to be driven by renewable energy sources could be promising in addressing this bottleneck. Aerated EC and electrochemical oxidation methods were the best choices to be coupled with other methods due to their ability to eliminate organic and inorganic contaminants from wastewater. The flotation process forms low sludge. So, this method is an excellent candidate to be integrated to build an efficient and cost-effective electrochemical treatment system.
The ion exchange method is similar to the adsorption techniques in which the stability and reusability issues might need more investigation. The photocatalyst method offers simple treatment with no or little chemicals used and no sludge production. However, it is still under research, has low throughput, depends on pH, and is inefficient when different metals are present.
Conclusion and recommendations
Selection of the most appropriate technique for heavy ions removal from wastewater depends on many key factors, including the operation cost, initial concentration of the metal ions, environmental impact, pH values, chemicals added, removal efficiency, and economic feasibility. These methods are classified as adsorption treatments (using different adsorbents, i.e., carbon-based, carbon-composites, minerals, CS, magnetic, biosorbents, and MOFs), membrane treatments (i.e., UF, nanofiltration, microfiltration, reverse osmosis, forward osmosis, and electrodialysis), chemical treatments (i.e., chemical precipitation, coagulation-flocculation, and flotation), electric treatments (i.e., electrochemical (reduction, EC, EF, and advanced oxidation) and ion exchange), and photocatalysis.
Adsorption is the most promising method widely investigated in removing heavy metal ions from wastewater due to simple operation, strong applicability, high removal rate, and low cost of reusability. However, this preference depends mainly on selecting low-cost materials, high uptake, and efficient regeneration processes. Comparing to adsorption, the membrane method is technically more mature as it is practical; however, minimizing the separation cost and membrane fouling is still challenging.
The chemical-based methods, especially chemical precipitation, are technically mature and practical. They are also considered cost-effective methods. They depend on the chemical consumed, unlike the electrochemical method that relies on additional factors, including electrodes, electrical energy, and other fixed costs. However, they produce large volume sludge and need sedimentation separation. The electrochemical process is a relatively expensive technology because of the passivation of electrodes and high electrical energy consumption. Furthermore, electric methods, besides the photocatalytic ones, are the least mature technologies. The merit of the photocatalytic method is that no (or less) chemical consumption and less sludge production, making it eco-friendly.
In general, chemical, adsorption, and membrane methods are the most practical method addressed in the literature. It has been noticed that there is a clear knowledge gap in the performance of treatment methods for the removal of heavy metal ions from real wastewater because most studies used synthetic wastewater in which one or few metal types are present. Accordingly, additional research should be conducted using real wastewater for treating different contaminants. More research on introducing cost-effective materials and methods for heavy metal removal from wastewater should be carried out. Future studies should also focus on the pilot-scale process. The best techniques to achieve efficient metals recovery with less environmental impact and low cost are still under development and should be considered in future research.
Data availability
The authors declare that the data supporting the findings of this study are available within the paper and its supplementary information file.
Change history
07 december 2021.
A Correction to this paper has been published: https://doi.org/10.1038/s41545-021-00144-z
El-Sherif, I. Y., Tolani, S., Ofosu, K., Mohamed, O. A. & Wanekaya, A. K. Polymeric nanofibers for the removal of Cr(III) from tannery waste water. J. Environ. Manag. 129 , 410–413 (2013).
CAS Google Scholar
Zou, Y. et al. Environmental remediation and application of nanoscale zero-valent iron and its composites for the removal of heavy metal ions: a review. Environ. Sci. Technol. 50 , 7290–7304 (2016).
Tjandraatmadja, G. et al. Sources of critical contaminants in domestic wastewater: contaminant contribution from household products. (2008).
Taseidifar, M., Makavipour, F., Pashley, R. M. & Rahman, A. F. M. M. Removal of heavy metal ions from water using ion flotation. Environ. Technol. Innov. 8 , 182–190 (2017).
Google Scholar
García-Niño, W. R. & Pedraza-Chaverrí, J. Protective effect of curcumin against heavy metals-induced liver damage. Food Chem. Toxicol. 69 , 182–201 (2014).
Borba, C. E., Guirardello, R., Silva, E. A., Veit, M. T. & Tavares, C. R. G. Removal of nickel(II) ions from aqueous solution by biosorption in a fixed bed column: Experimental and theoretical breakthrough curves. Biochem. Eng. J. 30 , 184–191 (2006).
Yang, X. et al. Surface functional groups of carbon-based adsorbents and their roles in the removal of heavy metals from aqueous solutions: a critical review. Chem. Eng. J. 366 , 608–621 (2019).
Karnib, M., Kabbani, A., Holail, H. & Olama, Z. Heavy metals removal using activated carbon, silica and silica activated carbon composite. Energy Procedia 50 , 113–120 (2014).
Demiral, İ., Samdan, C. & Demiral, H. Enrichment of the surface functional groups of activated carbon by modification method. Surf. Interfaces 22 , 100873 (2021).
Krishna Kumar, A. S., Jiang, S. J. & Tseng, W. L. Effective adsorption of chromium(vi)/Cr(iii) from aqueous solution using ionic liquid functionalized multiwalled carbon nanotubes as a super sorbent. J. Mater. Chem. A 3 , 7044–7057 (2015).
Duan, C., Ma, T., Wang, J. & Zhou, Y. Removal of heavy metals from aqueous solution using carbon-based adsorbents: a review. J. Water Process Eng. 37 , 101339 (2020).
Marciniak, M., Goscianska, J., Frankowski, M. & Pietrzak, R. Optimal synthesis of oxidized mesoporous carbons for the adsorption of heavy metal ions. J. Mol. Liq. 276 , 630–637 (2019).
Owalude, S. O. & Tella, A. C. Removal of hexavalent chromium from aqueous solutions by adsorption on modified groundnut hull. Beni-Suef Univ. J. Basic Appl. Sci. 5 , 377–388 (2016).
Ngah, W. S. W. & Fatinathan, S. Adsorption of Cu(II) ions in aqueous solution using chitosan beads, chitosan-GLA beads and chitosan-alginate beads. Chem. Eng. J. 143 , 62–72 (2008).
Upadhyay, U., Sreedhar, I., Singh, S. A., Patel, C. M. & Anitha, K. L. Recent advances in heavy metal removal by chitosan based adsorbents. Carbohydr. Polym. 251 , 117000 (2021).
Vakili, M. et al. Novel crosslinked chitosan for enhanced adsorption of hexavalent chromium in acidic solution. Chem. Eng. J. 347 , 782–790 (2018).
Mohammadzadeh Pakdel, P. & Peighambardoust, S. J. Review on recent progress in chitosan-based hydrogels for wastewater treatment application. Carbohydr. Polym. 201 , 264–279 (2018).
Refaat Alawady, A., Ali Alshahrani, A., Ali Aouak, T. & Mohamed Alandis, N. Polysulfone membranes with CNTs/Chitosan biopolymer nanocomposite as selective layer for remarkable heavy metal ions rejection capacity. Chem. Eng. J. 388 , 124267 (2020).
Kazemi, E., Dadfarnia, S., Haji Shabani, A. M. & Ranjbar, M. Synthesis, characterization, and application of a Zn (II)-imprinted polymer grafted on graphene oxide/magnetic chitosan nanocomposite for selective extraction of zinc ions from different food samples. Food Chem. 237 , 921–928 (2017).
Liu, L. et al. Preparation and characterization of chitosan/graphene oxide composites for the adsorption of Au(III) and Pd(II). Talanta 93 , 350–357 (2012).
Li, Y., Li, L. & Yu, J. Applications of zeolites in sustainable. Chem. Chem. 3 , 928–949 (2017).
Zhang, T. et al. Removal of heavy metals and dyes by clay-based adsorbents: from natural clays to 1D and 2D nano-composites. Chem. Eng. J. https://doi.org/10.1016/j.cej.2020.127574 (2020).
Article Google Scholar
Alshameri, A. et al. Understanding the role of natural clay minerals as effective adsorbents and alternative source of rare earth elements: adsorption operative parameters. Hydrometallurgy 185 , 149–161 (2019).
Hao, S. et al. Optimal synthesis of amino-functionalized mesoporous silicas for the adsorption of heavy metal ions. Microporous Mesoporous Mater. 236 , 250–259 (2016).
Li, Z. et al. Disinfection and removal performance for Escherichia coli , toxic heavy metals and arsenic by wood vinegar-modified zeolite. Ecotoxicol. Environ. Saf. 174 , 129–136 (2019).
Hua, M. et al. Heavy metal removal from water/wastewater by nanosized metal oxides: a review. J. Hazard. Mater. 211–212 , 317–331 (2012).
Zhou, W. et al. Construction of MoS2 nanoarrays and MoO3 nanobelts: two efficient adsorbents for removal of Pb(II), Au(III) and Methylene Blue. J. Environ. Sci. 111 , 38–50 (2022).
Behbahani, E. S., Dashtian, K. & Ghaedi, M. Fe3O4-FeMoS4: promise magnetite LDH-based adsorbent for simultaneous removal of Pb (II), Cd (II), and Cu (II) heavy metal ions. J. Hazard. Mater. https://doi.org/10.1016/j.jhazmat.2020.124560 (2020).
Liu, L. et al. Fabrication of novel magnetic core-shell chelating adsorbent for rapid and highly efficient adsorption of heavy metal ions from aqueous solution. J. Mol. Liq. 313 , 113593 (2020).
Costa, H. P. de S., da Silva, M. G. C. & Vieira, M. G. A. Biosorption of aluminum ions from aqueous solutions using non-conventional low-cost materials: a review. J. Water Process Eng. 40 , 101925 (2021).
Abdel Maksoud, M. I. A. et al. Insight on water remediation application using magnetic nanomaterials and biosorbents. Coord. Chem. Rev. 403 , 213096 (2020).
Ai, T., Jiang, X., Liu, Q., Lv, L. & Wu, H. Daptomycin adsorption on magnetic ultra-fine wood-based biochars from water: kinetics, isotherms, and mechanism studies. Bioresour. Technol. 273 , 8–15 (2019).
Gupta, V. K., Ali, I., Saleh, T. A., Siddiqui, M. N. & Agarwal, S. Chromium removal from water by activated carbon developed from waste rubber tires. Environ. Sci. Pollut. Res. 20 , 1261–1268 (2013).
Xu, G. R. et al. Metal organic framework (MOF)-based micro/nanoscaled materials for heavy metal ions removal: the cutting-edge study on designs, synthesis, and applications. Coord. Chem. Rev. 427 , 213554 (2021).
Jamshidifard, S. et al. Incorporation of UiO-66-NH2 MOF into the PAN/chitosan nanofibers for adsorption and membrane filtration of Pb(II), Cd(II) and Cr(VI) ions from aqueous solutions. J. Hazard. Mater. 368 , 10–20 (2019).
Zhang, Y. et al. Insight into the efficient co-removal of Cr(VI) and Cr(III) by positively charged UiO-66-NH2 decorated ultrafiltration membrane. Chem. Eng. J. 404 , 126546 (2021).
Wang, C. et al. Development of mercaptosuccinic anchored MOF through one-step preparation to enhance adsorption capacity and selectivity for Hg(II) and Pb(II). J. Mol. Liq. 317 , 113896 (2020).
Alizadeh, B., Ghorbani, M. & Salehi, M. A. Application of polyrhodanine modified multi-walled carbon nanotubes for high efficiency removal of Pb(II) from aqueous solution. J. Mol. Liq. 220 , 142–149 (2016).
Zhang, Y. et al. Highly efficient adsorption of copper ions by a PVP-reduced graphene oxide based on a new adsorptions mechanism. Nano-Micro Lett. 6 , 80–87 (2014).
Rad, L. R. et al. Removal of Ni2+ and Cd2+ ions from aqueous solutions using electrospun PVA/zeolite nanofibrous adsorbent. Chem. Eng. J. 256 , 119–127 (2014).
Shin, K.-Y., Hong, J.-Y. & Jang, J. Heavy metal ion adsorption behavior in nitrogen-doped magnetic carbon nanoparticles: isotherms and kinetic study. J. Hazard. Mater. 190 , 36–44 (2011).
Dubey, R., Bajpai, J. & Bajpai, A. K. Green synthesis of graphene sand composite (GSC) as novel adsorbent for efficient removal of Cr (VI) ions from aqueous solution. J. Water Process Eng. 5 , 83–94 (2015).
Hayati, B. et al. Heavy metal adsorption using PAMAM/CNT nanocomposite from aqueous solution in batch and continuous fixed bed systems. Chem. Eng. J. 346 , 258–270 (2018).
Karami, H. Heavy metal removal from water by magnetite nanorods. Chem. Eng. J. 219 , 209–216 (2013).
Malik, R., Dahiya, S. & lata, S. An experimental and quantum chemical study of removal of utmostly quantified heavy metals in wastewater using coconut husk: a novel approach to mechanism. Int. J. Biol. Macromol. 98 , 139–149 (2017).
Alijani, H. & Shariatinia, Z. Synthesis of high growth rate SWCNTs and their magnetite cobalt sulfide nanohybrid as super-adsorbent for mercury removal. Chem. Eng. Res. Des. 129 , 132–149 (2018).
Liu, L. et al. Surface charge of mesoporous calcium silicate and its adsorption characteristics for heavy metal ions. Solid State Sci. 99 , 106072 (2020).
Yi, X. et al. Graphene oxide encapsulated polyvinyl alcohol/sodium alginate hydrogel microspheres for Cu (II) and U (VI) removal. Ecotoxicol. Environ. Saf. 158 , 309–318 (2018).
Yi, X. et al. Encapsulating Fe3O4 into calcium alginate coated chitosan hydrochloride hydrogel beads for removal of Cu (II) and U (VI) from aqueous solutions. Ecotoxicol. Environ. Saf. 147 , 699–707 (2018).
Zeng, J. et al. Chitosan functionalized iron nanosheet for enhanced removal of As (III) and Sb (III): Synergistic effect and mechanism. Chem. Eng. J . 122999 (2019).
Rahmati, N. O., Pourafshari Chenar, M. & Azizi Namaghi, H. Recent trends of heavy metal removal from water/wastewater by membrane technologies. J. Ind. Eng. Chem. 76 , 17–38 (2019).
Rahmati, N. O., Pourafshari Chenar, M. & Azizi Namaghi, H. Removal of free active chlorine from synthetic wastewater by MEUF process using polyethersulfone/titania nanocomposite membrane. Sep. Purif. Technol. 181 , 213–222 (2017).
Huang, J. et al. Repeating recovery and reuse of SDS micelles from MEUF retentate containing Cd2+ by acidification UF. Colloids Surf. A Physicochem. Eng. Asp. 520 , 361–368 (2017).
Tanhaei, B. et al. Simultaneous removal of aniline and nickel from water by micellar-enhanced ultrafiltration with different molecular weight cut-off membranes. Sep. Purif. Technol. 124 , 26–35 (2014).
Fu, F. & Wang, Q. Removal of heavy metal ions from wastewaters: A review. J. Environ. Manag. 92 , 407–418 (2011).
Paulino, A. T. et al. Novel adsorbent based on silkworm chrysalides for removal of heavy metals from wastewaters. J. Colloid Interface Sci. 301 , 479–487 (2006).
Oyaro, N., Juddy, O., Murago, E. N. M. & Gitonga, E. The contents of Pb, Cu, Zn and Cd in meat in Nairobi, Kenya. J. Food, Agric. Environ. 5 , 119–121 (2007).
Rivas, BernabéL. & Eduardo Pereira, A. M. Functional water-soluble polymers: polymer–metal ion removal and biocide properties. Polym. Int. 58 , 1093–1114 (2009).
Huda, N., Nik, B., Nik, M., Mohamed, S. & Aroua, K. Removal of heavy metal ions from mixed solutions via polymer-enhanced ultrafiltration using starch as a water-soluble biopolymer. Environ. Prog. Sustain. Energy 34 , 359–367 (2015).
Wang, L. K., Chen, J. P., Hung, Y.-T. & Shammas, N. K. Membrane and Desalination Technologies. Membrane and Desalination Technologies vol. 13 (2011). Springer.
Jamil, T. S., Mansor, E. S., Abdallah, H., Shaban, A. M. & Souaya, E. R. Novel anti fouling mixed matrix CeO 2 /Ce7O 12 nanofiltration membranes for heavy metal uptake. J. Environ. Chem. Eng. 6 , 3273–3282 (2018).
Ozaki, H., Sharma, K. & Saktaywin, W. Performance of an ultra-low-pressure reverse osmosis membrane (ULPROM) for separating heavy metal: effects of interference parameters. Desalination 144 , 287–294 (2002).
Samaei, S. M., Gato-Trinidad, S. & Altaee, A. Performance evaluation of reverse osmosis process in the post-treatment of mining wastewaters: Case study of Costerfield mining operations, Victoria, Australia. J. Water Process Eng. 34 , 101116 (2020).
He, M. et al. Novel polydopamine/metal organic framework thin film nanocomposite forward osmosis membrane for salt rejection and heavy metal removal. Chem. Eng. J. 389 , 124452 (2020).
Cui, Y., Ge, Q., Liu, X.-Y. & Chung, T.-S. Novel forward osmosis process to effectively remove heavy metal ions. J. Memb. Sci. 467 , 188–194 (2014).
Al-Amshawee, S. et al. Electrodialysis desalination for water and wastewater: a review. Chem. Eng. J. 380 , 122231 (2020).
Nemati, M., Hosseini, S. M. & Shabanian, M. Novel electrodialysis cation exchange membrane prepared by 2-acrylamido-2-methylpropane sulfonic acid; heavy metal ions removal. J. Hazard. Mater. 337 , 90–104 (2017).
Gherasim, C. V., Křivčík, J. & Mikulášek, P. Investigation of batch electrodialysis process for removal of lead ions from aqueous solutions. Chem. Eng. J. 256 , 324–334 (2014).
Min, K. J., Kim, J. H. & Park, K. Y. Characteristics of heavy metal separation and determination of limiting current density in a pilot-scale electrodialysis process for plating wastewater treatment. Sci. Total Environ. 757 , 143762 (2021).
Basha, C. A., Selvi, S. J., Ramasamy, E. & Chellammal, S. Removal of arsenic and sulphate from the copper smelting industrial effluent. Chem. Eng. J. 141 , 89–98 (2008).
Santos, P. G., Scherer, C. M., Fisch, A. G. & Rodrigues, M. A. S. Petrochemical wastewater treatment: water recovery using membrane distillation. J. Clean. Prod. 267 , 121985 (2020).
Qu, D. et al. Experimental study of arsenic removal by direct contact membrane distillation. J. Hazard. Mater. 163 , 874–879 (2009).
Zaheri, P., Mohammadi, T., Abolghasemi, H. & Ghannadi Maraghe, M. Supported liquid membrane incorporated with carbon nanotubes for the extraction of Europium using Cyanex272 as carrier. Chem. Eng. Res. Des. 100 , 81–88 (2015).
Jean, E., Villemin, D., Hlaibi, M. & Lebrun, L. Heavy metal ions extraction using new supported liquid membranes containing ionic liquid as carrier. Sep. Purif. Technol. 201 , 1–9 (2018).
De Los Ríos, A. P. et al. On the selective separation of metal ions from hydrochloride aqueous solution by pertraction through supported ionic liquid membranes. J. Memb. Sci. 444 , 469–481 (2013).
Ojovan, M. I., Lee, W. E. & Kalmykov, S. N. in Treatment of Radioactive Wastes . (eds. Ojovan, M. I., Lee, W. E. & Kalmykov, S. N. B. T.-A. I. to N. W. I. (Third E.) Ch. 16, 231–269 (Elsevier, 2019).
Yadav, M., Gupta, R. & Sharma, R. K. in Green and Sustainable Pathways for Wastewater Purification . (ed. Ahuja, S. B. T.-A. in W. P. T.) 355–383 (Elsevier, 2019).
Park, J.-H., Choi, G.-J. & Kim, S.-H. Effects of pH and slow mixing conditions on heavy metal hydroxide precipitation. J. Korea. Org. Res. Recycl. Assos . 22 , 50–56 (2014).
Kurniawan, T. A., Chan, G. Y. S., Lo, W.-H. & Babel, S. Physico–chemical treatment techniques for wastewater laden with heavy metals. Chem. Eng. J. 118 , 83–98 (2006).
Al-Hemaidi, W. K. Approach in choosing suitable technology for industrial wastewater. J. Civil & Envir. Eng. 2 , 1000123 (2012).
Anotai, J., Tontisirin, P. & Churod, P. Integrated treatment scheme for rubber thread wastewater: sulfide precipitation and biological processes. J. Hazard. Mater. 141 , 1–7 (2007).
Patterson, J. W., Allen, H. E. & Scala, J. J. Carbonate precipitation for heavy metals pollutants. J. Water Pollut. Control Federation 49 , 2397–2410 (1977).
Zueva, S. B. in Waste Electrical and Electronic Equipment Recycling: Aqueous Recovery Methods (eds. Vegliò, F. & Birloaga, I. B. T.-W. E. and E. E. R.) 213–240 (Woodhead Publishing, 2018).
Kremer, M. L. The fenton reaction. Dependence of the rate on pH. J. Phys. Chem. A 107 , 1734–1741 (2003).
Bossmann, S. H. et al. New evidence against hydroxyl radicals as reactive intermediates in the thermal and photochemically enhanced fenton reactions. J. Phys. Chem. A 102 , 5542–5550 (1998).
Fu, F., Wang, Q. & Tang, B. Effective degradation of C.I. Acid Red 73 by advanced Fenton process. J. Hazard. Mater. 174 , 17–22 (2010).
Wang, S. A Comparative study of Fenton and Fenton-like reaction kinetics in decolourisation of wastewater. Dye. Pigment. 76 , 714–720 (2008).
Namkung, K. C., Burgess, A. E., Bremner, D. H. & Staines, H. Advanced Fenton processing of aqueous phenol solutions: a continuous system study including sonication effects. Ultrason. Sonochem. 15 , 171–176 (2008).
Ibarra-Rodríguez, D., Lizardi-Mendoza, J., López-Maldonado, E. A. & Oropeza-Guzmán, M. T. Capacity of ‘nopal’ pectin as a dual coagulant-flocculant agent for heavy metals removal. Chem. Eng. J. 323 , 19–28 (2017).
Chang, Q., Zhang, M. & Wang, J. Removal of Cu2+ and turbidity from wastewater by mercaptoacetyl chitosan. J. Hazard. Mater. 169 , 621–625 (2009).
Nourani, M., Baghdadi, M., Javan, M. & Bidhendi, G. N. Production of a biodegradable flocculant from cotton and evaluation of its performance in coagulation-flocculation of kaolin clay suspension: Optimization through response surface methodology (RSM). J. Environ. Chem. Eng. 4 , 1996–2003 (2016).
Teh, C. Y., Budiman, P. M., Shak, K. P. Y. & Wu, T. Y. Recent advancement of coagulation-flocculation and its application in wastewater treatment. Ind. Eng. Chem. Res. 55 , 4363–4389 (2016).
Edzwald, J. K. Dissolved air flotation and me. Water Res. 44 , 2077–2106 (2010).
Peng, W. et al. An overview on the surfactants used in ion flotation. J. Mol. Liq. 286 , 110955 (2019).
Hoseinian, F. S., Rezai, B., Kowsari, E., Chinnappan, A. & Ramakrishna, S. Synthesis and characterization of a novel nanocollector for the removal of nickel ions from synthetic wastewater using ion flotation. Sep. Purif. Technol. 240 , 116639 (2020).
Salmani, M. H., Davoodi, M., Ehrampoush, M. H., Ghaneian, M. T. & Fallahzadah, M. H. Removal of cadmium (II) from simulated wastewater by ion flotation technique. Iran. J. Environ. Heal. Sci. Eng. 10 , 16 (2013).
Mahne, E. J. & Pinfold, T. A. Precipitate flotation I. Removal of nickel from dilute aqueous solutions and its separation from cobalt. J. Appl. Chem. 18 , 52–54 (2007).
Pretorius, W. A., Johannes, W. G. & Lempert, G. G. Electrolytic iron flocculant production with a bipolar electrode in series arrangement. Water SA 17 , 133–138 (1991).
Yang, X., Liu, L., Tan, W., Qiu, G. & Liu, F. High-performance Cu2+ adsorption of birnessite using electrochemically controlled redox reactions. J. Hazard. Mater. 354 , 107–115 (2018).
Jin, W., Fu, Y., Hu, M., Wang, S. & Liu, Z. Highly efficient SnS-decorated Bi 2 O 3 nanosheets for simultaneous electrochemical detection and removal of Cd(II) and Pb(II). J. Electroanal. Chem. 856 , 113744 (2020).
Baghban, E., Mehrabani-Zeinabad, A. & Moheb, A. The effects of operational parameters on the electrochemical removal of cadmium ion from dilute aqueous solutions. Hydrometallurgy 149 , 97–105 (2014).
Moussa, D. T., El-Naas, M. H., Nasser, M. & Al-Marri, M. J. A comprehensive review of electrocoagulation for water treatment: potentials and challenges. J. Environ. Manag. 186 , 24–41 (2017).
Liu, L. et al. High-efficiency As(III) oxidation and electrocoagulation removal using hematite with a charge−discharge technique. Sci. Total Environ. 703 , 135678 (2020).
Zaied, B. K. et al. A comprehensive review on contaminants removal from pharmaceutical wastewater by electrocoagulation process. Sci.Total Environ. 726 , 138095 (2020).
Ingelsson, M., Yasri, N. & Roberts, E. P. L. Electrode passivation, faradaic efficiency, and performance enhancement strategies in electrocoagulation—a review. Water Res. 187 , 116433 (2020).
Sillanpää, M. & Shestakova, M. In Electrochemical Water Treatment Methods: Fundamentals, Methods and Full Scale Applications (eds. Martínez-Huitle, C. A., Rodrigo, M. A., & Scialdone, O.) 47–130 (ScienceDirect, 2017).
Chen, X., Chen, G. & Yue, P. L. Novel electrode system for electroflotation of wastewater. Environ. Sci. Technol. 36 , 778–783 (2002).
Kolesnikov, V. A., Il’in, V. I. & Kolesnikov, A. V. Electroflotation in wastewater treatment from oil products, dyes, surfactants, ligands, and biological pollutants: a review. Theor. Found. Chem. Eng. 53 , 251–273 (2019).
Shammas, N. K., Wang, L. K. & Hahn, H. H. in Flotation Technology (eds. Lawrence, K. W., Shammas, N. K., Selke, W. A., & Aulenbach, D. B.) 121–164 (Springer, 2010).
Mazumder, A., Chowdhury, Z., Sen, D. & Bhattacharjee, C. Electric field assisted membrane separation for oily wastewater with a novel and cost-effective electrocoagulation and electroflotation enhanced membrane module (ECEFMM). Chem. Eng. Process. - Process Intensif . 151 , (2020).
Ammar, H. B., Brahim, M., Ben, Abdelhédi, R. & Samet, Y. Green electrochemical process for metronidazole degradation at BDD anode in aqueous solutions via direct and indirect oxidation. Sep. Purif. Technol. 157 , 9–16 (2016).
Martínez-Huitle, C. A. & Ferro, S. Electrochemical oxidation of organic pollutants for the wastewater treatment: direct and indirect processes. Chem. Soc. Rev. 35 , 1324–1340 (2006).
Da̧browski, A., Hubicki, Z., Podkościelny, P. & Robens, E. Selective removal of the heavy metal ions from waters and industrial wastewaters by ion-exchange method. Chemosphere 56 , 91–106 (2004).
Tenório, J. A. S. & Espinosa, D. C. R. Treatment of chromium plating process effluents with ion exchange resins. Waste Manag. 21 , 637–642 (2001).
Kang, S.-Y., Lee, J.-U., Moon, S.-H. & Kim, K.-W. Competitive adsorption characteristics of Co2+, Ni2+, and Cr3+ by IRN-77 cation exchange resin in synthesized wastewater. Chemosphere 56 , 141–147 (2004).
Cavaco, S. A., Fernandes, S., Quina, M. M. & Ferreira, L. M. Removal of chromium from electroplating industry effluents by ion exchange resins. J. Hazard. Mater. 144 , 634–638 (2007).
Kobielska, P. A., Howarth, A. J., Farha, O. K. & Nayak, S. Metal–organic frameworks for heavy metal removal from water. Coord. Chem. Rev. 358 , 92–107 (2018).
Fang, Q.-R. R. et al. Functional mesoporous metal-organic frameworks for the capture of heavy metal ions and size-selective catalysis. Inorg. Chem. 49 , 11637–11642 (2010).
Zhang, Y. et al. Unveiling the adsorption mechanism of zeolitic imidazolate framework-8 with high efficiency for removal of copper ions from aqueous solutions. Dalt. Trans. 45 , 12653–12660 (2016).
Li, X., Gao, X., Ai, L. & Jiang, J. Mechanistic insight into the interaction and adsorption of Cr(VI) with zeolitic imidazolate framework-67 microcrystals from aqueous solution. Chem. Eng. J. 274 , 238–246 (2015).
Barakat, M. A. New trends in removing heavy metals from industrial wastewater. Arab. J. Chem. 4 , 361–377 (2011).
Nasir, A. M. et al. A review on floating nanocomposite photocatalyst: fabrication and applications for wastewater treatment. J. Water Process Eng. 36 , 101300 (2020).
Onotri, L. et al. Solar photocatalytic processes for treatment of soil washing wastewater. Chem. Eng. J. 318 , 10–18 (2017).
Satyro, S. et al. Removal of EDDS and copper from waters by TiO2 photocatalysis under simulated UV-solar conditions. Chem. Eng. J. 251 , 257–268 (2014).
Satyro, S. et al. Simulated solar photocatalytic processes for the simultaneous removal of EDDS, Cu(II), Fe(III) and Zn(II) in synthetic and real contaminated soil washing solutions. J. Environ. Chem. Eng. 2 , 1969–1979 (2014).
Dhandole, L. K. et al. Simultaneous and synergistic effect of heavy metal adsorption on the enhanced photocatalytic performance of a visible-light-driven RS-TONR/TNT composite. Environ. Res. 180 , 108651 (2020).
Xiao, Y. et al. CeO2/BiOIO3 heterojunction with oxygen vacancies and Ce4+/Ce3+ redox centers synergistically enhanced photocatalytic removal heavy metal. Appl. Surf. Sci. 530 , 147116 (2020).
Lee, S. C. et al. Hierarchically three-dimensional (3D) nanotubular sea urchin-shaped iron oxide and its application in heavy metal removal and solar-induced photocatalytic degradation. J. Hazard. Mater. 354 , 283–292 (2018).
Kaur, K. & Jindal, R. Synergistic effect of organic-inorganic hybrid nanocomposite ion exchanger on photocatalytic degradation of Rhodamine-B dye and heavy metal ion removal from industrial effluents. J. Environ. Chem. Eng. 6 , 7091–7101 (2018).
Al-Sherbini, A. S. A., Ghannam, H. E. A., El-Ghanam, G. M. A., El-Ella, A. A. & Youssef, A. M. Utilization of chitosan/Ag bionanocomposites as eco-friendly photocatalytic reactor for Bactericidal effect and heavy metals removal. Heliyon 5 , e01980 (2019).
Crini, G. & Lichtfouse, E. Advantages and disadvantages of techniques used for wastewater treatment. Environ. Chem. Lett. 17 , 145–155 (2019).
WHO. Guidelines for drinking-water quality: Fourthedition incorporating the first addendum . Guidelines for Drinking-Water Quality: Fourth Edition Incorporating the First Addendum (2014).
Qiu, B. et al. Biochar as a low-cost adsorbent for aqueous heavy metal removal: a review. J. Anal. Appl. Pyrolysis 155 , 105081 (2021).
Kumar, M., Nandi, M. & Pakshirajan, K. Recent advances in heavy metal recovery from wastewater by biogenic sulfide precipitation. J. Environ. Manag. 278 , 111555 (2021).
Martínez-Huitle, C. A. & Panizza, M. Electrochemical oxidation of organic pollutants for wastewater treatment. Curr. Opin. Electrochem. 11 , 62–71 (2018).
Hu, K., Xu, D. & Chen, Y. An assessment of sulfate reducing bacteria on treating sulfate-rich metal-laden wastewater from electroplating plant. J. Hazard. Mater. 393 , 122376 (2020).
Burakov, A. E. et al. Adsorption of heavy metals on conventional and nanostructured materials for wastewater treatment purposes: a review. Ecotoxicol. Environ. Saf. 148 , 702–712 (2018).
Download references
Acknowledgements
The authors would like to thank the Deanship of Research Oversight and Coordination at King Fahd University of Petroleum and Minerals (KFUPM), Dhahran, Saudi Arabia, for supporting this research.
Author information
Authors and affiliations.
Department of Aerospace Engineering, King Fahd University of Petroleum & Minerals (KFUPM), Dhahran, Saudi Arabia
Naef A. A. Qasem
Interdisciplinary Research Center for Membranes and Water Security, King Fahd University of Petroleum & Minerals (KFUPM), Dhahran, Saudi Arabia
Naef A. A. Qasem & Dahiru U. Lawal
Department of Mechanical Power Engineering, Zagazig University, Zagazig, Egypt
Ramy H. Mohammed
You can also search for this author in PubMed Google Scholar
Contributions
N.A.A.Q.: Conceptualization, Literature review, Data analysis, Editing, Revision, Writing. R.H.M.: Literature review, Data analysis, Editing, Writing. D.U.L.: Literature review, Data analysis, Editing, Writing.
Corresponding author
Correspondence to Naef A. A. Qasem .
Ethics declarations
Competing interests.
The authors declare no competing interests.
Additional information
Publisher’s note Springer Nature remains neutral with regard to jurisdictional claims in published maps and institutional affiliations.
Supplementary information
Supplementary information, rights and permissions.
Open Access This article is licensed under a Creative Commons Attribution 4.0 International License, which permits use, sharing, adaptation, distribution and reproduction in any medium or format, as long as you give appropriate credit to the original author(s) and the source, provide a link to the Creative Commons license, and indicate if changes were made. The images or other third party material in this article are included in the article’s Creative Commons license, unless indicated otherwise in a credit line to the material. If material is not included in the article’s Creative Commons license and your intended use is not permitted by statutory regulation or exceeds the permitted use, you will need to obtain permission directly from the copyright holder. To view a copy of this license, visit http://creativecommons.org/licenses/by/4.0/ .
Reprints and permissions
About this article
Cite this article.
Qasem, N.A.A., Mohammed, R.H. & Lawal, D.U. Removal of heavy metal ions from wastewater: a comprehensive and critical review. npj Clean Water 4 , 36 (2021). https://doi.org/10.1038/s41545-021-00127-0
Download citation
Received : 18 February 2021
Accepted : 17 June 2021
Published : 08 July 2021
DOI : https://doi.org/10.1038/s41545-021-00127-0
Share this article
Anyone you share the following link with will be able to read this content:
Sorry, a shareable link is not currently available for this article.
Provided by the Springer Nature SharedIt content-sharing initiative
This article is cited by
Combined advanced oxidation dye-wastewater treatment plant: design and development with data-driven predictive performance modeling.
- Pankaj Singh Chauhan
- Kirtiman Singh
- Shantanu Bhattacharya
npj Clean Water (2024)
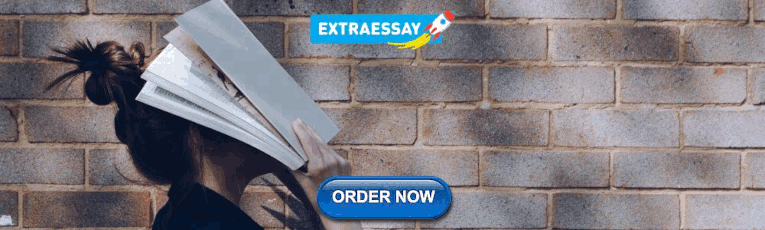
Isolation, characterization, identification, genomics and analyses of bioaccumulation and biosorption potential of two arsenic-resistant bacteria obtained from natural environments
- Barnan Kumar Saha
Scientific Reports (2024)
- Ghufran Ahmed
- Ashish Kumar
Adsorption of lead and chromium ions from electroplating wastewater using plantain stalk modified by amorphous alumina developed from waste cans
- E. O. Ajala
- M. O. Aliyu
- T. S. Olatunde
Associations of the utilization of household water treatment devices with mortality
- Shuai-Wen Huang
- Hong-Lian Zhou
Quick links
- Explore articles by subject
- Guide to authors
- Editorial policies
Sign up for the Nature Briefing newsletter — what matters in science, free to your inbox daily.


An official website of the United States government
The .gov means it’s official. Federal government websites often end in .gov or .mil. Before sharing sensitive information, make sure you’re on a federal government site.
The site is secure. The https:// ensures that you are connecting to the official website and that any information you provide is encrypted and transmitted securely.
- Publications
- Account settings
Preview improvements coming to the PMC website in October 2024. Learn More or Try it out now .
- Advanced Search
- Journal List
- Bioengineered
- v.14(1); 2023
- PMC10376923
Recent advances in microbial engineering approaches for wastewater treatment: a review
Monika sharma.
a Department of Zoology, University of Jammu, Jammu and Kashmir, India
Sangita Agarwal
b Department of Applied Science, RCC Institute of Information Technology Kolkata, West Bengal, India
Richa Agarwal Malik
c Department of Environmental Studies, PGDAV College, University of Delhi, New Delhi, India
Gaurav Kumar
Dan bahadur pal.
d Department of Chemical Engineering, Harcourt Butler Technical University, Kanpur, Uttar Pradesh, India
Mamun Mandal
e Laboratory of Applied Stress Biology, Department of Botany, University of Gour Banga, Malda, West Bengal, India
Abhijit Sarkar
Farkad bantun.
f Department of Microbiology, Faculty of Medicine, Umm Al-Qura University, Makkah, Saudi Arabia
Shafiul Haque
g Research and Scientific Studies Unit, College of Nursing and Allied Health Sciences, Jazan University, Jazan, Saudi Arabia
j Gilbert and Rose-Marie Chagoury School of Medicine, Lebanese American University, Beirut, Lebanon
k Centre of Medical and Bio-Allied Health Sciences Research, Ajman University, Ajman, United Arab Emirates
Pardeep Singh
Neha srivastava.
h Department of Chemical Engineering and Technology, Indian Institute of Technology (BHU), Varanasi, India
Vijai Kumar Gupta
i Biorefining and Advanced Materials Research Center, SRUC, UK
In the present era of global climate change, the scarcity of potable water is increasing both due to natural and anthropogenic causes. Water is the elixir of life, and its usage has risen significantly due to escalating economic activities, widespread urbanization, and industrialization. The increasing water scarcity and rising contamination have compelled, scientists and researchers, to adopt feasible and sustainable wastewater treatment methods in meeting the growing demand for freshwater. Presently, various waste treatment technologies are adopted across the globe, such as physical, chemical, and biological treatment processes. There is a need to replace these technologies with sustainable and green technology that encourages the use of microorganisms since they have proven to be more effective in water treatment processes. The present review article is focused on demonstrating how effectively various microbes can be used in wastewater treatment to achieve environmental sustainability and economic feasibility. The microbial consortium used for water treatment offers many advantages over pure culture. There is an urgent need to develop hybrid treatment technology for the effective remediation of various organic and inorganic pollutants from wastewater.
- Microbial engineering approaches for wastewater treatment.
- Current and emerging sources of water pollution are discussed.
- Various treatment technologies for wastewater treatment.
- Biological methods and microbes are used for degradation.
- Parameters responsible for the degradations processes of wastewater.
GRAPHICAL ABSTRACT

1. Introduction
World water consumption has almost doubled in a few decades [ 1 ]. The growing concerns over water contamination have led to extensive research and development in water treatment techniques. They are expanding to promote the reuse of water and improve the quality of water for human consumption. Water pollution is a worldwide issue that presents a severe threat to the survival of all life forms. Aquatic pollution can be caused by organic and inorganic impurities and microbiological contaminants. Population growth, industrial and mining activities, sewage and wastewater, radioactive waste, chemical fertilizers, pesticides, urban development, and other anthropogenic sources are all responsible for rising levels of aquatic pollution. Water quality is determined by the concentrations of particles and chemicals in water, such as heavy metals, nutrients, microorganisms, polycyclic aromatic hydrocarbon (PAH), and other pollutants. Many organic contaminants are endocrine-disrupting chemicals associated with testicular, prostate, and breast cancers. They can also cause serious complications in human and animal reproductive health, such as sperm count reduction in males and the production of fragile eggs in females, among other things [ 2 ].
Wastewater treatment has evolved as a feasible technique for tackling water scarcity and protecting the ecosystem from the harmful impacts of polluted/wastewater in the contemporary environment [ 3 ]. Many countries have passed stricter laws for treating sewage water before dumping it into water bodies. From sustainability, improved water management and wastewater recycling have begun to get active attention [ 4 ]. Many physical and chemical processes (flotation, precipitation, oxidation, adsorption, etc.) used for wastewater treatment are expensive, demand high maintenance, and require a complicated functional setup. As a result, there is an urgent necessity to move to green and sustainable technologies such as microbial wastewater treatment to serve as a long-term alternative to traditional wastewater treatment methods [ 3 ]. Wastewater treatment using microbes such as fungi, bacteria, microalgae, and others has caught the researcher’s attention in recent years. The presence of a significant amount of nutrients such as nitrogen, phosphorus, and carbon in wastewater emanating from different sources can aid in the growth and survival of these microbes [ 5 ].
Phytoremediation is frequently used to remediate wastewater. On the other hand, excessive salts in wastewater can induce plant toxicity. To solve the problem, Sarawaneeyaruk et al. [ 6 ] isolated multifarious plant growth-promoting bacteria (PGPB) Bacillus spp from the municipal wastewater and by using this PGPB they successfully enhanced plant growth under municipal wastewater irrigation. Hence, such green technology would be sustainable and help maintain a balance between socio-economic and environmental perspectives [ 3 ].
Food, water, and energy are interconnected and wastewater is crucial in this nexus. Although various wastewater treatment methods are available the aim of this review is to draw attention on the importance of microbes in wastewater. The goal of this review is to help in protecting water resources using effective treatment method. This review illustrates the wastewater treatment process by utilizing microorganisms (bacteria, fungi, microalgae, and yeast), highlighting the advantages and applications of microbes over other conventional approaches. This review further aims to provide useful information to researchers working in relevant fields worldwide to pique their interest in using microbes to improve and cost-effectively treat wastewater ( Table 1 ).
Categories of water pollutants and their probable effects are tabulated as.
2. Emerging sources of water pollution
A variety of organic contaminants can be found in water, including insecticides, herbicides and organohalides [ 7 ]. Industrial effluents contain inorganic pollutants such as silt from stormwater runoff and heavy metals from acid mine drainage. Different sources of domestic waste enter water bodies. Pesticides used in gardens and lawns may also enter the water bodies [ 8 ]. Cleaning products, detergents, and skincare products contain significant amounts of pollutants that can pollute water bodies and make them unfit for human consumption [ 9 ]. Chemicals and acids from industries like steel and paper are discharged into rivers [ 10 ]. Water bodies receive over 70% of industrial waste, containing many toxins [ 8 ]. Major agricultural wastes are fertilizers, pesticides (herbicides, insecticides), and other agrochemicals. Fertilizer production continues to rise year after year to increase productivity, resulting in increased waste generation. Irrigation contributes significantly to surface water pollution in China and is also a cause of nitrogen groundwater pollution in the United States [ 9 ]. Toxic chemicals can accumulate in the body and eventually reach toxic levels, causing food chain disruption. Another major source of water pollution is nutrient enrichment.
3. Emerging technologies for wastewater treatment
Physical treatment of wastewater entails removing contaminants from the water without affecting the biochemical properties of contaminants. Physical treatments usually follow chemical and biological treatments. Screening, Flotation, Flow equalization, Membrane-based technology, Thermal treatment, and other physical treatment techniques are commonly used. Some of the common chemical unit processes used in wastewater treatment technology include precipitation, adsorption, disinfection, chlorination, neutralization, chemical exchange, etc. [ 14 ] to bring changes in the quality of water.
3.1. Biological treatment for the wastewater
Biological treatment involves removing contaminants from wastewater using biological organisms or processes. Microbes are critical to wastewater treatment and reclamation, making them a promising green technology tool. The biological treatment uses bacteria, fungi, microalgae, yeast, and other microbial groups. Biological treatments are less expensive than physical and chemical treatments Table 2 [ 15 ]. Among the most widely used biological wastewater treatment methods are:
Showing advantages of biological methods in wastewater treatment [ 17 ].
3.1.1. Activated sludge method
One of the most commonly used biological processes in wastewater treatment to date is the activated sludge. It has been effectively used to treat industrial and municipal wastewater. This process uses a biological floc that consists of bacteria and protozoa under aerobic conditions [ 16 ]. The basic principle behind all activated sludge processes is that microbes grow within metabolizing organic material where they form clumps. The oxidizable matter is used as food for the microorganisms forming a suspended floc in the wastewater. The aeration/agitation provides the continued oxygen supply. The mixed liquor, which is the mix of wastewater and activated sludge, is allowed to settle down to segregate the activated sludge solids from treated wastewater while a part of the settled activated sludge is returned to the aeration site. The entire activated sludge process comprises interlinked elements such as an aeration tank, source of aeration, clarifier, and a collection system. The biological reaction occurs in an aeration tank fitted with a stirrer for mixing and source of oxygen, which is connected to a tank/clarifier where the settled solids are segregated from treated water along with a collection loop which either returns the activated sludge back to the aeration tank or is removed from the process. The process has high efficiency and can also be used for nutrient removal [ 18 ].
The activated sludge process is used in the treatment of industrial wastewater as well as domestic. In spite of having advantages like low operational cost with added treatment efficiency, the major drawback is the generation of excess quantity of waste activated sludge. The organic matter generated by the process needs to be properly treated and managed to reduce the ecological and financial burden [ 19 ].
3.1.2. Bioreactors and biofilters
Using physical retention and microbial biodegradation, membrane bioreactors eliminate pollutants from wastewater [ 20 ]. Biofilters use biological processes to filter wastewater [ 21 ]. It grows on top of the media, which is composed of gravel, sand, and ceramic. For example, a biofilm can contain a microbial (bacterial) community that helps to decompose organic content in water [ 37 ]. This process has been extensively used to remove H 2 S from municipal wastewater, according to Zhang et al. [ 38 ].
3.1.3. Biosorption
Certain biological molecules naturally can accumulate metals like copper, zinc, nickel, chromium, palladium from wastewater [ 39 ]. The process of biosorption is complex and involves various interactive mechanisms like ion-exchange, absorption, precipitation, and complexation through the participation of functional groups like hydroxyl, carbonyl, etc. [ 40 ]. It is a reversible process that involves interactions rather than oxidation to bind the biosorbent in an aqueous solution.[ 41 ]
The biosorbent is suspended in a contaminant solution (e.g. metal ions). After a while, contaminant-rich biosorbent can be separated. Microbes immobilize on adsorbants to form a biosorbent that captures contaminants [ 42 ].
Agricultural waste, microbial biomasses, industrial-by-products offer advantages over chemical methods in terms of efficiency, large abundance and low cost [ 43 ]. Factors like pH, concentration of the metals, ionic strength, other pollutants present in wastewater, temperature, etc., effect the process of biosorption [ 44 ]
4. Different microbial groups in wastewater degradation
Microbial treatment can be used instead of traditional wastewater treatment methods because it is cheaper, more efficient, and more competent [ 45 ]. Bioremediation involves bacteria, fungi, microalgae, yeast, etc. [ 46 ]. These microbes are responsible for degrading or converting contaminants into lesser harmful products [ 47 ]. They have become ideal bioagents of remediation owing to their high surface area-to-volume ratio, small size, and substantial surface area [ 48 ]. These microbes use biosorption and bioaccumulation to bioremediate. Adsorption occurs when pollutants (metals) interact with functional groups on the cell surface [ 49 ]. Biosorption can use both live and dead biomass. Bioaccumulation involves intracellular and extracellular processes. Toxins bioaccumulate when they are absorbed from the environment. Bioaccumulation uses only living biomass, limiting its reuse, and costing more than biosorption [ 50 ].
4.1. Bacterial removal of organic and inorganic pollutants
The treatment of wastewater effluents is based on the capability of bacterial cells to concentrate pollutants (metals). The microbial population and xenobiotic content determine the rate of biodegradation. Plants feed rhizosphere microbes’ organic carbon, which helps degrade pollutants. Aquatic plants’ biofilms can degrade organics like phenols, amines, and aliphatic aldehydes [ 51 ]. Methanotrophs use methane to obtain carbon and energy and break down various harmful organic compounds [ 52 ]. Eichhornia crassipes can help clean up eutrophic water by influencing nitrogen production [ 53 ]. Tolypothrixceytonica and Anabaena oryzae have also been shown to be effective in treating industrial wastewater [ 54 ]. Aphanocapsu sp . and Plectonema sp . have the ability to degrade crude oil [ 55 ]. Anaerobic bacteria in sewage treatments include sulfate-reducing bacteria like Desulfovibrio, Desulfotomaculum, Desulfobacter, and Desulfococcus genera [ 56 ].
The factors like abundance, size, growth under controlled conditions and resistance to environmental changes have marked bacteria as important biosorbents [ 57 ]. Metal ion biosorption into the cell wall can be active or passive. Passive biosorption occurs in both living and dead/inactive bacterial cells [ 58 ]. Active biosorption includes metal ion uptake within living bacterial cells. Metal ion binding involves ion exchange, chelation, complexation, and micro precipitation [ 59 ].
4.1.1. Dye degradations
Synthetic dyes have many advantages over natural dyes in terms of color variety, speed of coloration, absorption and water solubility [ 60 ], which explains the global dye production of 800,000 tons per year [ 61 ]. The impact of textile effluents on the overall health of the aquatic ecosystem is growing in concern as dye demand and production rise. Textile wastewater contains inorganic and organic additives and chemicals [ 62 ] as well as dyes [ 63 ] in concentrations ranging from 10 to 200 mg/L. In textile industries, the azo dyes (70%) are commonly used because of their low cost and ease of use. Since all dyes do not fix to fabrics during dyeing, unfixed dyes are washed out and found in high concentrations in effluents [ 64 ]. Bacterial-assisted dye degradation is nontoxic and can decolorize colored complex dyes. Table 3 lists some studies on bacterial dye degradation.
Shows the bacterial degradation of dyes and the mechanism involved.
Aeromonas hydrophila, Bacillus subtilis, Bacillus cereus have been studied effectively and have the potential for bioremediation of azo dyes [ 65 ]. Under anoxic conditions, Pseudomonas sp., Pseudomonas luteola, Proteus mirabilis have the ability to degrade azo dyes [ 66 ]. These bacteria utilize oxidoreductive enzymes for dye degradation. Aerobic bacteria use oxygen-catalyzed azoreductase to break the azo bonds [ 67 ]. Some bacterial strains degrade dyes in aerobic conditions and use mono and dioxygenase for oxidizing the aromatic ring of organic compounds [ 68 ]. Anaerobic bacteria use the enzyme azoreductase to degrade azo dyes. And generally, anaerobic conditions favor decolorization (Chang et al., 2001b). Mostly first-order kinetics is followed with respect to the concentration of the dye in the decolorization reaction and in some; zero-order kinetics is also seen [ 69 ]. The oxidoreductive enzymes also are involved in hydroxylation, desulfonation, and deamination. Pseudomonas aeruginosa could decolorize various azo dyes [ 70 ] and Navitan Fast Blue S5R under aerobic conditions.
4.1.2. Petrochemicals degradation
Petroleum hydrocarbons are divided into resins, asphaltenes, aromatics, and saturates [ 79 ]. Their degradation by microbes ( Figure 1 ) is complex, dependent on the nature and number of hydrocarbons available. The biodegradation of hydrocarbons is determined by agents such as temperature and concentration of inorganic nutrients such as phosphorus, nitrogen, and iron in some instances [ 80 ]. The vulnerability of hydrocarbons to attack by microbes is different, with linear alkanes being most susceptible and cyclic alkanes the least [ 81 , 82 ]. Polycyclic hydrocarbons having higher molecular weight might not be degraded [ 83 ]. Acinetobacter sp. degrades n-alkane having chain length C 10 -C 40, utilizing carbon as the sole source [ 84 ]. Mycobacterium , Burkholderia, Gordonia , Brevibacterium , Dietzia, Aeromicrobium, Pseudomonas, Aeromonas, Flavobacteria, Nocardia, Modococci, Chrobacteria, Moraxella, Cyanobacteria, Streptomyces, Bacilli, Arthrobacter , and other bacteria can degrade petroleum products [ 85 ]. The poly-aromatic hydrocarbons could be degraded by Sphingomonas [ 86 ]. The biodegradation efficiency of soil bacteria [ 87 ] and marine bacteria [ 88 ] are not the same. The microbes utilize specific enzymes systems (oxygenase, peroxidase, and hydroxylase) in degrading the petroleum hydrocarbons in aerobic conditions, and it starts with the attachment of microbial cells onto the substrates and is followed by the production of biosurfactants [ 89 , 90 ]. Biosurfactants are synthesized by various microorganisms ( Table 4 ) and are heterogeneous surface-active compounds. Biosurfactants are involved in enhancing the solubility and, finally, the removal of the contaminant [ 91 ]. They augment the surface area and the amount of oil available for the bacteria to utilize [ 92 ] and decrease surface tension to help form micelles.

Microorganism mediated degradation of hydrocarbons.
Biosurfactants produced by the various microbes for degradation of petroleum hydrocarbons.
4.1.3. Pharmaceutical and personal care products
Pharmaceuticals and personal care products (PPCPs) are emerging persistent pollutants [ 93 ]. Pharmaceuticals have increased steadily globally [ 94 ], especially since the COVID-19 pandemic. Individuals contribute PPCPs to the aquatic ecosystem ( Figure 2 ) by using sanitizers, shampoos, household cleaners, detergents, and medicines. PPCPs are complex and persistent molecules that reenter the hydrologic cycle, increasing antibacterial resistance, reproductive abnormalities, and tumor growth. These unregulated pollutants persist in water bodies, and many metabolites are converted back to their parent form [ 95 ]. They precipitate specific pollutants into complex and toxic forms that easily spread in aquatic phases. Figure 2 shows the circulation of PPCP in the surrounding [ 96 ]. The breakdown of PPCPs by microorganisms is difficult because pharmaceuticals were designed to be toxic to bacteria [ 97 ]. Nonetheless, some native bacterial species can help degrade pharmaceutical pollutants [ 98 ]. Microbes reduce or degrade the complex structure to a nontoxic or less toxic form.

Representative diagram of pharmaceuticals and personal care products circulating in the environment adopted from [ 73 ].
4.1.4. Pesticides
Pesticides are chemicals utilized to kill pests and are classified based on their functions (herbicides, algicides, fungicides, bactericides, nematicides, rodenticides, and insecticides) [ 99 ]. Chemical classes of organic pesticides include organophosphorus, organochlorine, carbamates, acetamides, neonicotinoids, pyrethroids, triazoles, and triazines. Inorganic pesticides include lead arsenate, and boric acid complexes, etc. Organochlorine compounds like chlordane, DDT, toxaphene, and heptachlor have been included in the list of persistent organic pollutants [ 100 , 101 ].
It has been reported by many researchers that effectively, less than 5% of total used pesticides are involved in targeting the pests while the rest of them are precipitated in the surrounding water and soil [ 102 ]. The pesticides left in the ecosystem have a detrimental effect on the ecosystem [ 103 ] and need to be removed. The chemical and physical methods of pesticide removal are unsustainable [ 104 ], hence bacteria could function as bio-weapon to fight toxic agricultural chemicals [ 105 ]. Various studies have elaborated on the role of bacteria in bioremediating pesticides, viz. Endosulfan removal by Bacillus and Staphylococcus [ 106 ]; Malathion removal by Arthrobacter sp ., Pseudomonas putida [ 107 ]; Ridomil and Fitoraz removal by Pseudomonas putida and Acinetobacter sp . [ 108 ]; Napthelene removal by Cyanobacteria [ 109 ]; Endosulfan removal by Staphylococcus aureus , Achromobacter sp ., Rhodococcus sp . [ 110 ]; Malathion, Ridomil and Fitoraz removal by Pseudomonas putida , Rhodococcus and Arhtrobacter sp . [ 111 ].
4.1.5. Heavy metals degradation
Heavy metals such as lead (Pb), cadmium (Cd), chromium (Cr), arsenic (As), and mercury (Hg) are ubiquitous environmental pollutants, having high toxicity and density. Natural and anthropogenic sources of heavy metal pollution cause detrimental effects on all living beings [ 112 ]. The microbial cells require cations for numerous cellular activities, but increasing concentration may retard growth by forming internal complexes [ 113 ]. Bacteria have the ability to immobilize and also mobilize, transform and uptake heavy metals [ 114 ]. Many studies have been published on the role of endophytic bacteria in heavy metal bioaccumulation and detoxification [ 115 , 116 ]. These studies show that bacteria secrete organic acids to help with the bioremediation process. Bacteria also produce biosurfactants released as root exudates and increase metal bioavailability in aquatic environments [ 117 ]. It was found that glutathione was involved in the intracellular sequestration of cadmium ions in the cells of Rhizobium leguminosarum [ 118 ]. Heavy metals can be reduced to less or nontoxic metals by iron-reducing bacteria like Geobacter sp. and sulfur-reducing bacteria like Desufuromonas sp. Sulfate-reducing bacteria and metal-reducing bacteria, for example, can convert chromium from the highly toxic Cr (VI) to the less toxic Cr (III) [ 119 ]. Sulfate-reducing bacteria produce a lot of hydrogen sulfide, which causes metal cations to precipitate [ 120 ]. Vibrio harveyi strain could precipitate the divalent lead as a salt of lead phosphate [ 121 ]. Many ionizable cell wall groups can help bacteria absorb metal ions (amino, carboxyl, phosphate, and hydroxyl gp). In metal remediation, microbial methylation is also important. For example, Bacillus sp., Clostridium sp., Pseudomonas sp., and Escherichia sp., can biomethylate Hg (II) [ 122 ]. Various heavy metals respond to the microorganism differently depending on the conditions. Some bacterial cells produce siderophores, and they form metal complexes, limiting their bioavailability and removing their toxicity [ 123 ]. Some of the bacterial species involved in the bioremediation of heavy metals ( Table 5 ) have been tabulated.
Some Bacterial species used for the removal of heavy metals.
4.2. Fungi and yeast
Fungi can help in the removal of pollutants (heavy metals) by increasing their bioavailability and converting them to lesser toxic forms [ 124 ]. Fungi are simple to grow and produce a significant amount of biomass. Several fungal strains have shown the ability to digest a variety of environmental contaminants, including dyes, pharmaceutical drugs, aromatic hydrocarbons, and heavy metals [ 125 , 126 ]. The two important characteristics of fungi that make them an ideal candidate for wastewater treatment are the secretion of many extracellular enzymes [ 127 ] and the hyphal mesh of fungi that protects the internal sensitive organelles from the ill effects of contaminants. Fungi are drawn to the rhizosphere by root exudates. Many factors influence plant-fungi interactions in the rhizosphere, including soil characteristics, plant species, water type, climate, and other microorganisms [ 128 ]. Plant-fungi interactions perform a variety of important functions, including metal-chelating siderophores emission, denitrification, and detoxification ( Figure 3 ). The organic wastes are transformed into industrially important biochemicals and other valuable compounds by fungi, which is an advantage of using fungal culture in wastewater treatment over bacterial culture (proteins, organic acids). Animal feed can also be made from fungal biomass [ 129 ]. Pleurotus pulmonarius, Stachybotrys sp., Cephalosporium aphidicola, Aspergillus parasitica, Verticillum terrestre, Candida sp., Acremonium sp., Glomus sp., Minimedusa sp., Talaromyces, Hydnobolites, Peziza , and other fungal species can be used in wastewater treatment [ 130 ]. Table 6 shows the effective fungal degradation of wastewater.

A schematic diagram of wastewater treatment by microorganisms with their different bioremediation mechanisms.
Some common fungal species used in pollutant remediation in wastewater.
Over the last years, many research studies have suggested the effective role of ligninolytic fungi in degrading synthetic dyes [ 131 ]. Interestingly, fungi possess ligninolytic enzymes that degrade complex dyes, including laccase, manganese peroxidase, and lignin peroxidase. Some research studies reflecting fungi’s role in degrading dyes have been elucidated in Table 7 .
Shows the degradation of some dyes by fungi along with the mechanism involved.
Many studies have shown that yeast can be used to eliminate pollutants (heavy metals) from the environment [ 132 ]. Yeast can also help reduce COD levels and remove mono and polyphenols [ 133 ] because yeast can absorb, accumulate, and degrade toxic compounds into nontoxic forms. It can be used to treat textile wastewater. Saccharomyces cerevisiae, Galactomyces geotrichum , Trichosporon beigelii , and Candida krusei can degrade dyes in wastewater [ 134 ].
4.3. Microalgae
These include the use of eukaryotic algae and cyanobacteria for biological wastewater treatment [ 135 ]. The term ‘phycoremediation’ refers to the use of algal species for bioremediation. Chlorella sp., Picochlorum sp., Tetraselmis sp., Scenedesmus sp., and other algal and cyanobacterial strains like Anabaena sp., Oscillatoria sp., Spirulina sp., Chroococcus sp., Pseudospongiococcus sp., Scytonema sp., Dolichospermum [ 136 ] are used in wastewater treatment. Microalgae have the following characteristics that make them an ideal candidate for wastewater treatment:
- Capability to utilize both inorganic and organic carbon, nitrogen, and phosphorus present in wastewater for growth [ 137 ].
- The life cycle is short and requires less nutrients [ 164 ].
- Scope of re-using algal biomass through adsorption/desorption mechanism [ 165 ].
- The growth of algal biomass is independent of environmental conditions, hence can be produced throughout the year [ 166 ].
- The efficiency of algal biomass is better than membranes to remove heavy metals [ 167 ].
- Source of Oxygen and helps in degradation process by heterotrophic bacteria [ 168 ].
- Useful in both anaerobic and aerobic effluent treatment plants [ 169 ].
Depending on the nutrient source, capital investment, and culture conditions (biofuels, CO 2 capture), microalgae culture-based wastewater treatments can be open or closed.
4.3.1. Open type
Algae are grown in open systems in places like ponds, lagoons, and deep channels. Natural (ponds, lagoons) or artificial (man-made ponds, tanks, containers) sites can be used. For domestic and industrial wastewater treatment, stabilization ponds containing bacteria and microalgae culture are most commonly used in temperate and tropical climates. Many studies have demonstrated the effective use of open microalgal cultured treatment plants in treating wastewater [ 170 ].
4.3.2. Closed systems
Microalgae is grown in closed environments in such systems. Photobioreactors are one example of such a system. Reduced water evaporation, higher biomass yield, and contamination elimination are all advantages of closed-type treatment over open type [ 171 ]. A pilot-scale tubular bioreactors are used to grow a diverse range of microalgae, including Arthrospira sp., Chlorella sp., Haematococcus sp., Spirulina and Phaeodactylum sp [ 172 ].
Algal biosorbents have a high sorption capacity [ 173 ]. Using algae-based biosorption, heavy metal ion extraction from wastewater could be an environmentally friendly, cost-effective, and efficient method [ 174 ]. Textile wastewater contains algae cultivation nutrients (phosphates, nitrates, micronutrients, etc.) as well as organic dyes [ 175 ]. Many studies ( Table 8 ) have shown that microalgae can remove pollutants from wastewater; for example, C. vulgaris and S. quadricauda can remove nitrate [ 190 ]; Chlorella sp., Scenedesmus sp., Cosmarium sp. for wastewater treatment [ 191 ]; Chlorella sp., Scenedesmus sp., Cosmarium sp. for treatment of wastewater (aquaculture wastewater and textile wastewater [ 192 ]. According to Ojha et al. [ 207 ], C. vulgaris and S. quadricauda cultures can be used for wastewater remediation.
Some microalgae species used in wastewater treatment.
Organic dyes are major pollutants in water. They can be found in many manufacturing industries, including textiles, plastics, and medicines. These dyes, when accumulated in aquatic systems, results into eutrophication and limited reoxygenation capacity. The production of poisonous amines during the decomposition of dyes is one of the most serious concerns [ 208 ]. Microalgae and Cyanobacteria represent a possible option for the bioremediation of wastewater. Microalgae decolorize dyes by adsorption or degradation. Microalgae can make use of wastewater dyes and nutrients. During the bioconversion process, microalgae can consume the dyes as a source of carbon and convert them to metabolites. The degradation of dyes by microalgae has been elucidated through some research studies in ( Table 9 ).
Shows the degradation of some dyes by microalgae along with the mechanism involved.
5. Factors affecting microbial biodegradation
Microbes can degrade various physical and chemical wastes through removal, alteration, immobilization, or detoxification. Microbes play a role because of their enzymatic pathways. Many factors influence bioremediation efficiency, including soil type, temperature, pH, oxygen, and other electron acceptors, nutrients, biological factors, and so on.
5.1. Environmental determinants
Temperature is the most important of all the physical factors that influence microorganism survival [ 209 ]. Microbial enzymes involved in biodegradation require the right temperature to metabolize substances. The rate of microbial activity increases as the temperature rises and peaks at the optimum temperature. The temperature of water influences various processes such as mineralization, diffusion, and chemical reactions [ 210 ]). Temperature extremes can kill bacteria and other microbes, affecting their growth [ 211 ]. Increases in temperature within the optimum range raise the reaction temperature, thereby increasing the solubility of contaminants, improving diffusion, and so on. The bacterial consortium of Bacillus pumilus HKG212 and Zobellella taiwanensis AT was used by [ 212 ] to degrade reactive green 19, and their findings revealed that the highest degradation occurs at 32.04°C.
The measurement of pH indicates microbial growth potential [ 224 ]. The pH range determines the survival of bacterial species, and thus bioremediation. Acidophilic, neutrophilic, and alkaliphilic biodegrading bacteria require acidic, neutral, and basic media for optimal activity [ 225 ]. According to [ 226 ], the pH of the affected site can be changed to achieve the desired biodegradation results. At pH 4.5, they were able to degrade malachite green by 98% using RuO 2 -TiO 2, and Pt coated Ti mesh electrodes.
Moisture has an impact on the rate of biodegradation because moisture affects the content and concentration of soluble materials available, as well as the osmotic pressure and pH of aquatic systems.
Different microbes require different oxygen levels, such as aerobic, anaerobic, and semi-anaerobic conditions. In most cases, the presence of oxygen can help with hydrocarbon metabolism. Some contaminants, such as petroleum hydrocarbons in wastewater, inhibit bacterial growth by reducing compressed oxygen and electron acceptors [ 227 ]. Although shaking can improve oxygenation, delivering enough oxygen for the biodegradation of organic pollutants is a part of an operational issue and is costly [ 228 ].
An optimum quantity of nutrients and other chemicals is important for microbial metabolism [ 229 ]). Additional input of nutrients changes the nutrient balance for microbial growth, affecting the rate and effectiveness of biodegradation [ 230 ]. Microbes require various nutrients, including carbon, nitrogen, and phosphorus, to survive and continue their metabolic activities [ 231 ]. Varjani et al. [ 232 ] identified phosphorus as a critical factor in microbe growth.
5.1.1. Contaminant concentration
The type and number of contaminants can have an impact on biodegradation. A high biodegradation rate can be achieved by increasing the contaminant concentration [ 233 ]. Heavy contaminants, such as oil petroleum-containing wastewater, have been fatal to the microbial community and negatively impact their biocatalytic activity. Low molecular weight contaminants with simple structures can achieve a high bioremediation rate [ 234 ]. Kerosene, for example, can be completely biodegraded at optimal concentrations due to its simple structure and low molecular weight [ 235 ].
5.1.2. Salinity
According to [ 236 ] organic pollutants present in wastewater which contain alkaline chemicals biodegrade very slowly due to their ability to persist in waste. Contaminants with a high salt content may reduce biodegradation activity by inhibiting biological movement [ 237 ].
5.2. Biological factors
Biological factors influence the breakdown of organic pollutants as microorganisms compete for limited carbon sources, and antagonistic interactions between microbes exist. Major biological factors that affect the bioremediation activity of microbes include enzyme activity, interactions (competition, succession, and predation), population size and composition, mutation, etc. [ 238 ]. The rate of biodegradation is dependent on the substrate as well as the biocatalyst [ 239 ] and the specificity of the enzyme. Inhibition of enzymatic activities due to several factors like competition for carbon and nutrient sources can affect the biodegradative activity of microbes [ 240 ].
6. Microbial consortium: emerging technology
The microbial consortium is the emerging biotechnology-based green approach. Using a single microbe strain to treat wastewater may not give effective results, and efficiency can be compromised. Thus, many research findings have proposed applying microbial consortia [ 241 , 242 ]. Consortia comprising different groups of environmental microbes capable of degrading pollutants in wastewater can be an effective choice. Such consortiums have many advantages over the application of a single strain like fast removal, assistance in secondary application of treated wastewater, along with promoting ecological sustainability.
In the natural habitat, biofilm is formed by aggregating different groups of microbes attached through exopolymeric substances. The whole system is synergistic with microbial partners’ contributing toward forming a strong community [ 243 ].
The development of consortia is an emerging approach for wastewater treatment. The algal-bacterial consortium has many advantages owing to its biomass refiniability and reduced power consumption [ 244 ]. The fundamental principle in the microbial community is utilizing beneficial relationships which are promoting in pollutant removal from wastewater. The synergy is observed in the relationship wherein bacteria are involved in BOD removal, and algae remove nitrogen and phosphorus by absorption [ 245 ]. The relationship established between algae and bacteria provides a suitable ground for bioremediation [ 246 ]. Photosynthesis is undertaken by cyanobacteria bacteria and converts inorganic carbon present in wastewater to organic carbon [ 247 ]. The CO 2 produced by bacterial oxidation serves as the carbon source for photosynthetic algae. Decomposers like Acinetobacter can remove BOD and oxidize organic carbon sources into CO 2, which serves in algae growth [ 248 ].
Extensive research findings have supported microbial consortium as a potential candidate for wastewater treatment [ 249 ]. Recent experimental studies conducted by [ 250 ] revealed the application of Ecobacter bacterial consortium facilitated the bioaugmentation for the biological removal of nitrogen compounds; showing ammonium was transformed by the microorganism reduction reaction; thus, presented decrease in the concentration of ammonium at the end of the treatment period. In their studies, Qi et al. [ 251 ] proved effectively that a well-established microbial (algal-bacterial) consortium in the phycosphere can be optimized and used in advanced wastewater treatment. The results of the research conducted by [ 252 ] showed that treatment of paper pulp wastewater by microbial consortium between microalgae and bacteria allowed good efficiency in removal of organic matter and nutrients. Rehman et al. [ 253 ] studied microbial consortium with Klebsiella sp. LCR187, Bacillus subtilis LOR166, Acinetobacter sp. BRS156 and Acinetobacter junii TYRH47 and Typhadomingensis and Leptochloafusca to treat oil field wastewater. Tara et al. [ 254 ] reported greater than 90% removal efficiency of pollutants from textile wastewater using microbial consortium. Leong et al. [ 255 ] reported 94% pollutant removal efficiency from municipal wastewater using microalgae consortium with bacteria. Microbes carry out the degradation through the secretion of various enzymes and organic acids [ 256 ]. Monica et al. [ 257 ] used Effective Microorganism (EM), which comprises Lactobacillus , Aspergillus, Pseudomonas , Streptomyces , and Saccharomyces , for biodegradation of sewage load in the water. Lactobacillus does the breakdown of lignin and cellulose in this consortium. Pseudomonas releases bioactive compounds which act on sewage and detoxifies or precipitate the metal. Aspergillus decomposes organic matter rapidly, producing alcohol and esters. Table 10 shows the effective utilization of consortia between microbes for treating wastewater from various sources.
Microbial consortium for treatment of wastewater.
7. Conclusion
Water contamination from various sources has become a serious problem around the world. The use of microbes as a treatment for water pollution is a viable alternative. Microbial remediation is an evolutionary and revolutionary technique for wastewater treatment that is currently in use. Microbes (bacteria, fungi, algae, and yeast) are naturally occurring and thus offer a long-term solution to the problem of water pollution. Lack of appropriate information about microbes’ metabolic capacity to degrade contaminants and a lack of controlled conditions such as temperature, pH, the appropriate number of contaminants, nutrients, and more time consumption are all possible limitations with the use of microbes in the treatment process. If the process is not controlled, contaminants may not be completely degraded, resulting in toxic byproducts. As a result, appropriate inside characterization can be an effective way to overcome the drawbacks of microbial-assisted wastewater remediation. Success in microbial wastewater treatment can also be attributed to advances in genetic engineering. Engineered microbial strains with high metabolic potential and well-understood detoxification pathways will undoubtedly aid in combating the wastewater threat to the greatest extent possible. Although several studies have been performed on the use of microbial consortium like microalgae-bacterial systems for the treatment of waste water, but still there is a need for further research in optimizing parameters for large-scale units. Maintaining the stability of the consortium is the main challenging task. More emphasis should be placed on some parameters viz. on selection of capable microbial strains, modeling the system in the long run and optimizing operational parameters, techno economic feasibility, etc. The present era demands to develop environmentally friendly technology that is also commercially viable. Engineered microbes must be integrated from the scientific stage to the practical and pilot stage in order to make significant advances in the use of microbes in the wastewater treatment process. Effective coordination across various disciplines and updated technologies are required to develop better environmental management in the near future.
Acknowledgments
Authors acknowledge to Department of chemical engineering IIT (BHU) Varanasi and PGDAV Delhi University for providing facilities.
Funding Statement
The author(s) reported that there is no funding associated with the work featured in this article.
Disclosure statement
No potential conflict of interest was reported by the authors.
wastewater treatment Recently Published Documents
Total documents.
- Latest Documents
- Most Cited Documents
- Contributed Authors
- Related Sources
- Related Keywords
Metagenomic insights into the profile of antibiotic resistomes in sediments of aquaculture wastewater treatment system
Corrigendum to ’beyond treatment technology: understanding motivations and barriers for wastewater treatment and reuse in unconventional energy production’, improving the efficiency of wastewater treatment and microalgae production for biofuels, hybrid zeolite-based ion-exchange and sulfur oxidizing denitrification for advanced slaughterhouse wastewater treatment, advanced wastewater treatment process using algal photo-bioreactor associated with dissolved-air flotation system: a pilot-scale demonstration, cometabolic biodegradation of antibiotics by ammonia oxidizing microorganisms during wastewater treatment processes, synergistic effects of oxidation, coagulation and adsorption in the integrated fenton-based process for wastewater treatment: a review, fate evaluation of pharmaceuticals in solid and liquid phases at biological process of full-scale municipal wastewater treatment plants, impact of different inoculum sources on the performance of membrane bioreactors for municipal wastewater treatment: dynamic membrane versus ultrafiltration membrane, enhancement of wastewater treatment performance using 3d printed structures: a major focus on material composition, performance, challenges, and sustainable assessment, export citation format, share document.

Switch to green wastewater infrastructure could reduce emissions and provide huge savings according to new research
University researchers have shown that a transition to green wastewater-treatment approaches in the U.S. that leverages the potential of carbon-financing could save a staggering $15.6 billion and just under 30 million tonnes of CO 2 -equivalent emissions over 40 years.
The comprehensive findings from Colorado State University were highlighted in Nature Communications Earth and Environment in a first-of-its-kind study. The work from the Walter Scott, Jr. College of Engineering explores the potential economic tradeoffs of switching to green infrastructure and technology solutions that go beyond existing grey-water treatment practices. Built off data collected at over 22,000 facilities, the report provides comprehensive baseline metrics and explores the relationship among emissions, costs and treatment capabilities for utility operators and decision makers.
Braden Limb is the first author on the paper and a Ph.D. student in the Department of Systems Engineering. He also serves as a research associate in the Department of Mechanical Engineering. He said the findings are a key initial step to categorize and understand potential green solutions for wastewater.
"These findings draw a line in the sand that shows what the potential for adopting green approaches in this space is -- both in terms of money saved and total emissions reduced," he said. "It is a starting point to understand what routes are available to us now and how financing strategies can elevate water treatment from a somewhat local issue into something that is addressed globally through market incentives."
The research was completed in partnership with the University of Colorado Boulder and Brigham Young University. Findings center around both point-source water treatment and non-point sources of water pollution.
Traditional point-source water treatment facilities such as sewage plants remove problem nutrients like nitrogen and phosphorus before releasing water back into circulation. This grey-infrastructure system -- as it is known -- is monitored by the Environmental Protection Agency. However, regulation standards may tighten in the future, and facilities would need more power, and in turn more emissions, to reach newly allowable thresholds. Existing facilities already account for 2% of all energy use in the U.S. and 45 million tonnes of CO 2 emissions, said Limb.
Another significant source of freshwater contamination in the U.S. comes from non-point source activity such as fertilizer runoff from agriculture entering rivers. Other non-point sources of pollution can come from wildfires -- aided by climate change -- or urban development, for example.
Limb said that rather than building more grey-infrastructure treatment facilities to address those increasing sources, the paper explores green approaches financed through carbon markets that can tackle both types simultaneously.
"There could be a switch to nature-based solutions such as constructing wetlands or reforestation instead of building yet another treatment facility," he said. "Those options could sequester over 4.2 million carbon dioxide emissions per year over a 40-year time horizon and have other complementary benefits we should be aiming for, such as cheaper overall costs."
Carbon financing is a mechanism aimed at mitigating climate change by incentivizing activities that reduce emissions or sequester them from the atmosphere. Companies voluntarily buy "credits" on an open market that represent a reduction or removal of carbon from the atmosphere that can be accomplished in a variety of ways. That credit offsets the institution's emissions from operations as it aims to reach sustainability goals.
These trades incentivize development of sustainable activities and can also provide a source of fresh money to further develop or scale up new approaches.
While there are similar financing markets for water, the problem is initially more localized than it is for air quality and carbon. That dynamic has limited the value of water market trades in the past. The paper suggests that these existing markets could be improved, and that the carbon markets could also be leveraged to change some of the financial incentives farmers have around water treatment and impacts from their activity.
The researchers found that using the markets could generate $679 million annually in revenue, representing an opportunity to further motivate green infrastructure solutions within water quality trading programs to meet regulated standards.
Mechanical Engineering Professor Jason Quinn is a co-author on the study. He said the findings have some limitations, but that this was an important first step to model both the problem and opportunity available now. He said the results in the paper have supported new research at CSU with the National Science Foundation to further develop the needed carbon credit methodology with stakeholders.
"This is the first time we are considering air and water quality simultaneously -- water is local and carbon is global," he said. "But by bringing these market mechanisms together we can capitalize on a window of opportunity to accelerate the improvement of America's rivers as we transition to a renewable energy and restored watershed future."
- Nature of Water
- Energy Policy
- Energy Technology
- Engineering
- Sustainability
- Environmental Issues
- Environmental Science
- Carbon dioxide sink
- Geologic temperature record
- Climate engineering
- Carbon dioxide
- Commercial fishing
- Automobile emissions control
Story Source:
Materials provided by Colorado State University . Original written by Josh Rhoten. Note: Content may be edited for style and length.
Journal Reference :
- Braden J. Limb, Jason C. Quinn, Alex Johnson, Robert B. Sowby, Evan Thomas. The potential of carbon markets to accelerate green infrastructure based water quality trading . Communications Earth & Environment , 2024; 5 (1) DOI: 10.1038/s43247-024-01359-x
Cite This Page :
Explore More
- Coffee's Prehistoric Origin and It's Future
- Can Animals Count? New Rat Study
- A Single Atom Layer of Gold: Goldene
- Fool's Gold May Contain Valuable Lithium
- Exercise Cuts Stress-Related Brain Activity
- Microplastics Go from the Gut to Other Organs
- Epilepsy Drug May Prevent Brain Tumors
- Evolution's Recipe Book
- Green Wastewater-Treatment: Huge CO2 Cut
- Tropical Forests Need Fruit-Eating Birds
Trending Topics
Strange & offbeat.
Source analysis of chlorine in municipal solid waste under waste classification: a case study of Hangzhou, China
- Research Article
- Published: 16 April 2024
Cite this article
- Yuyang Long 1 ,
- Ying Hu 1 ,
- Dongyun Liu 1 ,
- Dongsheng Shen 1 &
- Foquan Gu 1
Inorganic chlorine is susceptible to water and soil salinization due to its non-degradability and high mobility. To clarify the environmental risks associated with the active inorganic chlorine in municipal solid waste (MSW), the specific characteristics and contributions of inorganic chlorine in different MSW categories were investigated in this study. MSW samples were collected from eight representative waste classification residential areas in Hangzhou, China. It was found that the inorganic chlorine content in different MSW categories varied significantly (0–113 mg/g). Perishable waste, paper, and plastic were found to be the main sources of inorganic chlorine in MSW. A four-category classification system was used to quantify the contribution of inorganic chlorine from each waste category. It was found that the misclassification of inorganic chlorine contributions from perishable waste and other waste accounted for 51.96% and 48.04%, respectively. However, when correctly classified into the four-category system, their contributions were reduced to 67.14% and 30.65%, respectively. Therefore, MSW classification showed a significant reduction in the overall contribution of inorganic chlorine. The misclassification reduces the contribution of inorganic chlorine to 48.04%, while correct classification increases the reduction to 69.35%.
This is a preview of subscription content, log in via an institution to check access.
Access this article
Price includes VAT (Russian Federation)
Instant access to the full article PDF.
Rent this article via DeepDyve
Institutional subscriptions
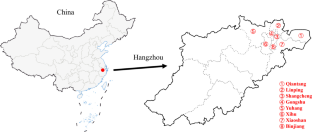
Bian R, Chen J, Zhang T, Gao C, Niu Y, Sun Y, Zhan M, Zhao F, Zhang G (2022) Influence of the classification of municipal solid wastes on the reduction of greenhouse gas emissions: a case study of Qingdao City, China. J Clean Prod 376:134275. https://doi.org/10.1016/j.jclepro.2022.134275
Article CAS Google Scholar
Cai Z, Du B, Dai X, Wang T, Wang J, Zhang Y (2023) Coupling of alkaline and mechanical modified fly ash for HCl and SO 2 removal in the municipal solid waste incineration plant. Fuel 346:128354. https://doi.org/10.1016/j.fuel.2023.128354
Cheng S, Ding X, Dong X, Zhang M, Tian X, Liu Y, Huang Y, Jin B (2023) Immigration, transformation, and emission control of sulfur and nitrogen during gasification of MSW: fundamental and engineering review. Carbon Resour Convers 6:184–204. https://doi.org/10.1016/j.crcon.2023.03.003
Deng Y, Chen N, Feng C, Chen F, Wang H, Kuang P, Feng Z, Liu T, Gao Y, Hu W (2019) Treatment of organic wastewater containing nitrogen and chlorine by combinatorial electrochemical system: taking biologically treated landfill leachate treatment as an example. Chem Eng J 364:349–360. https://doi.org/10.1016/j.cej.2019.01.176
Gu B, Jiang S, Wang H, Wang Z, Jia R, Yang J, He S, Cheng R (2017) Characterization, quantification and management of China’s municipal solid waste in spatiotemporal distributions: a review. Waste Manag 61:67–77. https://doi.org/10.1016/j.wasman.2016.11.039
Article Google Scholar
Honma S, Hu J (2021) Cost efficiency of recycling and waste disposal in Japan. J Clean Prod 284:125274. https://doi.org/10.1016/j.jclepro.2020.125274
Kassman H, Pettersson J, Steenari BM, Åmand LE (2013) Two strategies to reduce gaseous KCl and chlorine in deposits during biomass combustion — injection of ammonium sulphate and co-combustion with peat. Fuel Process Technol 105:170–180. https://doi.org/10.1016/j.fuproc.2011.06.025
Kruta de Araujo NC, Sato T (2018) A descriptive study of work ability and health problems among Brazilian recyclable waste pickers. J Commun Health 43:366–371. https://doi.org/10.1007/s10900-017-0432-6
Li H, Liu H, Huang Y, Chen X, Zhang X, Li J, Xu L, Yao H (2024a) Mechanism of coupling corrosion caused by flue gas and deposits in municipal solid waste incinerator: roles of H 2 O(g), HCl, and SO 2 . Corros Sci 231:111933. https://doi.org/10.1016/j.corsci.2024.111933
Li S, Zhang M, Hu H, Guo G, Gong L, Dong L, Xu S, Yao H (2024b) Fate of sulfur and chlorine during co-incineration of municipal solid waste and industrial organic solid waste. Sci Total Environ 920:171040. https://doi.org/10.1016/j.scitotenv.2024.171040
Liu Y, Wang J (2023) Spatiotemporal patterns and drivers of carbon emissions from municipal solid waste treatment in China. Waste Manag 168:1–13. https://doi.org/10.1016/j.wasman.2023.05.043
Liu Y, Xing P, Liu J (2017) Environmental performance evaluation of different municipal solid waste management scenarios in China. Resour Conserv Recycl 125:98–106. https://doi.org/10.1016/j.resconrec.2017.06.005
Liu B, Han Z, Liang X (2023) Dioxin emissions from municipal solid waste incineration in the context of waste classification policy. Atmos Pollut Res 14:101842. https://doi.org/10.1016/j.apr.2023.10184
Long Y, Shen D, Wang H, Lu W, Zhao Y (2011) Heavy metal source analysis in municipal solid waste (MSW): case study on Cu and Zn. J Hazard Mater 186:1082–1087. https://doi.org/10.1016/j.jhazmat.2010.11.106
Long Y, Liu D, Xu J, Fang Y, Du Y, Shen D (2018) Release behavior of chloride from MSW landfill simulation reactors with different operation modes. Waste Manag 77:350–355. https://doi.org/10.1016/j.wasman.2018.04.018
Lv L, Sun P, Gu Z, Du H, Pang X, Tao X, Xu R, Xu L (2009) Removal of chloride ion from aqueous solution by ZnAl-NO 3 layered double hydroxides as anion-exchanger. J Hazard Mater 161:1444–1449. https://doi.org/10.1016/j.jhazmat.2008.04.114
Lv J, Dong H, Geng Y, Li H (2020) Optimization of recyclable MSW recycling network: a Chinese case of Shanghai. Waste Manag 102:763–772. https://doi.org/10.1016/j.wasman.2019.11.041
Ma W, Wenga T, Frandsen FJ, Yan B, Chen G (2020) The fate of chlorine during MSW incineration: vaporization, transformation, deposition, corrosion and remedies. Prog Energy Combust Sci 76:100789. https://doi.org/10.1016/j.pecs.2019.100789
McSorley K, Rutter A, Cumming R, Zeeb BA (2016) Phytoextraction of chloride from a cement kiln dust (CKD) contaminated landfill with Phragmites australis. Waste Manag 51:111–118. https://doi.org/10.1016/j.wasman.2015.11.009
Shan L, Tian S, Hu Q (2016) Effect of deicing chloride salts on roadside plants and soil. Saf Environ Eng 23:89–95. https://doi.org/10.13578/j.cnki.issn.1671-1556.2016.03.015
Song Y, Xian X, Zhang C, Zhu F, Yu B, Liu J (2023) Residual municipal solid waste to energy under carbon neutrality: challenges and perspectives for China. Resour Conserv Recycl 198:107177. https://doi.org/10.1016/j.resconrec.2023.107177
Tai J, Zhang W, Che Y, Feng D (2011) Municipal solid waste source-separated collection in China: a comparative analysis. Waste Manag 31:1673–1682. https://doi.org/10.1016/j.wasman.2011.03.014
Tang L, Guo J, Wan R, Jia M, Qu J, Li L, Bo X (2023) Air pollutant emissions and reduction potentials from municipal solid waste incineration in China. Environ Pollut 319:121021. https://doi.org/10.1016/j.envpol.2023.121021
Teakle NL, Tyerman SD (2010) Mechanisms of Cl-transport contributing to salt tolerance. Plant Cell Environ 33:566–589. https://doi.org/10.1111/j.1365-3040.2009.02060.x
Vehlow J (1996) Municipal solid waste management in Germany. Waste Manag 16:367–374. https://doi.org/10.1016/S0956-053X(96)00081-5
Yang N, Zhang H, Chen M, Shao L, He P (2012) Greenhouse gas emissions from MSW incineration in China: impacts of waste characteristics and energy recovery. Waste Manag 32:2552–2560. https://doi.org/10.1016/j.wasman.2012.06.008
Zhao F, Bian R, Zhang T, Fang X, Chai X, Xin M, Li W, Sun Y, Yuan L, Chen J, Lin X, Liu L (2022) Characteristics of polychlorinated dibenzodioxins/dibenzofurans from a full-scale municipal solid waste (MSW) incinerator in China by MSW classification. Process Saf Environ 161:50–57. https://doi.org/10.1016/j.psep.2022.03.012
Zhou H, Long Y, Meng A, Li Q, Zhang Y (2015) Thermogravimetric characteristics of typical municipal solid waste fractions during co-pyrolysis. Waste Manag 38:194–200. https://doi.org/10.1016/j.wasman.2014.09.027
Download references
This work was partially supported by the Scientific Research Project of Zhejiang Provincial Department of Education (Y202147303).
Author information
Authors and affiliations.
School of Environmental Science and Engineering, Zhejiang Provincial Key Laboratory of Solid Waste Treatment and Recycling, Zhejiang Engineering Research Center of Non-Ferrous Metal Waste Recycling, Zhejiang Gongshang University, Hangzhou, 310012, Zhejiang, China
Yuyang Long, Ying Hu, Dongyun Liu, Dongsheng Shen & Foquan Gu
You can also search for this author in PubMed Google Scholar
Contributions
Yuyang Long: project administration, supervision, writing—review and editing; Ying Hu: investigation, data curation, visualization; Dongyun Liu: data curation, visualization; Dongsheng Shen: supervision, resources; Foquan Gu: writing—original draft, writing—review and editing, formal analysis, validation.
Corresponding author
Correspondence to Foquan Gu .
Ethics declarations
Ethics approval.
Not applicable.
Consent to participate
Consent for publication, conflict of interest.
The authors declare no competing interests.
Additional information
Responsible Editor: Xianliang Yi
Publisher's Note
Springer Nature remains neutral with regard to jurisdictional claims in published maps and institutional affiliations.
Rights and permissions
Springer Nature or its licensor (e.g. a society or other partner) holds exclusive rights to this article under a publishing agreement with the author(s) or other rightsholder(s); author self-archiving of the accepted manuscript version of this article is solely governed by the terms of such publishing agreement and applicable law.
Reprints and permissions
About this article
Long, Y., Hu, Y., Liu, D. et al. Source analysis of chlorine in municipal solid waste under waste classification: a case study of Hangzhou, China. Environ Sci Pollut Res (2024). https://doi.org/10.1007/s11356-024-33243-8
Download citation
Received : 29 September 2023
Accepted : 03 April 2024
Published : 16 April 2024
DOI : https://doi.org/10.1007/s11356-024-33243-8
Share this article
Anyone you share the following link with will be able to read this content:
Sorry, a shareable link is not currently available for this article.
Provided by the Springer Nature SharedIt content-sharing initiative
- Classification
- Find a journal
- Publish with us
- Track your research
Francis Collins: Why I’m going public with my prostate cancer diagnosis
I served medical research. now it’s serving me. and i don’t want to waste time..
Over my 40 years as a physician-scientist, I’ve had the privilege of advising many patients facing serious medical diagnoses. I’ve seen them go through the excruciating experience of waiting for the results of a critical blood test, biopsy or scan that could dramatically affect their future hopes and dreams.
But this time, I was the one lying in the PET scanner as it searched for possible evidence of spread of my aggressive prostate cancer . I spent those 30 minutes in quiet prayer. If that cancer had already spread to my lymph nodes, bones, lungs or brain, it could still be treated — but it would no longer be curable.
Why am I going public about this cancer that many men are uncomfortable talking about? Because I want to lift the veil and share lifesaving information, and I want all men to benefit from the medical research to which I’ve devoted my career and that is now guiding my care.
Five years before that fateful PET scan, my doctor had noted a slow rise in my PSA, the blood test for prostate-specific antigen. To contribute to knowledge and receive expert care, I enrolled in a clinical trial at the National Institutes of Health, the agency I led from 2009 through late 2021.
At first, there wasn’t much to worry about — targeted biopsies identified a slow-growing grade of prostate cancer that doesn’t require treatment and can be tracked via regular checkups, referred to as “active surveillance.” This initial diagnosis was not particularly surprising. Prostate cancer is the most commonly diagnosed cancer in men in the United States, and about 40 percent of men over age 65 — I’m 73 — have low-grade prostate cancer . Many of them never know it, and very few of them develop advanced disease.
Why am I going public about this cancer that many men are uncomfortable talking about? Because I want to lift the veil and share lifesaving information.
But in my case, things took a turn about a month ago when my PSA rose sharply to 22 — normal at my age is less than 5. An MRI scan showed that the tumor had significantly enlarged and might have even breached the capsule that surrounds the prostate, posing a significant risk that the cancer cells might have spread to other parts of the body.
New biopsies taken from the mass showed transformation into a much more aggressive cancer. When I heard the diagnosis was now a 9 on a cancer-grading scale that goes only to 10, I knew that everything had changed.
Thus, that PET scan, which was ordered to determine if the cancer had spread beyond the prostate, carried high significance. Would a cure still be possible, or would it be time to get my affairs in order? A few hours later, when my doctors showed me the scan results, I felt a rush of profound relief and gratitude. There was no detectable evidence of cancer outside of the primary tumor.
Later this month, I will undergo a radical prostatectomy — a procedure that will remove my entire prostate gland. This will be part of the same NIH research protocol — I want as much information as possible to be learned from my case, to help others in the future.
While there are no guarantees, my doctors believe I have a high likelihood of being cured by the surgery.
My situation is far better than my father’s when he was diagnosed with prostate cancer four decades ago. He was about the same age that I am now, but it wasn’t possible back then to assess how advanced the cancer might be. He was treated with a hormonal therapy that might not have been necessary and had a significant negative impact on his quality of life.
Because of research supported by NIH, along with highly effective collaborations with the private sector, prostate cancer can now be treated with individualized precision and improved outcomes.
As in my case, high-resolution MRI scans can now be used to delineate the precise location of a tumor. When combined with real-time ultrasound, this allows pinpoint targeting of the prostate biopsies. My surgeon will be assisted by a sophisticated robot named for Leonardo da Vinci that employs a less invasive surgical approach than previous techniques, requiring just a few small incisions.
Advances in clinical treatments have been informed by large-scale, rigorously designed trials that have assessed the risks and benefits and were possible because of the willingness of cancer patients to enroll in such trials.
I feel compelled to tell this story openly. I hope it helps someone. I don’t want to waste time.
If my cancer recurs, the DNA analysis that has been carried out on my tumor will guide the precise choice of therapies. As a researcher who had the privilege of leading the Human Genome Project , it is truly gratifying to see how these advances in genomics have transformed the diagnosis and treatment of cancer.
I want all men to have the same opportunity that I did. Prostate cancer is still the No. 2 cancer killer among men. I want the goals of the Cancer Moonshot to be met — to end cancer as we know it. Early detection really matters, and when combined with active surveillance can identify the risky cancers like mine, and leave the rest alone. The five-year relative survival rate for prostate cancer is 97 percent, according to the American Cancer Society , but it’s only 34 percent if the cancer has spread to distant areas of the body.
But lack of information and confusion about the best approach to prostate cancer screening have impeded progress. Currently, the U.S. Preventive Services Task Force recommends that all men age 55 to 69 discuss PSA screening with their primary-care physician, but it recommends against starting PSA screening after age 70.
Other groups, like the American Urological Association , suggest that screening should start earlier, especially for men with a family history — like me — and for African American men, who have a higher risk of prostate cancer. But these recommendations are not consistently being followed.
Our health-care system is afflicted with health inequities. For example, the image-guided biopsies are not available everywhere and to everyone. Finally, many men are fearful of the surgical approach to prostate cancer because of the risk of incontinence and impotence, but advances in surgical techniques have made those outcomes considerably less troublesome than in the past. Similarly, the alternative therapeutic approaches of radiation and hormonal therapy have seen significant advances.
A little over a year ago, while I was praying for a dying friend, I had the experience of receiving a clear and unmistakable message. This has almost never happened to me. It was just this: “Don’t waste your time, you may not have much left.” Gulp.
Having now received a diagnosis of aggressive prostate cancer and feeling grateful for all the ways I have benefited from research advances, I feel compelled to tell this story openly. I hope it helps someone. I don’t want to waste time.
Francis S. Collins served as director of the National Institutes of Health from 2009 to 2021 and as director of the National Human Genome Research Institute at NIH from 1993 to 2008. He is a physician-geneticist and leads a White House initiative to eliminate hepatitis C in the United States, while also continuing to pursue his research interests as a distinguished NIH investigator.
An earlier version of this article said prostate cancer is the No. 2 killer of men. It is the No. 2 cause of cancer death among men. The article has been updated.
- How to revive hair that thins, grays or gets out of control as you age April 15, 2024 How to revive hair that thins, grays or gets out of control as you age April 15, 2024
- Anxious about your first mammogram? Here’s what I learned. April 13, 2024 Anxious about your first mammogram? Here’s what I learned. April 13, 2024
- Medical Mysteries: Years of hives and fevers traced to a startling cause April 13, 2024 Medical Mysteries: Years of hives and fevers traced to a startling cause April 13, 2024

Jump to navigation
2024 Dress and Body Association Conference, 2-3 Nov (Abstracts due: July 1)
2024 Dress and Body Association Conference CALL FOR PAPERS
The Dress and Body Association invites submissions for the organization’s fifth annual conference, which will be held on November 2-3, 2024 . Consistent with our long-term goals for inclusivity and sustainability, all activities will be 100% online, including keynote speaker(s), research presentations, and opportunities for virtual networking. Visit the DBA website— www.dress-body-association.org —to learn more about this organization and consider becoming a member.
Food Studies Research Panel at PAMLA
Conference Dates - November 7th to 10th 2024
Location - Palm Springs, California U.S.A.
Topic - Food Studies Research on Culture, Literature, and Media
Conference organizers - Pacific Ancient & Modern Language Association
Overview - This Food Studies session aims to bring together a range of perspectives for the sake of examining the roles of food in literary and media texts. We seek abstracts that speak to the following questions: What roles do food and food studies play in our analyses of culture, literature, and media? How does food experience shape our creative expression and daily lives?
Summer Institute: Waste Worlding
Waste Worlding
June 3-6, 2024
Virtual Summer Institute
Bucknell Humanities Center
Application Deadline: May 10, 2024
Decisions by May 15, 2024
PAMLA 2024 Panel CFP: Voices of Palestinian Liberation
VOICES OF PALESTINIAN LIBERATION
PAMLA Conference
7-10 November 2024 in Palm Springs, California
Session Format: Panel of 3-4 presenters, 15-20 minutes each and a 30-minute group Q&A afterward
This panel centers the literary and artistic expressions of Palestinians by exploring how writers, artists, journalists, historians, and activists navigate themes of abject loss, violence, and trauma; the aim is to offer a multifaceted perspective on the Palestinian experience, one that transcends problematically uncomplicated narratives and foregrounds the power of language and media in shaping identity, fostering solidarity, and demanding justice.
The Translator at Work: Neutrality in Translation and Interpreting
« The Translator at Work: Neutrality in Translation and Interpreting »
Translators and interpreters are expected to be neutral mediators who facilitate dialogue and enable understanding and cooperation between speakers who do not speak the same language. Research has shown, however, that the transfer of information from one language to another is rarely performed without making certain contributions that go beyond the mere rendition of the message being transferred. Translation is a product of cross-cultural interactions that requires linguistic and at times, sociopolitical or even ideological changes.
Global Film Symposium: Engaging Global Cinema Cultures - Discourses and Disruptions
Engaging Global Cinema Cultures: Discourses and Disruptions
In-person at the University of Texas at Dallas
Keynote Speakers:
Dr. Lúcia Nagib (University of Reading)
Dr. Lalitha Gopalan (University of Texas at Austin)
Special guests include Dr. Iggy Cortez (University of California, Berkeley), Dr. Shekhar Deshpande (Arcadia University), and Dr. Meta Mazaj (University of Pennsylvania).
Call for Papers & Guest Editor Proposals: Interactions: Studies in Communication & Culture
This is a general call for papers, as well as for proposals for guest edited issues, for Interactions: Studies in Communication and Culture (ISCC), published by Intellect Books (ISSN 17572681, Online ISSN 1757269X).
Papers will be considered for publication in our Autumn 2024 issue as well as for 2025 and beyond, while proposals for guest edited issues will be considered for publication from early 2025.
View the full call here>>
[CfP] Contested & Erased Energy Knowledges
Call for Papers
Contested & Erased Energy Knowledges
A Trans-Disciplinary Conference
31 Oct – 2 Nov 2024
University of Dundee & University of Edinburgh
Edinburgh, Scotland
Masculinity Studies in Ireland: Mapping the Field
As an interdisciplinary academic field devoted to examining and critiquing the social, cultural, and historical constructions of masculinity, masculinity studies explores how masculinity is defined, performed, and experienced across different societies and time periods. This field intersects with various disciplines, including sociology, gender studies, psychology, cultural studies, and history, among others. This often leads to the absence of dedicated departments or research centres in academic institutions focusing exclusively on masculinity studies which, in turn, makes it challenging to enable productive dialogues among disciplinary boundaries.
Call for paper abstracts for the Thematic issue “Notions of Responsibility in Contemporary Discourse: Ethics, Law, Politics” (“Athena: Philosophical Studies” No. 19, 2024)
“Athena: Philosophical Studies” No. 19, 2024
Indexed in: Scopus (2023), CEEOL (Central and Eastern European Online Library) (2006), EBSCO Publishing, Humanities International Index (2006), The Philosopher’s Index (2006).
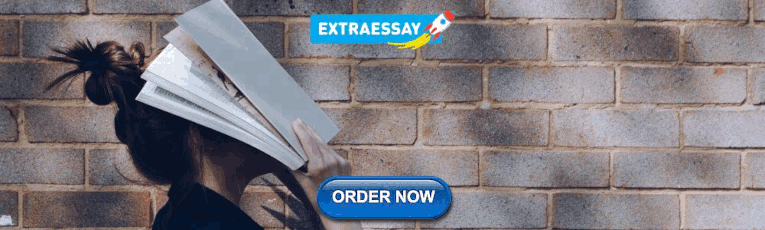
IMAGES
VIDEO
COMMENTS
Water scarcity is one of the major problems in the world and millions of people have no access to freshwater. Untreated wastewater is widely used for agriculture in many countries. This is one of the world-leading serious environmental and public health concerns. Instead of using untreated wastewater, treated wastewater has been found more applicable and ecofriendly option. Moreover ...
A puri fication process generally consists of five. successive steps as described in Fig. 1.1: (1) preliminary treatment or. pre-treatment (physical and mechanical); (2) primary treatment (physi ...
The Treatment of Wastewater, Recycling and Reuse. - Past, Present, and in the Future. Jyotsana Maura, Suchita Atreya, Anfal Arshi. Defence Institute of Bio-Energy Research (DIBER), DRDO, Haldwani ...
This paper discusses the potential of recycled wastewater (also known as reused water) to become a significant source of safe water for drinking purposes and improved sanitation in support of the ...
Mexico City 11350, Mexico; [email protected]; Tel.: +52-55-5729-6000. Abstract: W astewater treatment involves the extraction of pollutants, removal of coarse particles, and elimination of toxicants ...
Microbial electrolysis cell technology (MEC) MEC's is a cutting-edge technology that can replace tradi-tional WWT methods, like conventional aerobic and non-aerobic treatment methods. The main attributes of MEC's are that they enable the conversion of organic content pre-sent in sewage water into hydrogen gas.
Feature papers represent the most advanced research with significant potential for high impact in the field. A Feature Paper should be a substantial original Article that involves several techniques or approaches, provides an outlook for future research directions and describes possible research applications. ... Wastewater and landfill ...
Besides, the review also covers research focused on wastewater treatment plants, disposal, and the management of wastewater sludge as well as biosolids in the environment. Practitioner points This paper highlights the review of scientific literature published in the year 2019.
Due to unrestricted entry of wastewater into the environment and the transportation of microbial contaminants to humans and organisms, environmental protection requires the use of appropriate purification systems with high removal efficiency for microbial agents are needed. The purpose of this study was to determine the efficacy of current wastewater treatment systems in removing microbes and ...
Therefore, this paper presents a clear view to the researchers as well as the industries about the existing scenario, treatment options, challenges, and opportunities associated with wastewater treatment methods. Also, the paper highlights the promising tools to find the solution of wastewater and converting it into the availability of treated ...
The table presents a summary of review-papers published from 2013 to 2022 on different topics related to water and wastewater management. The review-papers have been analyzed based on critical aspects like; their authors, year of publication, research area, the number of papers reviewed, the method/procedure used, limitations observed and cited ...
His research interests are in the area of water quality engineering, with emphasis on biotechnology for water and wastewater treatment, sustainable, low-cost water/wastewater treatment processes ...
More research on introducing cost-effective materials and methods for heavy metal removal from wastewater should be carried out. Future studies should also focus on the pilot-scale process.
The removal and recovery of phosphorus from wastewater is a crucial topic for environmental protection and resource utilization. This work provides a review of research advances in phosphorus removal and recovery from wastewater, based on a visual analysis of relevant literatures using bibliometric tools such as Citespace, VOSviewer, and Bibliometrix R-package.
This paper shows that wastewater research offers interesting possibilities, but that legal regulation is still lacking, while there are ethical risks involved. This is a well-known phenomenon in the development of new technologies and it inevitably requires a balancing act to establish guidelines that are not either too permissive or too ...
Wastewater Research. Wastewater management is a critical part of the anthropogenic water cycle that helps ensure that water is clean, safe to use, and protective of ecosystems. Wet weather runoff that flows from urban communities into wastewater collection systems is a significant challenge. Runoff from unpredictable strong storms or continuous ...
The consumption of crop irrigated by wastewater has leading health implications also discussed in this review paper. This review further reveals that our current understanding of the wastewater ...
The growing concerns over water contamination have led to extensive research and development in water treatment techniques. They are expanding to promote the reuse of water and improve the quality of water for human consumption. ... The results of the research conducted by showed that treatment of paper pulp wastewater by microbial consortium ...
Hybrid zeolite-based ion-exchange and sulfur oxidizing denitrification for advanced slaughterhouse wastewater treatment. Journal of Environmental Sciences . 10.1016/j.jes.2021.06.002 . 2022 . Vol 113 . pp. 219-230. Author (s): Shuang Tong . Shaoxiang Zhang .
Switch to green wastewater infrastructure could reduce emissions and provide huge savings according to new research. ScienceDaily . Retrieved April 15, 2024 from www.sciencedaily.com / releases ...
Research trends in the use of waste materials as landfill liners from 2002 to 2021 Data processing. The first search, performed on Scopus, used filters from keywords, titles, and abstracts with the "TITLE-ABS-KEY" function. TITLE-ABS-KEY (Crack AND Using AND Waste AND Material). There, 2025 papers were found.
1. Introduction. Wastewater generation has increased at an alarming rate in recent years, becoming a matter of great environmental and public health concern [1], because when untreated, wastewater discharged into water bodies or into the ground, besides be considered an crime, may contributes to development of waterborne diseases [2].Some of the factors responsible for this vast amount ...
Filtration is a water treatment system that involves the separation of solids from liquids using a porous medium to remove suspended solids, colloids, and other substances (Charles 2009;Zeki 2009
Data, research, outlooks and country reviews on environment including biodiversity, water, resource and waste management, climate change, global warming and consumption., Extended Producer Responsibility (EPR) is a policy approach that makes producers responsible for their products along the entire lifecycle, including at the post-consumer stage.
This paper reviews the existing research where digital twins have been directly used to enhance sustainability throughout the entire life cycle of a building (including design, construction, operation and maintenance, renovation, and demolition). Additionally, we introduce a conceptual framework for this industry, which involves the elements of ...
Inorganic chlorine is susceptible to water and soil salinization due to its non-degradability and high mobility. To clarify the environmental risks associated with the active inorganic chlorine in municipal solid waste (MSW), the specific characteristics and contributions of inorganic chlorine in different MSW categories were investigated in this study. MSW samples were collected from eight ...
I don't want to waste time. Accessibility ... served as director of the National Institutes of Health from 2009 to 2021 and as director of the National Human Genome Research Institute at NIH ...
2024 Dress and Body Association Conference CALL FOR PAPERS The Dress and Body Association invites submissions for the organization's fifth annual conference, which will be held on November 2-3, 2024.Consistent with our long-term goals for inclusivity and sustainability, all activities will be 100% online, including keynote speaker(s), research presentations, and opportunities for virtual ...
waste materials [140,141,142143,144,145]. ese materials are abundant, envir onmentally friendly , reusable and low cost, T able 4. T able 4: Some natural polymers and agricultural wastes for heavy ...
2016. ABSTRACT. This paper reviewed five (5) researches on industrial wastewater treatment processes, the. methods emplo yed in these researches are aerobic, anaerobic or the combination of both ...